Abstract
Keywords
Introduction
Zirconium dioxide (ZrO2), an essential trace metal, is a silvery, lustrous, strong transition metal which is very similar to titanium and hafnium (1-2). In recent years, usage of Zirconium dioxide nanoparticles (nano-ZrO2) is rapidly growing in biological fields. they are widely used as drug delivery carriers for some medicines like itraconazole, penicillin, alendronate and zoledronate (-); as gene delivery vehicles with target specificity for some tissues (6-7); for improving the properties of traditional bone cements in orthopedia (8); and some other purposes such as production of poisons like parathion (9) or nerve agents (10).
Although ZrO2 is a neutral bioceramic metal, but it is propounded that the introduction of these materials into clinical practice may be associated with some short- and long-term risks in living systems. For example, a significant DNA damage in human T-cells (11), an induction of apoptosis in human mesenchymal stem cells (12), and inhibition of cell proliferation in human mesothelioma (MSTO-211H) and rodent fibroblast cell lines (13) are reported after exposure to zirconium.
Increased levels of reactive oxygen species (ROS) are involved in DNA damage and apoptosis because they damage cellular macromolecules including lipids, proteins and nucleic acids (14). Neurons are especially sensitive to the oxidative stress due to their high rate of oxidative metabolic activity and having relatively low amounts of endogenous antioxidants (15-16). As a result of high ATP demand of the brain, O2 is consumed rapidly and free radicals are a constant production in this tissue.
Tissue and cellular damage can be resulted from extensive ROS production in inflammatory disease (17). Superoxide (O2) and its product hydrogen peroxide (H2O2) can even produce more reactive species like hydroxyl radical, hypochlorous acid and singlet oxygen which can damage the components of extracellular matrix (18-19). Moreover, localized effect of ROS will continue to the production of extra cytokine and more damage (20). Cell signaling episodes can be initiated in macrophages by H2O2 which lead to the activation of transcriptional factor NF-κB (19, 21).
Studies have shown that, in turn, cell can defense itself with multiple antioxidant enzymes including superoxide dismutase (SOD), Glutathione Peroxidase (GPx) and catalase (CAT) (22-23). The GPx enzyme belongs to a family of selenoproteins whose function is to catalyze the reduction of various peroxides (24). GSH-Px acts as the second line of defense by converting peroxide into water and molecular form of oxygen. Especially, in mammalian cells it plays a critical role to protect them from oxidative stress (25-26).
An imbalance between the productions of free radicals and the cell ability for detoxifying these radicals is involved in molecular mechanism of neurotoxicity (27-28).
Since, the cytotoxic effects of nano-ZrO2 has not been investigated before, this study was designed to elucidate the effect of these nanoparticles on viability and GPx enzyme activity of N2a and PC12 cells.
Experimental
Cell lines and reagents
Commercial nano-ZrO2 (<100 nm in average size) were purchased from Sigma-Aldrich (St Louis, MO). PC12 and N2a cell lines, derived from rat pheochromocytoma and mouse neuroblastoma, respectively, were purchased from Pasteur Institute (Tehran, Iran). High glucose (4.5 g/L) Dulbecco’s Modified Eagles Medium (DMEM) and fetal calf serum were purchased from Gibco (Carlsbad, CA). 3-(4,5-dimethylthiazol-2-yl)-5-(3-carboxymethoxyphenyl)-2-(4-sulfophenyl)-2H-tetrazolium (MTS) was obtained from Promega (Madison, WI, USA). Glutathione Peroxidase (GPx) Assay kit was purchased from Northwest Life Science Specialties, LLC (Vancouver, WA USA).
Dispersion and characterization of the zirconia nanoparticles
Nano-ZrO2 was suspended in media and dispersed by an ultrasonic bath for 60 min. Then the size of nanoparticles in suspension was characterized by Transmission Electron Microscopy (TEM, LEO 912AB, Germany), using one drop of sample on a carbocoated grid and high voltage (120K.V.) imagining TEM.
