Abstract
Keywords
Multidrug resistance ATP-binding cassette transporter ABCG2 MCF-7 Celecoxib
Introduction
Cyclooxygenases-2 (COX-2) plays an important role in the prostaglandins production involved in pathophysiological processes like inflammation and carcinogenesis (1, 2). COX-2 has been characterized as a critical prognostic factor in numerous solid tumors (3). Numerous studies showed that specific COX-2 inhibitors (coxibs) enhance the efficacy of different anticancer therapy methods through inhibition of cell cycle progression, induction of apoptosis, inhibition of angiogenesis and decreased invasive potential of tumor cells (4-6). Another mechanism by which COX-2 inhibitors could sensitize cells to chemotherapeutic drugs is functional blockade of membrane transporter proteins of the ATP binding cassette transporter (ABC-transporter) family (7, 8).
The development of intrinsic or acquired resistance to a wide variety of anticancer drugs is a major obstacle to successful cancer chemotherapy. One of the mechanisms by which human cancers develop multidrug resistance is the overexpression of efflux transport proteins on the plasma membrane of cancer cells. ATP-binding cassette sub-family G member 2 (ABCG2), a novel known protein, is an efflux pump which transports a variety of xenobiotics and endogenous compounds across cellular membranes. Tissue localization of ABCG2 indicates that ABCG2 plays an important role in absorption, distribution and elimination of its substrates (9, 10). Alteration in ABCG2 expression and activity can significantly affect the disposition of the transporter drug substrates, so it's over expression in cancer cells is responsible for decreasing in drug concentration within the cell and a reduced cancer-chemotherapy efficacy (9, 11).
Previously, we showed that proinflammatory cytokines (IL-1β and TNF-α) significantly increased the levels of ABCG2 mRNA, protein expression and function, while dexamethasone (an anti-inflammatory drug) reduced its expression and activity (12-16). The present study aimed to examine the relationship between the inhibition of COX-2 and expression of ABCG2 in parental and resistant breast cancer cell lines. Understanding the relationship between COX-2 and ABCG2 may open novel perspectives in cancer therapy, in which, coxibs could be employed as a chemotherapy-supporting treatment. Introducing coxibs into the treatment regimen will then augment efficiency of chemotherapy and maybe helpful in prevention or inhibition of the development of the multidrug resistance (MDR) phenomenon.
Experimental
Chemicals and antibodies
TPA (12-O-tetradecanoylphorbol-13-acetate), BSA and penicillin–streptomycin were purchased from Sigma–Aldrich (Germany). Celecoxib was generousely provided by Daru Pakhsh (Tehran, Iran). RPMI 1640 with L-glutamine and FBS were purchased from Biosera (UK) and Gibco (USA), respectively.
Cell culture
The ABCG2-overexpressing, mitoxantrone-resistance epithelial breast cancer cell line, MCF-7/MX, and its parental line, MCF-7 were generously provided by Dr. Erasmus Schneider (Wadsworth Center, New York State Department of Health, USA) and MDA-MB-231 (COX-2 expressing cell line) was generously provided by Dr. Mohammad Kazem Koohi (Faculty of Veterinary Medicine, university of Tehran, Iran). Cells were cultured in RPMI-1640, supplemented with 2 mM of L-glutamine, heat inactivated FBS 10% (v/v), penicillin (50 U/mL), and streptomycin (50 μg/mL) at 37 °C in humidified air containing CO2 5%. Since FBS has been shown to induce COX-2 (due to the presence of growth factors (17)), before treatment, subconfluent cells were washed with PBS and changed to serum-free medium containing BSA 0.1% (w/v) for 24 h. Mitoxantrone (MX) (100 nM) was added to MCF-7/MX cells to maintain the multidrug-resistant phenotype. MCF-7/MX cells were cultured on MX free for at least 7 days prior to experiments.
