Abstract
Keywords
Rutin Buckwheat Angiotensin II Cardiac hypertrophy Calcineurin
Introduction
Myocardial hypertrophy, which often leads to congestive heart failure, severe arrhythmia and disruption of important artery branches, is a commonly seen complication of cardiovascular system diseases. It is an independent risk factor causing the increase of death rate of cardiovascular diseases, and it gravely threatens the physical and mental health and life quality of human being.
Myocardial hypertrophy is commonly caused by hypertension, vascular disease of the heart, coronary heart disease, myocardial infarction and congenital heart disease etc. In recent years, the experts have thought highly of the imbalance of neurohumor factors such as catecholamine, endothelin-1, various growth factors, especially the renin-angiotensin-aldosterone system that causes myocardial hypertrophy. Generous investigations have manifested that AngII has growth factor like effect. It can cause the increase in total protein content of cardiac myocytes and in protein synthesis rate, which results in the hypertrophy of cardiac myocytes. It can also increase the synthesis and excretion of matrixes of myocardium interstitial cells (mainly cardiac fibroblasts) and causes myocardial hypertrophy or myocardial fibrosis. The elevation of intracellular Ca2+ level is indicated to be the central link of hemodynamics overload and various neurohumor factors inducing myocardial hypertrophy. Ca2+ - calmodulin (CaM) dependent CaN pathway is a recently discovered important signal transduction pathway, which directly participates in several extracellular signal pathways that cause myocardial hypertrophy.
As to the treatment of myocardial hypertrophy, immunodepressant Cyclosporin A (CsA) is studied the most. CsA could effectively suppress the expression of myocardial CaN-NFAT3-GATA4 thus inhibit myocardial hypertrophy and improve congestive heart failure. Due to the impact of side effects, CsA has not been spreadly applied in the clinic.
Rutin, also named as globulariacitrin and rutoside, possess many pharmacological activities including lowering the capillary permeability, anti-inflammatory, anti-anaphylaxis, anti-tumor, anti-bacteria, anti-virus and hepato-protection. Buckwheat rutin is an active ingredient extracted from Fagopyrum esculentum Moench. [F. sagittatum Gilib; Polygonum fagopyrum L.] with abundant resources and mature techniques. Our previous studies have found rutin could inhibit AngII - induced hypertrophy in cultured neonatal rat cardiomyocytes. To investigate the mechanism, intracellular Ca2+ level, the activities and protein expression of CaN, and the expression of proto - oncogene c - fos mRNA in cultured neonatal rat cardiomyocytes induced by AngII were determined. The finding could provide experimental evidence for the development of new drugs for prevention and cure of myocardial hypertrophy.
Experimental
Plant materials and isolation of rutin
Buckwheat, flowers and leaves collected in late autumn from Ku Lun, Inner Mongolia (China), was identified by the Department of Natural Medicinal Chemistry, Hebei United University, Tangshan, China. A voucher specimen is deposited in the Department of Pharmacology, Hebei United University, Tangshan, China. Buckwheat rutin was isolated with the method demonstrated in the literature (1).
Cell culture and induction of hypertrophy
Primary cardiac myocytes were prepared from 1 to 3-day-old Wistar rats hearts following the sequential enzymatic digestion method described previously and were cultured in Dulbecco’s Modified Eagle Medium (DMEM) supplemented with 10% FBS, 100 U/mL penicillin, and 100 μg/mL streptomycin at 37 °C in a 5% CO2 humidified incubator. Hypertrophy of cardiac myocytes was induced by adding 10-7 mol/L AngII, a known promoting growth factor, to serum-free medium (1). All procedures were approved by the Ethical Committee for Laboratory Animals of Hebei United University and were performed in accordance with the Principles in Animal Care and Use of the Committee.
Experimental groups(1)
The primarily cultured cardiac myocytes were synchronized with serum-free DMEM for 24 h as they grew to the proper density, then the cells were grouped as follows:
Control group: Cultured in serum-free DMEM.
Model group: Cultured in serum-free DMEM supplemented with 10-7 mol/L Ang II.