Cell culture and nanoparticle treatment
The cells were maintained in Dulbecco's modified Eagle's medium (DMEM) containing 10% fetal calf serum, penicillin 100 IU/mL, and streptomycin 100 µg/mL. They were grown and maintained in 75 cm2 cell culture flasks at 37 oC in a 5% CO2 humidified incubator. The test suspension of nano-ZrO2 was prepared using the culture media and dispersed for 20 min by using a sonicator (Bandelin electronic, DT 510, Germany) to prevent aggregation. The cells were treated with various concentrations of nanoparticles according to the time schedule.
Cell viability assay
The cell viability assay is based on the reduction of novel tetrazolium compound (MTS) by mitochondrial dehydrogenase in metabolically active cells to the water-soluble formazan (29). About 104 cells were seeded in each well of a 96-microwell plate and treated with various concentrations (0-2000 µg/mL) of nanoparticle suspension for 12, 24 and 48 h. At the end of the treatment, the MTS reagent, which is composed of the novel tetrazolium compound and an electron coupling reagent phenazine ethosulfate, was added to the cell media. After 3 h, cell viability was determined by measuring the optical absorbance at 490 nm using an ELISA microplate reader (Awareness, Palm City, FL, USA). The cytotoxicity of nano-ZrO2 was calculated using Graph Pad Software (Graph Pad prism 5 software) and presented as mean ± SEM of three independent experiments with three replicates for each concentration.
Determination of glutathione peroxidase activity
Glutathione peroxidase activity was determined using a sensitive kit produced based on the method of Paglia and Valentine (30). In this method, glutathione (GSH) is oxidized to form oxidized glutathione (GSSG) which is then reduced by glutathione reductase (GR) and b-nicotinamide adenine dinucleotide phosphate (NADPH) forming NADP+ (resulting in decreased absorbance at 340 nm) and recycling the GSH. Because GPx is limiting, the decrease in the absorbance is directly proportional to the GPx concentration.
Cells were seeded in 6-well tissue culture plates with 106 cells per mL media. After allowing the cells to be seeded for 24 h, nanoparticle suspension with different concentration (2000, 1000, 250, 62.5, 31.25 µg/mL) were added to each well for 12, 24 and 48 h. Then, Cells were washed with PBS, scraped and homogenized in 20 mM PBS containing 0.5 mM butylated hydroxyltoluene to prevent sample further oxidation. The homogenate was centrifuged at 3,000 g for 20 min at 4
Statistical analysis
The results are presented as the mean ± SEM. The values were compared using the one-way analysis of variance (ANOVA) followed by Tukey’s post tests for multiple comparisons. The p<0.05 was considered to be statistically significant.
Results
Characterization of nano-ZrO2
The size of the nano-ZrO2 was found to be less than 100 nm as shown in the images of Transmission Electron Microscopy (Figure 1).
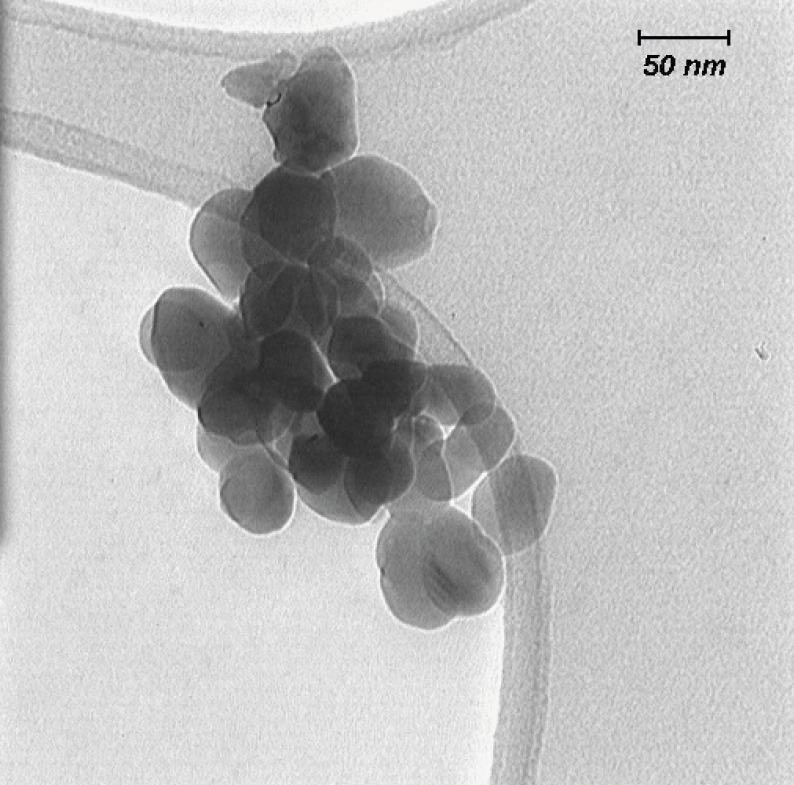
Effect of nano-ZrO2 on cell viability
The effect of nano-ZrO2 on viability of N2a cells are shown in Figure 2. After 12 h incubation, cell viability was significantly decreased at concentrations of ≥15.6 µg/mL. As compared with untreated cells (100 ± 9.3 %), the nano-ZrO2 at 2000 µg/mL decreased the cell viability to 32.76 ± 2.8 (P < 0.001), which was lowest viability amount among all the concentrations. Similarly, exposure of the N2a cells to the nanoparticles for 24 and 48 h showed a concentration-dependent decrease in cell viability. Again the cytotoxic effect was observed at concentrations more than 15.6 µg/mL (P < 0.05 and P<0.001) after 24 and 48 h exposure, respectively. No significant difference in cell viability was found between the three incubation times.