Reverse transcription-real-time polymerase chain reaction (RT-PCR)
Total cellular RNA was extracted using a High Pure RNA Isolation Kit from Roche Applied Science (Cat. No. 11828665001). The eluted RNA samples were stored at –80 °C for later analysis or directly used in RT-PCR. In order to evaluate the effects of celecoxib on ABCG2 and COX-2 expression, comparative Ct methods using EXPRESS One-Step SYBR® GreenER™ Universal (Invitrogen) were performed and analyzed on a Real time cycler Mx3000P™ Stratagen (Stratagen, USA ). The primers had the following sequences: ABCG2: 5´- TATCAATGGGATCATGAAACCTGG-3´ (forward) and 5´-GCGGTGCTCCATTTATCAGAAC-3´(reverse); COX-2, 5´-AATCATTCACCAGGCAAATTG- 3´ (forward ) and 5´-TCTGTACTGCGGGTGGAACA- 3´ (reverse ); β-actin: 5´-TCATGAAGTGTGACGTGGACATC-3´ (forward) and 5´-CAGGAGGAGCAATGATCTTGATCT-3´ (reverse). Relative mRNA levels of the target gene in each sample were normalized to its β-actin content. The results were expressed as ratio of relative quantity to calibrator of the treated samples divided by relative quantity to calibrator of untreated sample.
Flow cytometric detection of COX-2 protein expression
For intracellular detection of COX-2 by flow cytometry, breast cancer cells were fixed and permeabilised using the Leucoperm™ fixation and permeabilization solution, following the manufacturer's instructions (18). Thereafter, the cells were blocked with BSA 10 % (w/v) and incubated with 50 ng of Phycoerythrin (PE) -conjugated anti-human COX-2 for 30 min. Finally samples were washed, resuspended in PBS and analysed by PartecTM cytometer with 585/42 band pass filter (FL2). Relative expression of COX-2 was calculated, comparing the values of mean fluorescence intensity (MFI) of treated samples to the values of MFI of untreated sample.
Flow cytometric detection of ABCG2 protein expression
The ABCG2 protein expression was measured with the BXP-21 monoclonal antibodies, which recognize an internal epitope of the ABCG2 protein as we previously described (12, 14). In brief, after fixation and permeabilization, the cells were blocked with bovine serum albumin (BSA) 10% (w/v) for 1 h at room temperature. Thereafter, the cells were incubated for 60 min on ice with anti-ABCG2 mAb BXP-21 (1:100). Cells were washed with phosphate buffered saline/bovine serum albumin and incubated on ice for 20 minutes with Fluorescein isothiocyanate (FITC) -conjugated goat anti-mouse antibody (1:50) to detect the primary anti-ABCG2 mAb. FITC fluorescence was measured on a PartecTM cytometer with 530/30 nm band pass filter (FL1). Flow cytometry data were processed and analyzed using FloMax version 2.52 and WinMdi version 2.8 software. All assays were performed in at least three independent experiments. Relative expression of ABCG2 was determined as the ratio of MFI of treated samples divided by MFI of untreated sample.
Statistical analyses
Statistical analyses were carried out using SPSS 16.00 software. Results (mean ± S.D.) were reported as three independent experiments each performed in triplicate. The statistical significance of the results was calculated using multivariate analysis of variance (MANOVA) with Tukey’s post-hoc or Student’s t-test and p-values < 0.05 were considered significant.
Results
ABCG2 and COX-2 expression in MCF7 cells
In order to assess ABCG2 and COX-2 expression in MCF7 cells, the expression of these two genes was evaluated in MCF7 and control cell lines (MCF7-MX and MDA-MB-231cells) by real time RT-PCR. As shown in Figure 1, ABCG2 mRNA level in resistant cell line MCF7-MX was ≈ 850 and 5200 times more than the ABCG2 mRNA level in MCF-7 and MDA-MB-231 cells, respectively. The expression of COX-2 in MDA-MB-231 cells was ≈ 5.5 and 9.2 times more than MCF-7 and MCF7-MX cells, respectively (Figure 1). These data are further strengthened by the results of flowcytometry analyses for ABCG2 and COX-2 protein expression (data not shown).
