Abstract
Keywords
Introduction
The dimorphic fungus, Candida albicans, is a major fungal pathogen responsible for causing a variety of candidiasis. It is the fourth leading cause of nosocomial infections, with a mortality rate approaching 50% (1,2). The organism is known to cause local infections, such as vaginitis and thrush, and can cause serious life-threatening invasive and systemic disease, especially among immunocompromised and immunodeficient patients who are receiving broad-spectrum antibiotics, patients undergoing cancer chemotherapy, organ transplant recipients, or individuals infected with the human immunodeficiency virus (HIV) (3,4).
Currently, the azoles, which have a broad-spectrum antifungal activity against a wide variety of candida species, are widely used for both the prevention and treatment of candidiasis (5). However, more recently, the treatment of candidal infections has led to several problems. In addition to the toxicity presented by some fungicidal agents, such as amphotericin B (AMB)(6), other conventional fungistatics have been rendered ineffective by resistance or dose-dependent susceptibility found in some C. albicans isolates. In particular, azole-resistant isolates are appearing at a high frequency due to the increasing clinical use of the azoles. Thus, new therapeutic strategies to cope with candidal infections are necessary. Combination therapy is a novel approach that can be used to decrease the toxicity of an antifungal drug and improve the efficacy of the antifungal therapy and may be especially useful for treating infections caused by drug-resistant fungi (7).
Chelerythrine (CHT, C21H17NO4, as illustrated in Figure 1), one of the more important benzophenanthridine alkaloids derived from the roots of Chelidonium majus, has been shown to have various biological activities, including antimicrobial, antiplatelet, and antitumor activities (8,9,10). Additionally, a previous report showed that CHT has been recommended for medical use in the treatment of oral inflammatory processes due to its low toxicity and strong anti-inflammatory effects (11, 12).
In this study, we investigated the antifungal activity of CHT and econazole-nitrate (ECZN) against C. albicans, and assessed the combination of CHT and ECZN for the treatment of candidasis. To evaluate this combination for synergism, the checkerboard microtiter test and time-kill assays were performed.
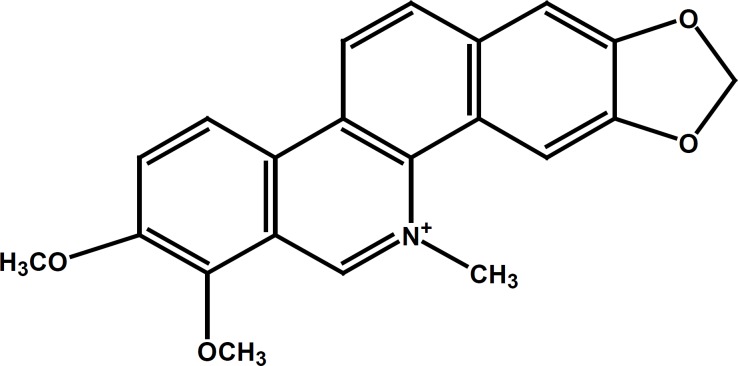
Experimental
Strains and growth conditions
C. albicans ATCC 10231 was obtained from the American Type Culture Collection (ATCC, Gaithersburg, MD, USA). In addition, the ten fluconazole-resistant isolates of C. albicans used in this study were kindly provided by Jiang Y.Y. The strains were maintained on Sabouraud dextrose agar (SDA, 4% glucose, 1% Bacto peptone and containing 3% agar) plates and stored at 4 ℃ during the experimental period. C. albicans ATCC 10231 was used as the quality control strain.
Antifungal agents
CHT and ECZN were used in this study. The CHT (≥98% pure) and the ECZN (≥98% pure) were purchased from the National Institute for the Control of Pharmaceutical and Biological Products, Beijing, China. DMSO (dimethyl sulfoxide) was used to prepare stock solutions of CHT (20480 µg/mL) and ECZN (40960 µg/mL). All of the antifungal stock solutions were maintained at -20 ℃. The final concentration of DMSO in the wells was less then 1% v/v, which did not affect the growth of the test organisms in all of the susceptibility tests (13).