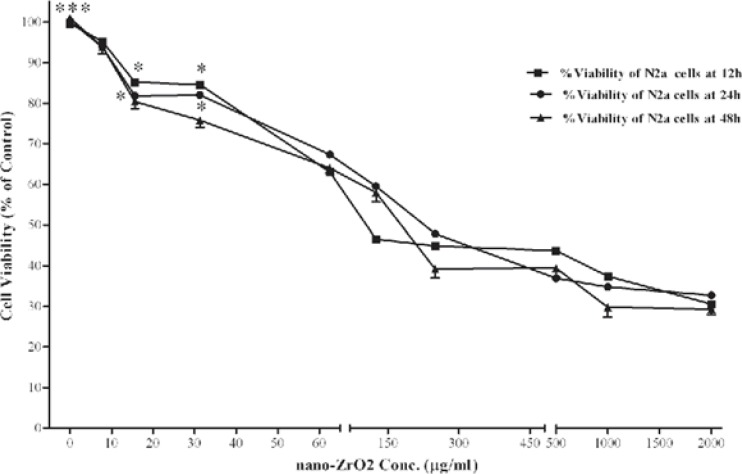
Figure 3 shows the effect of nano-ZrO2 on viability of PC12 cells. After 12 h, the level of viability in the cells exposed to 31.25, 62.5, 125, 250, 500, 1000 and 2000 µg/mL of nano-ZrO2 was 80.90 ± 5.1, 69.66 ± 7.2, 66.23 ± 7.0, 69.29 ± 7.1, 51.75 ± 6.9, 48.45 ± 7.9, and 46.46 ± 3.5 %, respectively, which at concentrations more than 31.25 µg/mL was significantly (P < 0.05) lower than that of untreated cells (100 ± 5.2 %). Approximately, the same level of viability was observed when incubation time extended to 24 h. However, 48 h incubation with nano-ZrO2 leads to more reduction of cell surviving, so that, its cytotoxicity was started at concentrations of ≥15.6 µg/mL (P < 0.05).
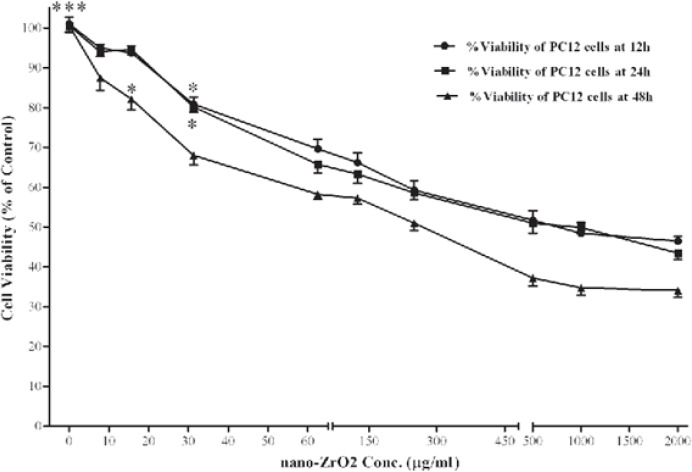
Effect of nano-ZrO2 on glutathione peroxidase activity
The influence of nano-ZrO2 on GPx enzyme is determined with decreasing its activity comparing to the untreated group which this reduction is started from 250 µg/mL (p < 0.001) after 12 h exposure of N2a cell line (Figure 4A) and it is distributed to lower concentration via rising the duration of exposure time up to 24 h and 48 h which are 62.5 µg/mL and 31.25 µg/mL (p < 0.05) (Figure 5A and 6A), respectively.