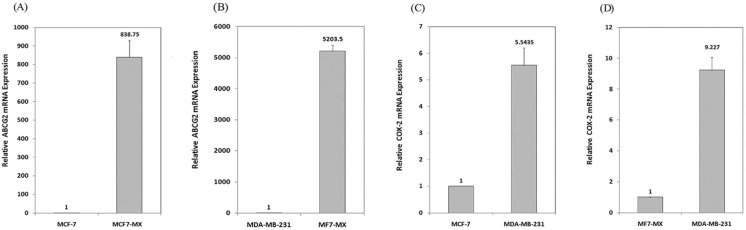
Effects of celecoxib on COX-2 expression
To evaluate the expression of COX-2 mRNA under celecoxib treatment, three cell lines were treated with non-toxic concentration of TPA (10 nM) and celecoxib (0-40 µM) for 4-24 h (15). Expression of COX-2 was studied using Real-time RT-PCR. We have previously reported that TPA was a potent stimulator of COX-2 expression in breast cancer cell lines; as it stimulates COX-2 expression up to 4.5 fold vs. control by 4 h in MDA-MB-231 cells (15). Figure 2 indicates that celecoxib reverses the effects of TPA on COX-2 expression to the control level in MDA-MB-231 by 4 h. Celecoxib significantly inhibited COX-2 expression by 12 and 24 h in MCF-7 and by 24 h in MCF7-MX (data not shown). To verify whether the inhibitory effects of celecoxib on COX-2 expression could be observed also in protein level, cells were treated for 4 and 24 h without or with TPA 10 nM, celecoxib 40 µM, or both. Then, the cells were incubated with the specific COX-2 antibody. As shown in Figure 3, MDA-MB-231 cells treated with TPA displayed an increased COX-2 protein expression, which was significantly reduced by co-treatment with celecoxib.
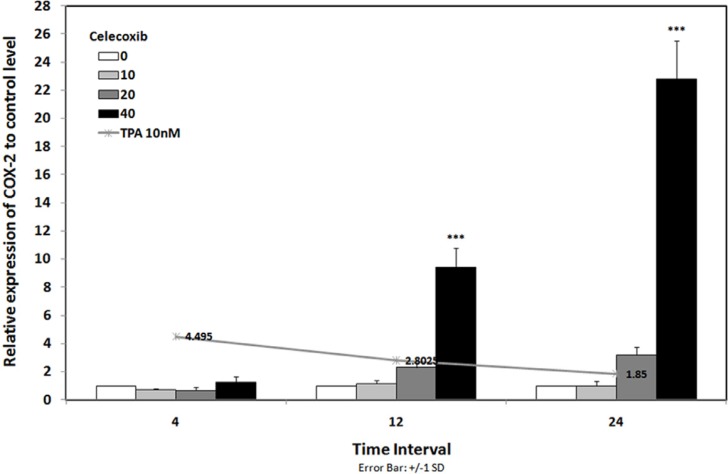
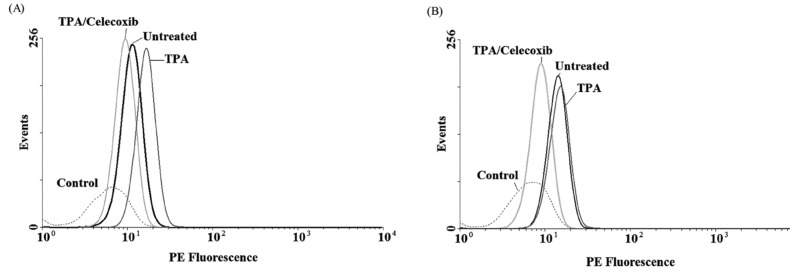
Effects of celecoxib on ABCG2 gene expression
To verify whether ABCG2 gene expression could be influenced by incubation with celecoxib, real time RT-PCR analysis was performed on RNA from breast cancer cells previously treated for 4, 12 and 24 h with TPA 10 nM in combination with celecoxib 0-40 µM. The results showed that celecoxib enhanced the effects of TPA on ABCG2 expression in MCF-7 and MCF7-MX. In MCF-7 (Figure 4a), ABCG2 expression increased up to 1.62 times under treatment with TPA 10 nM for 12 h while combination treatment with celecoxib 40 µM / TPA 10 nM caused up to 2.67 times increased in ABCG2 expression to control level in the same incubation time. As shown in Figure 4b, celecoxib 20 µM induced ABCG2 expression from 1.69 times (under TPA 10 nM treatment) to 2.29 times to control level by 4 h in MCF7-MX cells. On the other hand, celecoxib reversed the TPA effects on ABCG2 expression in MDA-MB-231 cell line. We previously reported that TPA significantly reduced ABCG2 expression to about 0.2 times to control level by 12 and 24 h (18), while as shown in Figure 4c, celecoxib stimulated the expression of ABCG2 up to 4.27 times to control level by 12 h in MDA-MB-231 cells.