Antifungal susceptibility testing
The minimum inhibitory concentrations (MICs) of CHT and ECZN against the Candida strains were determined using the broth microdilution method as described by the Clinical and Laboratory Standards Institute (CLSI, formerly the National Committee for Clinical Laboratory Standards) document M27-A. The susceptibility test was performed in a 96-well flat-bottomed microtitration plate according to the process of L. Drago et al. (14). Briefly, all tested isolates were incubated at 35 ℃ in Sabouraud dextrose broth (SDB and diluted with the same fresh medium to a density of ~106 cfu/mL, which was further diluted to generate a final concentration of 5×105 cfu/mL dilutions. The MIC values of CHT and ECZN were tested after serial 2-fold dilutions in 96-well flat-bottomed microtitration plates in SDB, and the final concentrations of the antimicrobial broth ranged from 0.25 to 512 µg/mL. The MIC was defined as the lowest concentration with no visible growth compared to that of the drug-free control. The quality control (QC) strain, C. albicans ATCC 10231, was included in each batch of the susceptibility tests to ensure quality.
Checkerboard method
Interactions between ECZN and CHT were tested in 96-well flat-bottomed microtitration plates by the checkerboard method against the ten C. albicans isolates and the susceptible C. albicans ATCC 10231 strain in the same medium as used previously. The final antimicrobial agent concentrations after the addition of 100 µL of inoculum ranged from 0.25 µg/mL to 512 µg/mL for ECZN and from 8 µg/mL to 512 µg/mL for CHT. The inocula were prepared at a final concentration of 5×105 cfu/mL per well. The plates were incubated at 35 ℃ for 24 - 48 h. The effects of the combinations of antimicrobial agents were interpreted by the fractional inhibitory concentration index (FICI). Based on LA theory, the FICI was calculated by the following equation (15):
FICI = FICA + FICB = MICAcomb ⁄ MICAalone + MICBcomb ⁄ MICBalone
The effect of the combinations of antimicrobial agents was classified by the following standard: (1) FICI ≤ 0.5, synergistic effect; (2) 0.5 ≤ FICI ≤ 4.0, additive or indifferent; and (3) FICI > 4.0, antagonistic. Student’s t-test analysis was performed to analyse the means of MICs between used ECZN alone and the ECZN-CHT combination, based on spss version 17.0 for windows. p-values<0.05 were accepted as statistically significant.
Time-kill studies
Time–kill studies were performed with the chosen isolates using the methodology of L. Drago et al. (14). DMSO comprised <1% of the total test volume. CHT and ECZN were diluted in SDB to obtain a final concentration of 1/2 MIC. C. albicans 687 and 762 were prepared at the starting inoculum of 106 cfu/mL of 0.5 mL volume to obtain a final concentration of 105 cfu/mL in the 5 mL final volume system. The concentrations of the agents were determined by the MIC values obtained in the previous experiment. The tubes containing CHT (32 µg/mL), ECZN (16 µg/mL), CHT/ECZN (32 µg/mL and 16 µg/mL, respectively) and 105 cfu/mL of the tested isolates were incubated at 35 °C. At various predetermined time points (0, 12, 24, and 48 h), 100 µL aliquots were removed from each test tube and serially diluted 10 fold in sterile water. A volume of 100 µL of each dilution was spread on the Sabouraud dextrose agar plates to incubate at 35 °C for 24 h prior to colony counts enumeration. Each assay was performed in triplicate. Synergism and antagonism were defined by the following criteria (16): (1) synergy: a 2 log10cfu/mL decrease by the combination compared to the most active agent; (2) antagonism: a 2 log10cfu/mL increase by the combination compared to the most active agent; and (3) indifferent: a change of < 2 log10cfu/mL between the combination and the most active agent.
Results
Antifungal activities and interactions of drugs
The in-vitro antifungal activities of CHT and ECZN alone and in combination were assessed. The results for the tested drugs alone and the checkerboard analysis are summarized in Table 1.
In testing the two antifungals independently, the MIC values for these two agents against the clinical isolates of C. albicans ranged from 16 to 32 µg/mL for ECZN and 32 to 128 µg/mL for CHT treatment. These results showed that CHT has antifungal activity against clinical isolates of C. albicansin-vitro.
In the combination studies, the interaction between ECZN and CHT displayed synergism for all tested strains, including C. albicans ATCC 10231, with FICI values ranging from 0.078125 to 0.5 using the FICI method. Moreover, an antagonism interaction was not observed for any of the tested strains. As shown in Table 1, the ECZN-CHT combination markedly reduced the MICs. The data above showed that there was a good synergistic antifungal effect against C. albicans when CHT was combined with ECZN.