Rutin I group: Cultured in serum-free DMEM supplemented with 0.8 mg/L rutin 30 min prior to Ang II.
Rutin II group: Cultured in serum-free DMEM supplemented with 4.0 mg/L rutin 30 min prior to Ang II.
Rutin III group: Cultured in serum-free DMEM supplemented with 8.0 mg/L rutin 30 min prior to Ang II.
Different dosages of rutin were chosen according to previous studies.
Detection of intracellular Ca2+ level (2)
Cardiac myocytes were treated with various concentrations of rutin for 24 h, then, intracellular Ca2+ levels were detected with Fura-2/AM, the specific indicator of Ca2+. Briefly, cardiac myocytes were loaded with 4 µmol/L Fura-2 / AM (dissolved in dimethyl sulfoxide with 0.02% pluronic) for 30 minutes at 37 °C in a humidified incubator with 95% air /5% CO2. Cells were then washed three times with modified Hanks' buffer containing (mmol/L) NaCl 137, NaHCO3 4.2, NaHPO4 3, KCl 5.4, KH2PO4 0.4, CaCl2 1.3, MgCl2 0.5, MgSO4 0.8, glucose 10, and HEPES 5 (pH 7.4). Fluorescence was determined with the Hitachi - 850 fluorospectrophotometer using dual excitatory wavelengths of 343 and 380 nm and a single-emission wavelength of 520 nm. [Ca2+]i was determined with the equation of Grynkiewicz et al: [Ca2+]i=Kdxß(R-Rmin)/(Rmax-R), where Kd is the dissociation constant for fura 2 - Ca2+ and taken to be 224 nmol/L, ß is the ratio of fluorescence at 380 nm and zero Ca2+ (F380min) and saturating Ca2+ (F380max) conditions, and R is the ratio of fluorescence obtained with excitation at 343 and 380 nm, with min and max subscripts denoting the ratios obtained under Ca2+-free and Ca2+-saturated conditions, respectively. The unit of [Ca2+]i is nmol/ L. The test was repeated for six times.
Preparation of cardiac myocytes and measurement of protein content
Cardiac myocytes were dealed with drugs and AngII for 24 h, and then they were washed thoroughly with PBS for three times and collected, precooled homogenate (50 mmol/L Tris PH7.5, 0.1 mmol/L EGTA, 1 mmol/L EDTA, 0.5 mmol/L DTT, 50 μg/mL PMSF, 50 μg/mL STI, 5 μg/mL leupeptin, 5 μg/mL aprotinin) was added and the cells were broken into pieces by repeated freezing and thawing, and centrifuged at 4 °C, 12000 g for 10 min. The supernatant was obtained and protein content was measured by Brad-ford method.
Measurement of CaN activity
CaN activity was measured by colorimetric method referred to the literature (3) with some modification. Briefly, after 20 μL of above supernatant and 380 μL of substrate reacted at 30 °C for 10 min, 0.5 mmol/L NaCO3 and 0.4 mmol/L EGTA were added to stop the reaction and the absorbance was measured by automatic microplate reader at 410 nm (results expressed as A410nm). The substrates were used for zero setting. Substrate I contained 50 mmol/L Tris-HCl PH 7.4, 0.5 mmol/L DTT, 0.2 mg/mL BSA, 10 mmol/L PNPP, 0.5mmol/L MnCl2, 0.2 mmol/L CaCl2, 0.3 μmol/L CaM. Substrate II contained 3 mmol/L EGTA without CaCl2 and CaM. A410nm with substrate I reflected the activity of total phosphatases, and A410nm with substrate II reflected the activity of other phosphatases except CaN. So, A410nm of CaN was their subtraction. CaN activity was expressed as A410nm/mgpr.
CaN protein expression observed by immunocytochemistry
Primarily cultured cardiac myocytes were seeded in 24-well plate with 10 mm×8 mm sterile coverslips (3×105 cells/mL, 1 mL per hole) and cultured for 48 h prior to 24 h’ synchronization, dealed with drugs for another 24 h before termination, fixed with 4% paraformaldehyde. CaN protein expression was detected using mouse monoclonal antibody raised against rat CaN-α (Wuhan Boster Bio - Engineering Limited Company, China) as first antibody and biotin-labeled goat anti-mouse IgG as second antibody. Photos were analyzed by MoticMed System 6.0A (Beihang University, Beijing, China).