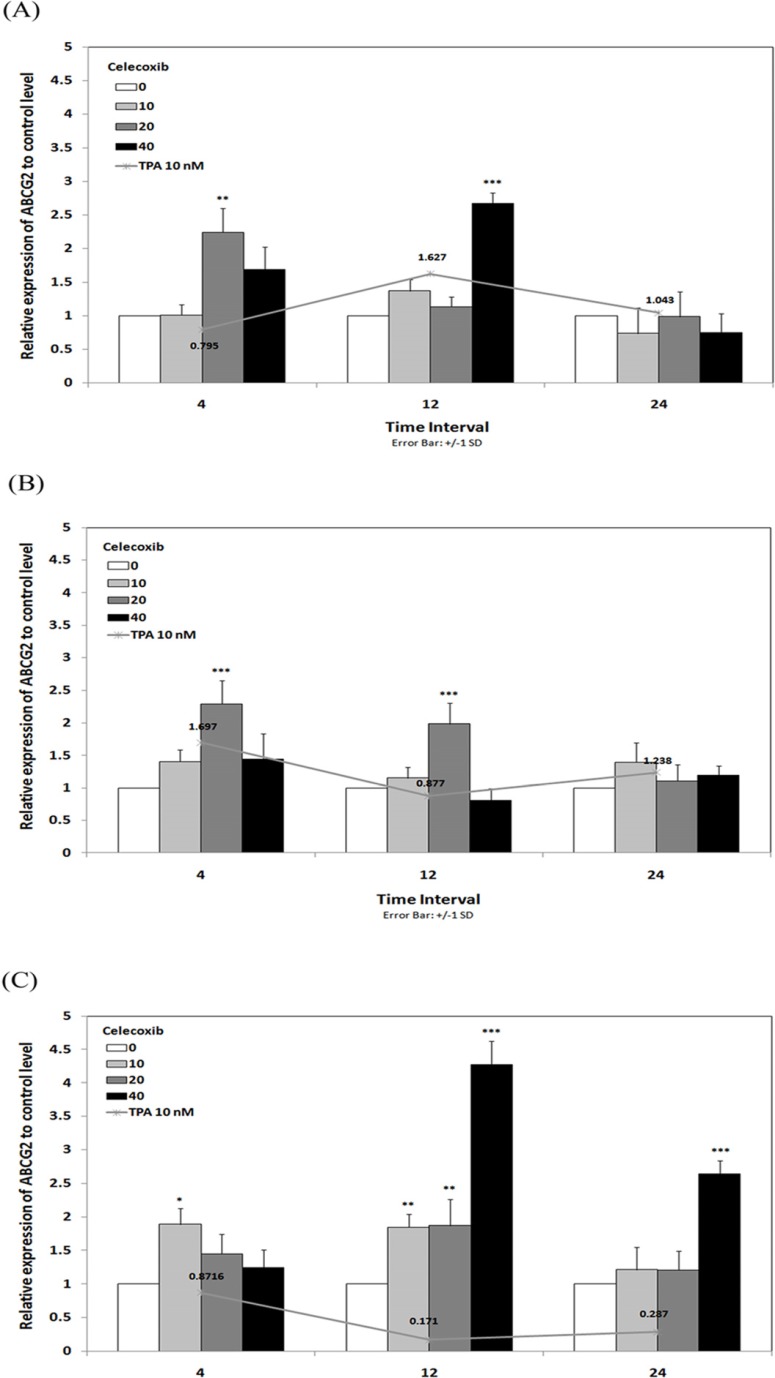
Influence of celecoxib on ABCG2 protein expression
In order to verify whether the observed effects at mRNA level were mirrored by parallel changes in ABCG2 protein levels, flow cyotmtry analysis for ABCG2 was performed using breast cancer cells treated for 4 and 24 h with TPA 10 nM and celecoxib 40 µM. We previously showed that TPA could cause a small increase in ABCG2 expression in MCF-7, on the contrary, treatment of MCF7-MX and MDA-MB-231 cells with TPA resulted in a small decrease in ABCG2 protein expression (18). As indicated in Figure 5, celecoxib reversed the inhibitory effects of TPA on ABCG2 protein expression and increased its expression to the basal level in MCF7-MX and MDA-MB-231. Co-treatment of MCF-7 cells with TPA and Celecoxib caused increased ABCG2 protein expression to a small amount more than TPA lonely (Figure 5c).