In-vitro interaction between chelerythrine and econazole-nitrate against clinical isolates of Candida albicans
Strains | Median MIC (range) of durg along (µg/mL) | Median MIC (range) in combination (µg/mL) | Results | |||
---|---|---|---|---|---|---|
CHT | ECZN | CHT | ECZN | FICI | INT | |
C. albicans 580 | 32 | 16 | 8 | 1 | 0.3125 | SYN |
C. albicans 659 | 64 | 16 | 4 | 1 | 0.125 | SYN |
C. albicans 687 | 64 | 32 | 16 | 8 | 0.5 | SYN |
C. albicans 762 | 64 | 32 | 16 | 4 | 0.375 | SYN |
C. albicans 817 | 128 | 32 | 16 | 1 | 0.15625 | SYN |
C. albicans 876 | 128 | 32 | 8 | 0.5 | 0.078125 | SYN |
C. albicans 885 | 64 | 16 | 16 | 2 | 0.375 | SYN |
C. albicans 893 | 32 | 32 | 8 | 0.5 | 0.265625 | SYN |
C. albicans 904 | 64 | 32 | 16 | 4 | 0.375 | SYN |
C. albicans 0604109 | 64 | 32 | 16 | 4 | 0.375 | SYN |
C. albicans 10231 | 128 | 32 | 16 | 2 | 0.1875 | SYN |
Time-kill curves
To analyze the interaction of these drug combinations, we used the time-kill approach. The results of the time-kill curves with the antimicrobials alone or in combination against two chosen clinical isolates, C. albicans 687 and 762, are presented in Figure 2. As shown in the graph, the lines that represent the agents alone have a similar tend. This suggests that the tested strains had similar susceptibilities to CHT (32 µg/mL) and ECZN (16 µg/mL). Given an initial inoculum density of 105cfu/mL, combination therapy yielded a 2.06 log10cfu/mL decrease for C. albicans 687 and a 2.26 log10cfu/mL decrease for C. albicans 762, compared to 16 µg/mL of ECZN after 48 h of incubation. The fungistatic activity of ECZN was dramatically enhanced by the addition of CHT. For the two strains tested, time–kill curves verified synergism for the ECZN⁄CHT combination.
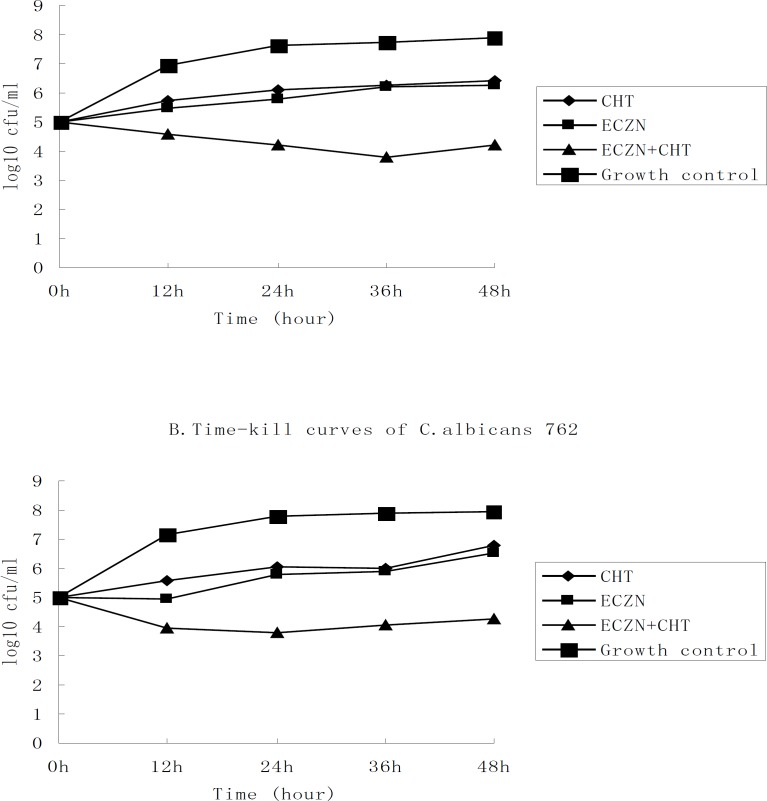
Discussion
A synergistic strategy can be an important approach for the treatment of disease, as it often shows a better effectiveness compared to monotherapy and can lower drug dosage requirements, reduce the toxic side-effects of drugs and prevent or delay the emergence of drug resistance. Recently, there have been many reports that have shown synergistic effects between antibacterial agents or peptides in combination with fluconazole against C. albicans (17,18,19 and 20), but few studies have been conducted investigating synergism with ECZN. ECZN, which belongs to the imidazoles, is another important antifungal agent largely used for the treatment of many nosomycosis, especially surface infections, such as mucous membranes infections, dermatophytosis and vaginitis (21). The present study was undertaken to analyze the drug–drug interactions between ECZN and CHT using the checkerboard microdilution method. Based on the experimental data, it can be concluded that a synergistic combination effect was observed in all of the test strains, and no antagonistic action was observed. Furthermore, the positive interactions between the two antimicrobials were also confirmed by the time–kill curves in the selected strains. Moreover, the results of both indicated that there was good agreement between the conclusions drawn from the FICI method and the time–kill curves for the strains tested.