Measurement of c-fos mRNA by RT-PCR
Total RNA was isolated by Trizol and its density and purity were monitored by extra-violet spectro- luminosity instrument. The ratio of A260 /A280 was among 1.8~2.0. Apex time of c-fos mRNA expression stimulated by Ang II was observed and the effect of buckwheat rutin on c-fos mRNA expression at apex time was evaluated by RT-PCR. The following c-fos primers designed by Stepien (4) were used: sense primer 5'-AGC TGA CAG ATA CGC TCC AA-3', and antisense primer 5'-TAG GTG AAG ACA AAG GAA GAC G-3', length of the amplification fragment was 556 bp. The control GAPDH primer: sense primer 5'-TGC TGA GTA TGT CGT GGA G-3', and antisense primer 5'-GTC TTC TGA GTG GCA GTG AT-3'), length of the amplification fragment was 288 bp (Synthesized by Shanghai biological engineering limited company). Destination gene magnification was carried out according to directions of RT-PCR kit. The conditions were as follows: synthesis and pre-denaturation of c-DNA at 37 °C for 60min, one cycle at 95 °C for 5min, amplification of PCR by 35 cycles at 94 °C for 50 s, 60 °C for 45 s and 72 °C for 1 min, and then extension at 72 °C for 7 min. Amplified product of c-fos and GAPDH 5 μL plus 2 μL sample buffer solution were electrophoresed for 40 min in 2% agarose gel at 80V respectively. The electrophoretogram was scanned by gel image analysis instrument and gray scale measurement was carried out through QuantityOne image analysis software. The ratio of c-fos to GAPDH was calculated.
Statistical analysis
Statistical analyses were performed with the GraphPad instat software. Data were expressed as mean ± standard deviation (S.D). Statistical significances were analyzed using the ANOVA test. A value of P < 0.05 was considered significant.
Results and Discussion
Results showed [Ca2+]i in control group, model group, Rutin I group, Rutin II group and Rutin III group were 121 ± 22 nmol/ L, 178 ± 25 nmol/ L (P < 0.01 v.s. control group ), 153 ± 27 nmol/ L, 132 ± 21 nmol/ L (P < 0.05 v.s. model group ), 128 ± 24 nmol/ L (P < 0.05 v.s. model group ) respectively. Buckwheat rutin inhibited increase of [Ca2+]i in Ang II - induced cardiac myocytes remarkably.
CaN activity of 10-7 mol/L Ang II -induced cardiac myocytes was twice more than that of control group. Buckwheat rutin at 0.8 mg/L, 4.0 mg/L, 8.0 mg/L could obviously inhibit the increasing of CaN activity caused by Ang II (P < 0.05, P < 0.01, P < 0.01) and showed dose-dependent relationship (Figure 1). Results of immunocytochemistry showed that in control group, CaN protein mainly presented intracytoplasm in cardiac myocytes especially remarkable around the nuclear membrane, i.e., cytoplasm of cardiac myocytes was brown while the nuclei were almost not stained. As shown in the photos (Figure 2), the brown granulas in the cytoplasm of cardiac myocytes stimulated by Ang II accumulated obviously especially remarkable around the nuclear membrane. The staining in buckwheat rutin treated groups was much lighter than the model group. When analyzed by the MoticMed System 6.0A, the CaN protein expression (OD ratio) in cardiac myocytes of Ang II group (1.541 ± 0.075) was notably increased compared with the control group (0.682 ± 0.038) (P<0.01), whereas, buckwheat rutin at dosages of 0.8 mg/L, 4.0 mg/L, 8.0 mg/L could greatly decrease the Ang II induced expression of CaN protein to 1.503 ± 0.064, 1.488 ± 0.071 and 0.822 ± 0.051 respectively (P<0.01) in a dose-effect relationship (Figure 3).