For PC12 cell line, there is almost the same condition. In this case the level of enzyme activity is decreasing from concentration 250 µg/mL (p < 0.01) after 12 h and 24 h exposure (Figure 4B and 5B) and from concentration 31.25 µg/mL (p < 0.05) after 48 h incubation with nano-ZrO2 (Figure 6B).
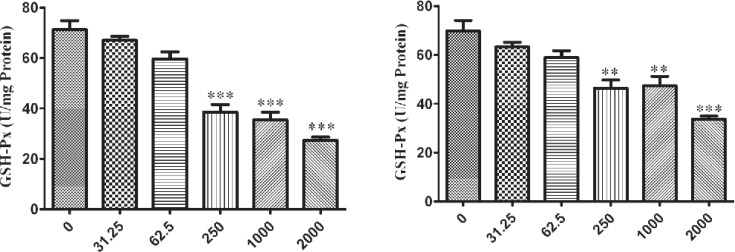
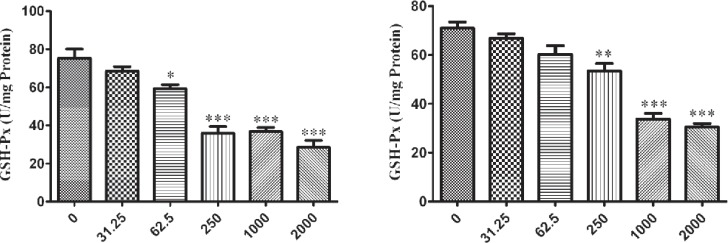
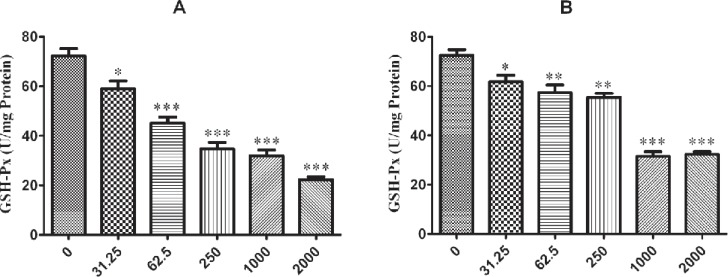
Discussion
Considering the increased usage of zirconia nanoparticles in many aspects of biological field, more attention should be considered to their safety or toxicity. The particles can enter the body via inhalation, skin penetration, ingestion or via metallic implant corrosion (31-33). It has been well documented that zirconium dioxide can induce cell cytotoxicity by its own. For instance, Caicedo and his colleagues show that zirconium ion at concentrations more than 5 mM can induce apoptosis in T-Helper Jurkat Cells (11). Moreover, another study showed that zirconia with a particle size around 0.6 µm induces apoptosis through increase of TNF-α release in J744 macrophage cells (34-35).
In this work for the first time we showed that nano-ZrO2 particles have antiproliferative effect on N2a and PC12 cell cells. These neuronal cell lines have extensively used in researches to test possible neurotoxic or neuroprotective effects of compounds (36-38). Assessment of nano-ZrO2 cytotoxicity through the MTS assay revealed a dose dependent toxic effect on the neuronal cell viability in a concentration range of 31 to 2000 µg/mL. On the other hand, this effect was not changed while incubation time increased, which suggests that the peak of nano-ZrO2 cytotoxicity occurs during first 12 h exposure.