In C. albicans, there are four targets (cell wall biosynthesis, membrane integrity, sterol biosynthesis and DNA/RNA synthesis) of fungicidal agents. The specific mechanism of the antifungal effect of ECZN has been reported. In brief, ECZN inhibits the activity of the cytochrome P-450 lanosterol 14a-demethylase, which plays important roles in the ergosterol biosynthetic pathway, and this pathway is considered to be the primary target of the azole antifungal drugs (22,23). Hence, two effects are produced. First, it disrupts the biosynthesis of ergosterol (the main sterol in the fungal cell membrane), which influences cell membrane integrity. Second, it can accumulate toxic methylated sterol intermediates that can damage the fungal cell.
Although the underlying mechanism of the synergism between ECZN and CHT remains unclear, some understanding may be derived from previous studies. CHT has been reported to have significant antibacterial activity against Gram-positive bacteria and C. albicans (24) and has recently been extensively studied as a protein kinase C (PKC) inhibitor (25,26,27). Its antimicrobial activity may be involved with the inhibitory action against PKC. PKC has been associated with the regulation of cell proliferation, differentiation, and survival, and it has been widely studied in fungi. More recently, many researchers have shown that pkc1, a more primitive PKC isoenzyme in fungus, can regulate chitin (a component of the cell wall) synthesis and drug susceptibility in C. albicans. The inhibition of pkc1 could enhance the efficacy of antifungal agent targeting the cell membrane, including the azoles (2,28). Thus, it can be inferred that the mechanism of synergy between ECZN and CHT may be due to the PKC inhibitory effect of CHT. In addition, recent studies have shown that both CHT and ECZN produce reactive oxygen species (ROS), which leads to apoptosis (29,30,31). Moreover, CHT has been shown to play a role in DNA damage (32). Although there is no evidence to indicate that all of these drug characters could act on C. albicans, hypothetically, these functions may be involved in the synergic mechanism. The exact cooperative mechanism may be multifactorial and will have to be further explored.
CHT is a potent PKC inhibitor and antifungal compound that may have more significant therapeutic potential against candidiasis, especially in immunocompromised patients, due to its low toxicity and antitumor activity. In conclusion, the results of this study suggest that CHT can markedly enhance the effects of ECZN against isolates of C. albicans. Moreover, this synergism can markedly reduce the dosage requirements of ECZN, decreasing the ECZN toxic side effects. This ECZN-CHT synergism may have significant clinical implications for the treatment of superficial mycosis.
Acknowledgements
References
-
1.
Ambrogi V, Perioli L, Pagano C, Marmottini F, Moretti M, Mizzi F, Rossi C. Econazole nit rate-loaded mcm-41 for an antifungal topical powder formulation. J. Pharm. Sci. 2010;99:4738-4745. [PubMed ID: 20845470].
-
2.
Berger SA, Yu Y, Niapour M, Zhang Y. Mitochondrial regulation by c-Myc and hypoxia-inducible factor-1 alpha controls sensitivity to econazole. Mol. Cancer Ther. 2008;7:483-491. [PubMed ID: 18347136].
-
3.
Che X, Sheng C, Wang W, Cao Y, Xu Y, Ji H, Dong G, Miao Z, Yao J, Zhang W. New azoles with potent antifungal activity: design, synthesis and molecular docking. Eur. J. Med. Chem. 2009;44:4218-4226. [PubMed ID: 19539408].
-
4.
Cowen LE, Steinbach WJ. Stress, drugs, and evolution: the role of cellular signaling in fungal drug resistance. Eukaryot. Cell. 2008;7:747-764. [PubMed ID: 18375617].