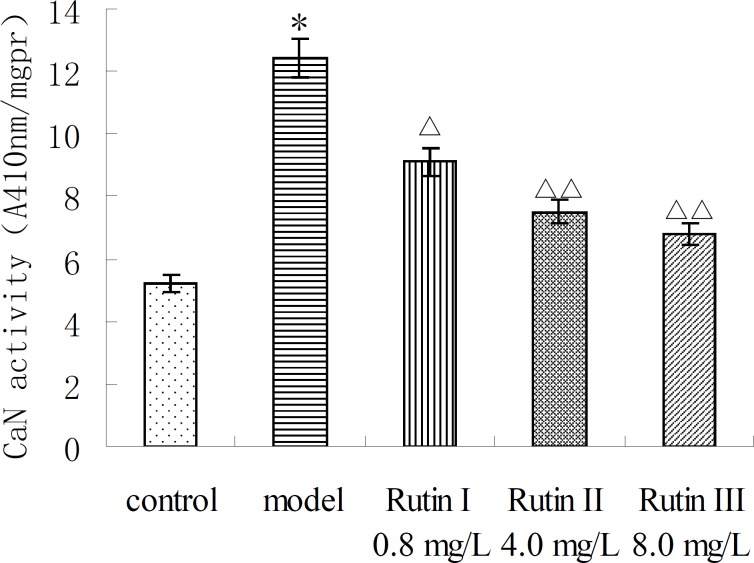
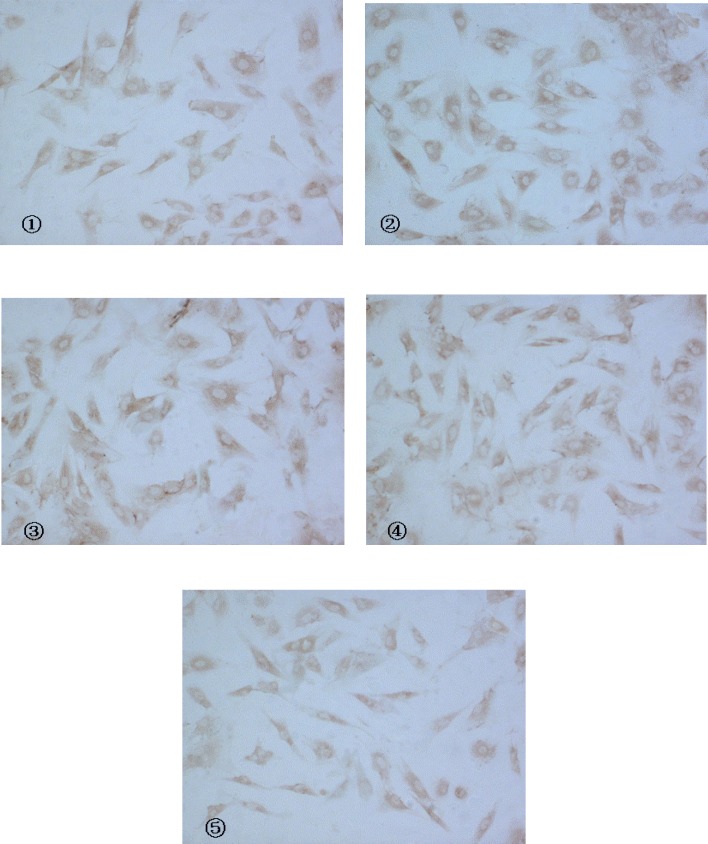
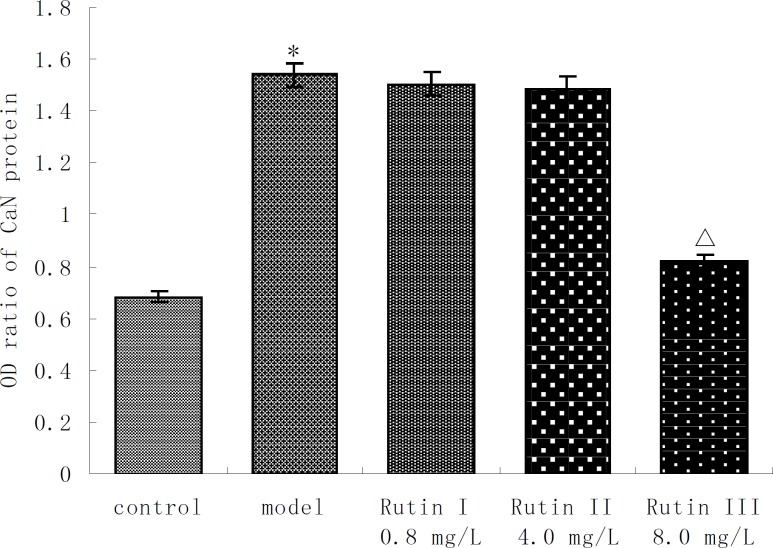
Apex time of c-fos was measured, and the results of five repeated experiments indicated that c-fos mRNA expression started to increase 15min after the action of 10-7 mol/L Ang II in cultured neonatal rat cardiac myocytes, and reached its peak at 30min which was 2.8 times that of control group (P <0.01). After that, the expression of c-fos mRNA began to decrease gradually and nearly went back to normal level in 2 hours. This demonstrated that c-fos mRNA expression was stimulated by Ang II and existed specific time difference (Figure 4). The primarily cultured cardiac myocytes were synchronized with serum-free DMEM for 24 h as they grew to the proper density, then they were treated with different dosages of buckwheat rutin at 30 min prior to Ang II. At the apex time when the expression of c-fos mRNA reached the peak, i.e., 30 min after the addition of Ang II, total RNA was isolated from the cells and the expression of c-fos mRNA was detected. Results showed that c-fos mRNA expression in Ang II group was much higher than that in control group (P < 0.01), while different concentrations of buckwheat rutin could obviously reverse these changes. Compared with Ang II group, c-fos mRNA expression in different concentrations of buckwheat rutin decreased 26% (P < 0.05), 36% (P < 0.01) and 47% (P < 0.01) individually (Table 1, Figure 5).
Effects of buckwheat rutin on c-fos mRNA expression in cardiac myocytes of neonatal rats induced by Ang II.
Group | Dose | c-fos mRNA / GAPDH mRNA(mean ± SD) |
---|---|---|
Control | - | 0.69 ± 0.22 |
Model | 10-7 mol/L Ang II | 1.61 ± 0.35* |
Rutin I | 10-7 mol/L Ang II + 0.8 mg/L rutin | 1.19 ± 0.44△ |
Rutin II | 10-7 mol/L Ang II + 4.0 mg/L rutin | 1.03 ± 0.24△ |
Rutin III | 10-7 mol/L Ang II + 8.0 mg/L rutin | 0.86 ± 0.22△ |
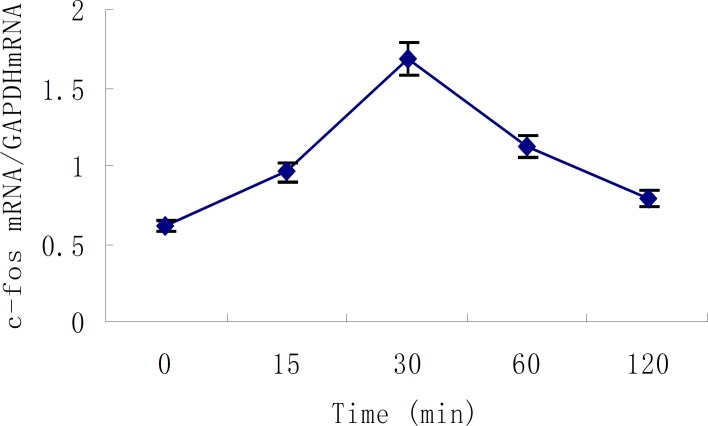
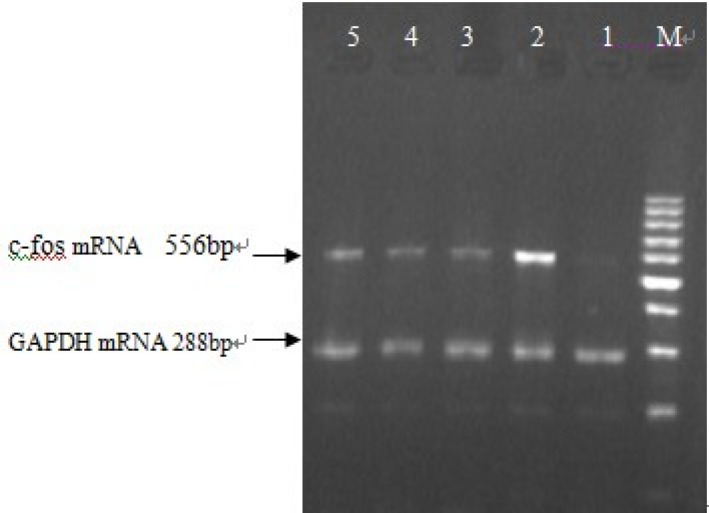