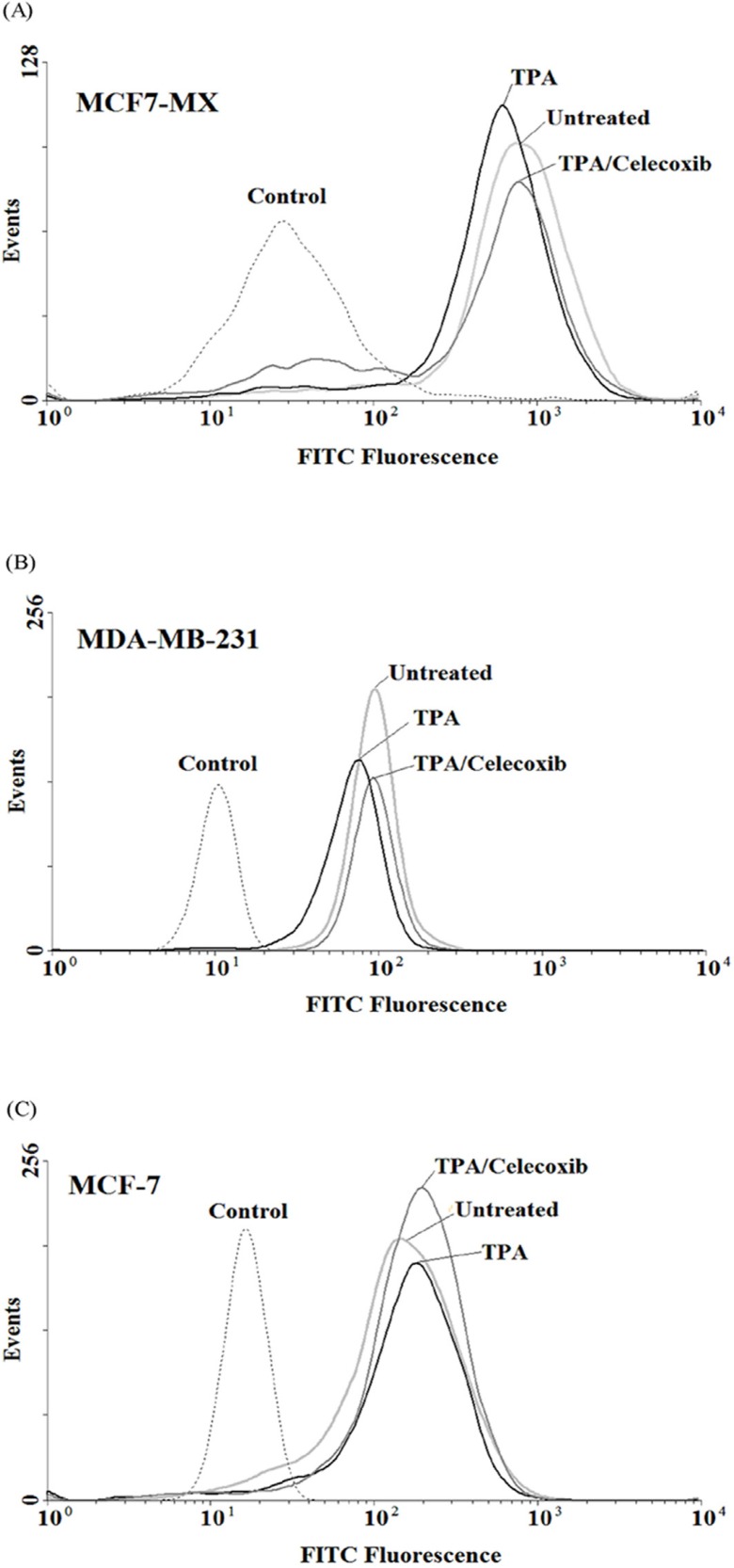
Discussion
ABCG2 is an important member of the ABC- transporter family, which functions as pumps to extrude anticancer drugs from cancer cells, by this means causing MDR in cancer patients (9). This study tested the hypothesis that COX-2 inhibitor celecoxib plays a role in regulating ABCG2 expression in human breast cancer cells. Recent studies indicated that the activation of the cyclooxygenase system is correlated with the increased expression of MDR1 in acute myeloid leukemia HL-60 cells, primary rat hepatocytes, renal rat mesangial cells as well as breast cancer patients (7, 19-21). While, selective COX-2 inhibitors have been shown to increase sensitivity of cancer cells to chemotherapy via reduction of drug resistance in human patients with AML, breast, ovarian and colon cancer (3, 19, 22, 23). On the other hand, in this study we showed that celecoxib up-regulated ABCG2 mRNA expression in drug sensitive MCF-7 cells.