-
5.
Dias BP, Endo EH, Cortez DAG, Ueda-Nakamura T, Nakamura CV. Potent antifungal activity of extracts and pure compound isolated from pomegranate peels and synergism with fluconazole against Candida albicans. Res. Microbiol. 2010;161:534. [PubMed ID: 20541606].
-
6.
Drago L, Nicola L, Rodighiero V, Larosa M, Mattina R, De Vecchi E. Comparative evaluation of synergy of combinations of beta-lactams with fluoroquinolones or a macrolide in streptococcus pneumoniae. J. Antimicrob. Chemoth. 2011;66:845-849.
-
7.
Funakoshi T, Aki T, Nakayama H, Watanuki Y, Imori S, Uemura K. Reactive oxygen species-independent rapid initiation of mitochondrial apoptotic pathway by chelerythrine. Toxicol. In-vitro. 2011;25:1581-1587. [PubMed ID: 21664962].
-
8.
Han Y, Lee JH, Jang EC. Combination immunotherapy of MAb B6.1 with fluconazole augments therapeutic effect to disseminated candidiasis. Pharmacal. Res. 2011;34:399-405.
-
9.
Harmati G, Papp F, Szentandrassy N, Barandi L, Ruzsnavszky F, Horvath B, Banyasz T, Magyar J, Panyi G, Krasznai Z, Nanasi PP. Effects of the PKC inhibitors chelerythrine and bisindolylmaleimide I (GF 109203X) on delayed rectifier K+ currents. Naunyn. Schmiedebergs. Arch. Pharmacol. 2011;383:141-148. [PubMed ID: 21120453].
-
10.
Hitchcock CA, Dickinson K, Brown SB, Evans EG, Adams DJ. Interaction of azole antifungal antibiotics with cytochrome P-450-dependent 14 alpha-sterol demethylase purified from Candida albicans. Biochem. J. 1990;266:475-480. [PubMed ID: 2180400].
-
11.
Huang S, Cao YY, Dai BD, Sun XR, Zhu ZY, Cao YB, Wang Y, Gao PH, Jiang YY. In-vitro synergism of fluconazole and baicalein against clinical isolates of Candida albicans resistant to fluconazole. Biol. Pharm. Bull. 2008;31:2234-2236. [PubMed ID: 19043205].
-
12.
Jin Q, Yu L, Ling GH, Deng XM, Jin J, Guo N. In-Vitro interaction between fluconazole and triclosan against clinical isolates of fluconazole-Resistant Candida albicans determined by different Methods. Antimicrob. Agents Chemother. 2011;55:3609-3612. [PubMed ID: 21576450].
-
13.
Kaminskyy V, Lin KW, Filyak Y, Stoika R. Differential effect of sanguinarine, chelerythrine and chelidonine on DNA damage and cell viability in primary mouse spleen cells and mouse leukemic cells. Cell Biol. Int. 2008;32:271-277. [PubMed ID: 18029203].
-
14.
Kosina P, Walterova D, Ulrichova J, Lichnovsky V, Stiborova M, Rydlova H, Vicar J, Krecman V, Brabec MJ, Simanek V. Sanguinarine and chelerythrine: assessment of safety on pigs in ninety days feeding experiment. Food Chem. Toxicol. 2004;42:85-91. [PubMed ID: 14630132].
-
15.
LaFayette SL, Collins C, Zaas AK, Schell WA, Betancourt-Quiroz M, Gunatilaka AA, Perfect JR, Cowen LE. PKC signaling regulates drug resistance of the fungal pathogen Candida albicans via circuitry comprised of Mkc1, calcineurin, and Hsp90. PLos. Pathog. 2010;6:1001069.
-
16.
Lenfeld J, Kroutil M, Marsalek E, Slavik J, Preininger V, Simanek V. Antiinflammatory activity of quaternary benzophenanthridine alkaloids from Chelidonium majus. Planta. Med. 1981;43:161-165. [PubMed ID: 7312984].
-
17.
Matkar SS, Wrischnik LA, Hellmann-Blumberg U. Production of hydrogen peroxide and redox cycling can explain how sanguinarine and chelerythrine induce rapid apoptosis. Arch. Biochem. Biophys. 2008;477:43-52. [PubMed ID: 18555791].
-
18.