Myocardial hypertrophy and fibrosis are the basic responses of myocardium to the alteration of mechanical load and neurohumor factors. The pathological changes of myocardial hypertrophy mainly include hypertrophy of cardiac myocytes and proliferation of non- cardiac myocytes. Renin-angiotensin system (RAS) is one of the most important reasons besides pressure overloading, nordrenaline and thyrine for myocardial hypertrophy. Ang II, the chief active peptide of RAS, is an effective promoter for cell growth which can facilitate the growth of various cardiovascular cells such as the cardiac myocytes, cardiac fibroblasts and vascular smooth muscle cells (5, 6). In recent years, the role of Ang II in the mechanism of myocardial hypertrophy has been investigated intensively and researchers have found that Ang II acts on AT1 receptor to promote cell growth in a concentration-dependent manner (7). Ang II receptors have two major subtypes, AT1 and AT2 (8, 9), and the former is considered to be present on the surface of human and rat cardiac myocytes with high affinity. Ang II can activate phospholipase C β by stimulating its G - protein coupled receptor (GPCR) subtype Gq/11, thus leads to the production of the second messenger diacylglycerol (DAG) and inositol (1,4,5)-trisphosphate (IP3). DAG can activate PKC, as a result, the cell membrane ion channel Ca-L opens and Ca2+ inflows, meanwhile, PKC can act on the down level protein substrates and promote myocardial hypertrophy. IP3 combines directly with its receptors in sarcoplasmic reticulums and endoplasmic reticulums and causes the release of Ca2+ stored in cellular nuclear envelope gaps to caryoplasms and cytoplasms (10-12). Moreover, AT2 was also reported to play a functional role in the cardiac hypertrophic process in-vivo (13).