Our finding indicated that celecoxib reduced TPA-induced COX-2 mRNA expression at 10 µM while treatment with celecoxib 40 µM resulted in a significant overstimulation of COX-2 expression. On the other hand, celecoxib 40 µM inhibits TPA induced COX-2 expression at protein level (Figure 2 and 3). Our results are in good agreement with Niederberger et al. who showed that celecoxib 1 µM slightly reduced the IL-1β-induced COX-2 expression at both mRNA and protein level but increased COX-2 mRNA and protein expression at 50 µM. It has been shown that transcription factor NF-κB regulates the transcription of the COX-2 gene. On the other hand, high concentrations of celecoxib induced the activation of NF-kB, so activated NF-kB would stimulate the transcription of NF-kB-dependent genes such as COX-2 (24).
In this study, we reported that treatment of MCF-7 ,MCF7-MX and MDA-MB-231 cells with specific COX-2 inhibitor celecoxib up-regulates ABCG2 expression at both mRNA and protein levels. In the same way, Zrieki et al. showed that treatment of human colorectal Caco-2 cell line with COX-1/ COX-2 inhibitor naproxen led to an stimulation of ABCG2 expression corresponding to the significant decrease of rodamine 123 (Rho123) retention achieved in activity study. In contrast, treatment with selective COX-2 inhibitors nimesulide did not influence the expression of ABCG2 in protein level (25). Another study indicated that sulindac induced MRP1 and MRP3 gene expression via a ROS-related, COX-2-independent mechanism in human colorectal cancer cell line HCT15 (26). Kang et al. reported that enforced COX-2 expression in human lung carcinoma cell line H460 did not cause increase in MRP1 expression, while the celecoxib COX-2 inhibitor reduced the expression and function of MRP1 protein. They suggested that celecoxib down-regulated MRP1 expression with a COX-independent mechansim (27). In contrast, several studies have shown that specific COX-2 inhibitors could prevent or reduce the development of chemoresistance phenotype by downregulation of the expression and activity of P-glycoprotein (MDR1) (8, 26, 28-30). These inconsistencies may be due to the diversity among species, tissue origins, or cell types.
In conclusion, our findings provide evidence that selective COX-2 inhibitor celecoxib induces ABCG2 mRNA and protein expression in the sensitive and drug resistant MCF7 breast cancer cell lines. These data may indicate a possible COX-2 independent mechanism for the stimulatory effect of celecoxib on ABCG2. Further studies are needed to determine whether other COX-2 selective inhibitors will show the similar effects to celecoxib and to clarify the molecular mechanism by which they induce ABCG2 expression.
Acknowledgements
References
-
1.
Trebino CE, Stock JL, Gibbons CP, Naiman BM, Wachtmann TS, Umland JP, Pandher K, Lapointe JM, Saha S, Roach ML, Carter D, Thomas NA, Durtschi BA, McNeish JD, Hambor JE, Jakobsson PJ, Carty TJ, Perez JR, Audoly LP. Impaired inflammatory and pain responses in mice lacking an inducible prostaglandin E synthase. Proc. Natl. Acad. Sci. USA. 2003;100:9044-9049. [PubMed ID: 12835414].
-
2.
Razmi A, Zarghi A, Arfaee S, Naderi N, Faizi* M. Evaluation of Anti-nociceptive and Anti-inflammatory Activities of Novel Chalcone Derivatives. Iran. J. Pharm. Res. 2013;12:153-159. [PubMed ID: 24250683].
-
3.
Surowiak P, Materna V, Matkowski R, Szczuraszek K, Kornafel J, Wojnar A, Pudelko M, Dietel M, Denkert C, Zabel M, Lage H. Relationship between the expression of cyclooxygenase 2 and MDR1/P-glycoprotein in invasive breast cancers and their prognostic significance. Breast Cancer Res. 2005;7:862-870.
-
4.