Miao F, Yang XJ, Zhou L, Hu HJ, Zheng F, Ding XD, Sun DM, Zhou CD, Sun W. Structural modification of sanguinarine and chelerythrine and their antibacterial activity. Nat. Prod. Res. 2011;25:863-875. [PubMed ID: 21491327].
-
19.
Mukherjee PK, Sheehan DJ, Hitchcock CA, Ghannoum MA. Combination treatment of invasive fungal infections. Clin. Microbiol. Rev. 2005;18:163-194. [PubMed ID: 15653825].
-
20.
Munro CA, Selvaggini S, de Bruijn I, Walker L, Lenardon MD, Gerssen B, Milne S, Brown AJ, Gow NA. The PKC, HOG and Ca2+ signalling pathways co-ordinately regulate chitin synthesis in Candida albicans. Mol. Microbiol. 2007;63:1399-1413. [PubMed ID: 17302816].
-
21.
Odds FC. Synergy, antagonism, and what the chequerboard puts between them. J. Antimicrob. Chemother. 2003;52:1. [PubMed ID: 12805255].
-
22.
Pfaller MA, Diekema DJ. Epidemiology of invasive candidiasis: a persistent public health problem. Clin. Microbiol. Rev. 2007;20:133. [PubMed ID: 17223626].
-
23.
Quan H, Cao YY, Xu Z, Zhao JX, Gao PH, Qin XF, Jiang YY. Potent in-vitro synergism of fluconazole and berberine chloride against clinical isolates of Candida albicans resistant to fluconazole. Antimicrob. Agents. Ch. 2006;50:1096-1099.
-
24.
Sacchetti B, Bielavska E. Chelerythrine, a specific PKC inhibitor, blocks acquisition but not consolidation and retrieval of conditioned taste aversion in rat. Brain Res. 1998;799:84-90. [PubMed ID: 9666086].
-
25.
Sanglard D, Marchetti O, Moreillon P, Entenza JM, Vouillamoz J, Glauser MP, Bille J. Fungicidal synergism of fluconazole and cyclosporine in Candida albicans is not dependent on multidrug efflux transporters encoded by the CDR1, CDR2, CaMDR1, and FLU1 genes. Antimicrob. Agents Chemother. 2003;47:1565-1570. [PubMed ID: 12709323].
-
26.
Sroussi HY, Kohler GA, Agabian N, Villines D, Palefsky JM. Substitution of methionine 63 or 83 in S100A9 and cysteine 42 in S100A8 abrogate the antifungal activities of S100A8/A9: potential role for oxidative regulation. FEMS Immunol. Med. Microbiol. 2009;55:55-61. [PubMed ID: 19087201].
-
27.
Sun S, Li Y, Guo Q, Shi C, Yu J, Ma L. In-vitro interactions between tacrolimus and azoles against Candida albicans determined by different methods. Antimicrob. Agents Chemother. 2008;52:409-417. [PubMed ID: 18056277].
-
28.
Von Stebut E, Amon U, Herbert JM, Wolff HH. Investigations with the selective PKC inhibitor chelerythrine on human basophils. Agents Actions. 1994;41:56-57.
-
29.
Weichselbaum RR, Chmura SJ, Dolan ME, Cha A, Mauceri HJ, Kufe DW. In-vitro and in-vivo activity of protein kinase C inhibitor chelerythrine chloride induces tumor cell toxicity and growth delay in-vivo. Clin. Cancer Res. 2000;6:737-742. [PubMed ID: 10690561].
-
30.
Yang R, Piperdi S, Gorlick R. Activation of the RAF/mitogen-activated protein/extracellular signal-regulated kinase kinase/extracellular signal-regulated kinase pathway mediates apoptosis induced by chelerythrine in osteosarcorna. Clin. Cancer Res. 2008;14:6396-6404. [PubMed ID: 18927278].
-
31.
Yu R, Mandlekar S, Tan TH, Kong ANT. Activation of p38 and c-Jun N-terminal kinase pathways and induction of apoptosis by chelerythrine do not require inhibition of protein kinase C. J. Biol. Chem. 2000;275:9612-9619. [PubMed ID: 10734112].
-
32.
Zaoutis TE, Argon J, Chu J, Berlin JA, Walsh TJ, Feudtner C. The epidemiology and attributable outcomes of candidemia in adults and children hospitalized in the United States: a propensity analysis. Clin. Infect. Dis. 2005;41:1232-1239. [PubMed ID: 16206095].