Viewed from cellular and molecular levels, myocardial hypertrophy consists of the following three fundamental aspects, i.e., extracellular stimulation, intracellular signal transduction and intranuclear activation and rearrangement of genes, which evoke the cells to hypertrophy phenotype changes. Different stimuli of hypertrophy can cause activation of specific genes and form various types of distinctive myocardial hypertrophy (14), which depends mainly on the signal pathway they trigger. At present, three signal pathways are considered important in inducing myocardial hypertrophy and fibrosis (15), i.e., PKC cascade, MAPK cascade and CaN pathway. CaN is belonged to serine/threonine protein phosphatase, also called protein phosphatase 2 B (PP2B), which is the unique protein phosphatase found to be activated by Ca2+ and calmodulin (CaM). CaN is a heterodimer composed of one 61 KD catalytic subunit CnA and one 19 KD regulatory subunit CnB, and generally distributes in a variety of tissues including the heart. It was reported that all the increase of intracellular Ca2+ from different origins could activate CaN. The nuclear factors of activated T cells (NFAT3) could be dephosphorylated by activated CaN, enter the cell nuclei, and act on the zinc finger transcription factor GATA4 to cause hypertrophy of cardiac myocytes and expression of embryo genes (16-19). Hypertrophy could be suppressed in transgenic mice expressing excessive CaN when given specific inhibitor cyclosporin A to them after birth (20). This illustrated that CaN signal transduction exerted significant role in myocardial hypertrophy process. Furthermore, it was reported that CaN dependent signal pathway participated in Ang II - induced hypertrophy of cardiomyocytes and proliferation of cardiac fibroblasts (21-23). Proto-oncogene c-fos is one of the earlier period respond genes, the expression of which could be induced by many stimuli causing myocardial hypertrophy such as Ang II, endothelin, heart pressure overloading by coarctation of abdominal aorta, and dragging of cultured myocardial cells. The excessively expressed c-fos is an important intranuclear transcription factor in the development of myocardial hypertrophy.