Hashitani S, Urade M, Nishimura N, Maeda T, Takaoka K, Noguchi K, Sakurai K. Apoptosis induction and enhancement of cytotoxicity of anticancer drugs by celecoxib, a selective cyclooxygenase-2 inhibitor, in human head and neck carcinoma cell lines. Int. J. Oncol. 2003;23:665-672. [PubMed ID: 12888902].
-
5.
Masferrer JL, Leahy KM, Koki AT, Zweifel BS, Settle SL, Woerner BM, Edwards DA, Flickinger AG, Moore RJ, Seibert K. Antiangiogenic and antitumor activities of cyclooxygenase-2 inhibitors. Cancer Res. 2000;60:1306-1311. [PubMed ID: 10728691].
-
6.
Gasparini G, Longo R, Sarmiento R, Morabito A. Inhibitors of cyclo-oxygenase 2: a new class of anticancer agents? Lancet Oncol. 2003;4:605-615. [PubMed ID: 14554238].
-
7.
Patel VA, Dunn MJ, Sorokin A. Regulation of MDR-1 (P-glycoprotein) by cyclooxygenase-2. J. Biol. Chem. 2002;277:38915-38920. [PubMed ID: 12138126].
-
8.
Zatelli MC, Luchin A, Piccin D, Tagliati F, Bottoni A, Vignali C, Bondanelli M, degli Uberti EC. Cyclooxygenase-2 inhibitors reverse chemoresistance phenotype in medullary thyroid carcinoma by a permeability glycoprotein-mediated mechanism. J. Clin. Endocrinol. Metab. 2005;90:5754-5760. [PubMed ID: 16091477].
-
9.
Mao Q, Unadkat JD. Role of the breast cancer resistance protein (ABCG2) in drug transport. Aaps. J. 2005;7:118-133.
-
10.
Krishnamurthy P, Ross DD, Nakanishi T, Bailey-Dell K, Zhou S, Mercer KE, Sarkadi B, Sorrentino BP, Schuetz JD. The stem cell marker Bcrp/ABCG2 enhances hypoxic cell survival through interactions with heme. J. Biol. Chem. 2004;279:24218-24225. [PubMed ID: 15044468].
-
11.
Sarkadi B, Ozvegy-Laczka C, Nemet K, Varadi A. ABCG2 - a transporter for all seasons. FEBS Lett. 2004;567:116-120. [PubMed ID: 15165903].
-
12.
Mosaffa F, Lage H, Afshari JT, Behravan J. Interleukin-1 beta and tumor necrosis factor-alpha increase ABCG2 expression in MCF-7 breast carcinoma cell line and its mitoxantrone-resistant derivative, MCF-7/MX. Inflamm Res. 2009;58:669-676. [PubMed ID: 19333723].
-
13.
Mosaffa F, Kalalinia F, Parhiz BH, Behravan J. Tumor Necrosis Factor Alpha Induces Stronger Cytotoxicity in ABCG2-Overexpressing Resistant Breast Cancer Cells Compared with Their Drug-Sensitive Parental Line. DNA Cell Biol. 2011.
-
14.
Elahian F, Kalalinia F, Behravan J. Dexamethasone downregulates BCRP mRNA and protein expression in breast cancer cell lines. Oncol Res. 2009;18:9-15. [PubMed ID: 19911699].
-
15.
Kalalinia F, Elahian F, Behravan J. Potential role of cyclooxygenase-2 on the regulation of the drug efflux transporter ABCG2 in breast cancer cell lines. J. Cancer Res. Clin. Oncol. 2011;137:321-330. [PubMed ID: 20422426].
-
16.
Elahian F, Kalalinia F, Behravan J. Evaluation of indomethacin and dexamethasone effects on BCRP-mediated drug resistance in MCF-7 parental and resistant cell lines. Drug Chem. Toxicol. 2010;33:113-119. [PubMed ID: 20307139].
-
17.
Shen SC, Ko CH, Hsu KC, Chen YC. 3-OH flavone inhibition of epidermal growth factor-induced proliferaton through blocking prostaglandin E2 production. Int. J. Cancer. 2004;108:502-510. [PubMed ID: 14696113].
-
18.
Kalalinia F, Elahian F, Hassani M, Kasaeeian J, Behravan J. Phorbol Ester TPA Modulates Chemoresistance in the Drug Sensitive Breast Cancer Cell Line MCF-7 by Inducing Expression of Drug Efflux Transporter ABCG2. Asian Pac. J. Cancer Prev. 2012;13:2979-2984. [PubMed ID: 22938493].