Buckwheat rutin is a major component of buckwheat flavonoids, and rutin exists in no grains other than buckwheat. Previous studies (1, 24) have found that buckwheat rutin could inhibit the hypertrophy of neonatal rat cardiac myocytes induced by Ang II. Our results showed that buckwheat rutin could inhibit the increasing of above parameters in Ang II -induced cardiac myocytes, which manifested that buckwheat could inhibit hypertrophy of Ang II -induced neonatal cardiac myocytes by blockade of CaN - dependent signal pathway. This provided potent experimental basis for the development of drugs preventing and treating cardiac hypertrophy. As it should be, the signal transduction pathways inside cardiac myocytes are rather complex, so the mechanism of buckwheat rutin need further investigation.
Acknowledgements
References
-
1.
Han SY, Chu JX, Li GM, Zhu LS, Shi RF. Effects of rutin from leaves and flowers of buckwheat (Fagopyrum esculentum Moench.) on angiotensin II-induced hypertrophy of cardiac myocytes and proliferation of fibroblasts. Lat. am J. Pharm. 2010;29:137-140.
-
2.
Kanaya N, Murray PA, Damron DS. The differential effects of midazolam and diazepam on intracellular Ca2+ transients and contraction in adult rat ventricular myocytes. Anesth. Analg. 2002;95:1637-1644. [PubMed ID: 12456430].
-
3.
Shang FJ, Zhao LY, Zheng QS, Wang JP. Inhibitory effect of cyclosporin A on growth and collagen synthesis of rat cardiac fibroblasts induced by arginine vasopressin. Acta Pharm. Sinica. 2006;41:1044-1049.
-
4.
Stepien O, Gogusev J, Zhu DL, Iouzalen L, Herembert T, Drueke TB, Marche P. Amlodipine inhibition of serum-, thrombin-, or fibroblast growth factor-induced vascular smooth-muscle cell proliferation. J. Cardiovasc. Pharmacol. 1998;31:786-793. [PubMed ID: 9593080].
-
5.
Schorb W, Booz GW, Dostal DE, Conrad KM, Chang KC, Baker KM. Angiotensin II is mitogenic in neonatal rat cardiac fibroblasts. Circ. Res. 1993;72:1245-1254. [PubMed ID: 8495553].
-
6.
Dostal DE, Baker KM. The Cardiac Renin-Angiotensin System Conceptual, or a Regulator of Cardiac Function? Circ. Res. 1999;85:643-650. [PubMed ID: 10506489].
-
7.
Delbosc S, Cristol JP, Descomps B, Mimran A, Jover B. Simvastatin prevents angiotensin II–induced cardiac alteration and oxidative stress. Hypertension. 2002;40:142-147. [PubMed ID: 12154104].
-
8.
Regitz-Zagrosek V, Fielitz J, Fleck E. Myocardial angiotensin receptors in human hearts. Basic Res. Cardiol. 1998;93:37-42. [PubMed ID: 9833160].
-
9.
Brilla CG, Zhou G, Rupp H, Maisch B, Weber KT. Role of angiotensin II and prostaglandin E2 in regulating cardiac fibroblast collagen turnover. Am. J. Cardiol. 1995;76:8-13.
-
10.
Dolmetsch RE, Lewis RS, Goodnow CC, Healy JI. Differential activation of transcription factors induced by Ca2+ response amplitude and duration. Nature. 1997;386:855. [PubMed ID: 9126747].
-
11.
Bers DM, Perez-Reyes E. Ca2+ channels in cardiac myocytes: structure and function in Ca2+ influx and intracellular Ca2+ release. Cardiovasc Res. 1999;42:339-360. [PubMed ID: 10533572].
-
12.
Anger M, Lompré AM, Vallot O, Marotte F, Rappaport L, Samuel JL. Cellular distribution of Ca2+ pumps and Ca2+ release channels in rat cardiac hypertrophy induced by aortic stenosis. Circulation. 1998;98:2477-2486. [PubMed ID: 9832495].
-
13.
Lakó-Futó Z, Szokodi I, Sármán B, Földes G, Tokola H, Ilves M, Leskinen H, Vuolteenaho O, Skoumal R, Ruskoaho H. Evidence for a functional role of angiotensin II type 2 receptor in the cardiac hypertrophic process in vivo in the rat heart. Circulation. 2003;108:2414-2422. [PubMed ID: 14568903].
-
14.
Calderone A, Takahashi N, Izzo Jr NJ, Thaik CM, Colucci WS. Pressure-and volume-induced left ventricular hypertrophies are associated with distinct myocyte phenotypes and differential induction of peptide growth factor mRNAs. Circulation. 1995;92:2385-2390. [PubMed ID: 7586335].
-
15.
Sugden PH. Signaling pathways in cardiac myocyte hypertrophy. Ann. Med. 2001;33:611-622. [PubMed ID: 11817656].
-
16.
Zhu ZM, Yang YJ, Zhu SJ. Inositol 1, 4, 5-trisphosphate receptor/calcineurin signaling pathway mediated cardiac hypertrophy. Chinese J. Cardiol. 2002;30:680-683.
-
17.
Zhu SJ, Yang YJ, Yu LJ, Huang L. CaN-NFAT3 signal pathway: a crucial hinge relates Ca2+ signal with cardiomyocyte hypertrophy. Chinese J. Int. Med. 2004;43:19-21.
-
18.
Yang YJ, Zhu SJ, Zhu ZM, Hu HY, Ding G, Zhong J. Effect of intracelluar calcium regulation on the growth of cultured cardiomyocytes. Chinese J. Cardiol. 2001;29:45-47.
-
19.
Schulz RA, Yutzey KE. Calcineurin signaling and NFAT activation in cardiovascular and skeletal muscle development. Dev. Biol. 2004;266:1-16. [PubMed ID: 14729474].
-
20.
Molkentin JD, Lu JR, Antos CL, Markham B, Richardson J, Robbins J, Grant SR, Olson EN. A calcineurin-dependent transcriptional pathway for cardiac hypertrophy. Cell. 1998;93:215-228. [PubMed ID: 9568714].
-
21.
White M, Montezano AC, Touyz RM. Angiotensin II signalling and calcineurin in cardiac fibroblasts: differential effects of calcineurin inhibitors FK506 and cyclosporine A. Ther. Adv. Cardiovasc Dis. 2012;6:5-14. [PubMed ID: 22184128].
-
22.
Xu S, Fu M, Xu Y, Pang Y, Tang C. Role of calcineurin in cardiac fibroblast proliferation stimulated by angiotensin II. Acta physiologica Sinica. 2000;52:305-307. [PubMed ID: 11951112].
-
23.
Han ZM, Yang YH, Zhang KL, Xiang JZ. Effects of cyclosporine A on angiotensin II-induced cardiomyocyte hypertrophy in neonatal rats. J. Huazhong. Univ. Sci. Technolog. Med. Sci. 2005;25:558-560. [PubMed ID: 16463674].
-
24.
Chu JX, Li GM, Han SY, Shi RF, Zhu LS. In-vitro inhibitory effects of rutin from buckwheat flowers and leaves on angiotensin Ⅱ-induced cardiomyocytes hypertrophy of neonatal rat. West China J. Pharm. Sci. 2010;25:428-430.