-
19.
Puhlmann U, Ziemann C, Ruedell G, Vorwerk H, Schaefer D, Langebrake C, Schuermann P, Creutzig U, Reinhardt D. Impact of the cyclooxygenase system on doxorubicin-induced functional multidrug resistance 1 overexpression and doxorubicin sensitivity in acute myeloid leukemic HL-60 cells. J. Pharmacol. Exp. Ther. 2005;312:346-354. [PubMed ID: 15501994].
-
20.
Ziemann C, Schafer D, Rudell G, Kahl GF, Hirsch-Ernst KI. The cyclooxygenase system participates in functional mdr1b overexpression in primary rat hepatocyte cultures. Hepatol. 2002;35:579-588.
-
21.
Ratnasinghe D, Daschner PJ, Anver MR, Kasprzak BH, Taylor PR, Yeh GC, Tangrea JA. Cyclooxygenase-2, P-glycoprotein-170 and drug resistance; is chemoprevention against multidrug resistance possible? Anticancer Res. 2001;21:2141-2147. [PubMed ID: 11501838].
-
22.
Raspollini MR, Amunni G, Villanucci A, Boddi V, Taddei GL. Increased cyclooxygenase-2 (COX-2) and P-glycoprotein-170 (MDR1) expression is associated with chemotherapy resistance and poor prognosis. Analysis in ovarian carcinoma patients with low and high survival. Int. J. Gynecol. Cancer. 2005;15:255-260. [PubMed ID: 15823108].
-
23.
Saikawa Y, Sugiura T, Toriumi F, Kubota T, Suganuma K, Isshiki S, Otani Y, Kumai K, Kitajima M. Cyclooxygenase-2 gene induction causes CDDP resistance in colon cancer cell line, HCT-15. Anticancer Res. 2004;24:2723-2728. [PubMed ID: 15517878].
-
24.
Niederberger E, Tegeder I, Vetter G, Schmidtko A, Schmidt H, Euchenhofer C, Brautigam L, Grosch S, Geisslinger G. Celecoxib loses its anti-inflammatory efficacy at high doses through activation of NF-kappaB. Faseb. J. 2001;15:1622-1624. [PubMed ID: 11427506].
-
25.
Zrieki A, Farinotti R, Buyse M. Cyclooxygenase inhibitors down regulate P-glycoprotein in human colorectal Caco-2 cell line. Pharm. Res. 2008;25:1991-2001. [PubMed ID: 18581209].
-
26.
Kim SK, Lim SY, Wang KC, Kim YY, Chi JG, Choi YL, Shin HJ, Cho BK. Overexpression of cyclooxygenase-2 in childhood ependymomas: role of COX-2 inhibitor in growth and multi-drug resistance in-vitro. Oncol Rep. 2004;12:403-409. [PubMed ID: 15254709].
-
27.
Kang HK, Lee E, Pyo H, Lim SJ. Cyclooxygenase-independent down-regulation of multidrug resistance-associated protein-1 expression by celecoxib in human lung cancer cells. Mol. Cancer Ther. 2005;4:1358-1363. [PubMed ID: 16170027].
-
28.
Zatelli MC, Luchin A, Tagliati F, Leoni S, Piccin D, Bondanelli M, Rossi R, degli Uberti EC. Cyclooxygenase-2 inhibitors prevent the development of chemoresistance phenotype in a breast cancer cell line by inhibiting glycoprotein p-170 expression. Endocr. Relat. Cancer. 2007;14:1029-1038. [PubMed ID: 18045954].
-
29.
Huang L, Wang C, Zheng W, Liu R, Yang J, Tang C. Effects of celecoxib on the reversal of multidrug resistance in human gastric carcinoma by downregulation of the expression and activity of P-glycoprotein. Anticancer Drugs. 2007;18:1075-1080. [PubMed ID: 17704658].
-
30.
Roy KR, Reddy GV, Maitreyi L, Agarwal S, Achari C, Vali S, Reddanna P. Celecoxib inhibits MDR1 expression through COX-2-dependent mechanism in human hepatocellular carcinoma (HepG2) cell line. Cancer Chemother. Pharmacol. 2010;65:903-911. [PubMed ID: 19685055].