The correlation between oxidative stress and decrease of cell viability has been well documented (39-40). The unwanted effects of oxidative stress is resulted from increasing the lipid peroxidation, DNA damage and apoptosis induction (41). The mitochondrial thiol proteins, in particular GPx, have a critical role in protecting the cell against redox signaling and cell death programming (42). Here we showed that nano-ZrO2 decrease GPx activity and therefore oxidative stress may be, in part, involved in the cytotoxicity effect of this nanoparticle. Our finding is in accordance with what olmedo and his colleagues revealed in 2011 about the effect of zirconia on oxidant–antioxidant balance in tissues (43). Also it was previously reported that submicron Zirconia increases the release of superoxide (O2-) and nitric oxide by Human THP-1 Macrophages (19). Taken together, direct interaction of nano-ZrO2 with cell membrane and/or accumulation of free radicals such as HO2•, O2•, HO• and carbon-centered radicals as a result of GPx activity decrease, can initiate oxidative damage and induce cell apoptosis (40, 44-46).
In conclusion, our study showed that nano-ZrO2 at concentrations more than 250 µg/mL has neurotoxic effects through increasing the oxidative stress and this should be considered for therapeutic use.
Acknowledgements
References
-
1.
Greenwood NN. Chemistry of the Elements. Oxford: Butterworth-Heinemann; 1997. p. 954-975.
-
2.
Schroeder HA, Balassa JJ. Abnormal trace metals in man: zirconium. J. Chronic Dis. 1966;19:573-586. [PubMed ID: 5338082].
-
3.
Catauro M, Raucci M, Ausanio G. Sol-gel processing of drug delivery zirconia/polycaprolactone hybrid materials. J. Mater. Sci. Mater. Med. 2008;19:531-540. [PubMed ID: 17619979].
-
4.
Colilla M, Manzano M, Izquierdo-Barba I, Vallet-Regi M, Boissiere C, Sanchez C. Advanced drug delivery vectors with tailored surface properties made of mesoporous binary oxides submicronic spheres. Chem. Materials. 2009;22:1821-1830.
-
5.
Nakarani M, Nakarani M, Misra AK, Patel JK, Vaghani SS. Itraconazole nanosuspension for oral delivery: Formulation, characterization and in-vitro comparison with marketed formulation. Daru. 2010;18:84-90. [PubMed ID: 22615599].
-
6.
Tan K, Cheang P, Ho IA, Lam PY, Hui KM. Nanosized bioceramic particles could function as efficient gene delivery vehicles with target specificity for the spleen. Gene Ther. 2007;14:828-835. [PubMed ID: 17344903].
-
7.
Link N, Brunner TJ, Dreesen IA, Stark WJ, Fussenegger M. Inorganic nanoparticles for transfection of mammalian cells and removal of viruses from aqueous solutions. Biotechnol. Bioeng. 2007;98:1083-1093. [PubMed ID: 17546691].
-
8.
Gillani R, Ercan B, Qiao A, Webster TJ. Nanofunctionalized zirconia and barium sulfate particles as bone cement additives. Int. J. Nanomed. 2010;5:1-11.
-
9.
Parham H, Rahbar N. Square wave voltammetric determination of methyl parathion using ZrO2-nanoparticles modified carbon paste electrode. J. Hazard Mater. 2010;177:1077-1084. [PubMed ID: 20097474].
-
10.
Liu G, Lin Y. Electrochemical sensor for organophosphate pesticides and nerve agents using zirconia nanoparticles as selective sorbents. Anal. Chem. 2005;77:5894-5901. [PubMed ID: 16159119].
-
11.
Caicedo M, Jacobs JJ, Reddy A, Hallab NJ. Analysis of metal ion-induced DNA damage, apoptosis, and necrosis in human (Jurkat) T-cells demonstrates Ni2+ and V3+ are more toxic than other metals: Al3+, Be2+, Co2+, Cr3+, Cu2+, Fe3+, Mo5+, Nb5+, Zr2+. J. Biomed. Mater. Res. A. 2008;86:905-913. [PubMed ID: 18050301].
-
12.
Wang ML, Tuli R, Manner PA, Sharkey PF, Hall DJ, Tuan RS. Direct and indirect induction of apoptosis in human mesenchymal stem cells in response to titanium particles. J. Orthop. Res. 2003;21:697-707. [PubMed ID: 12798071].
-
13.
Brunner TJ, Wick P, Manser P, Spohn P, Grass RN, Limbach LK, Bruinink A, Stark WJ. In-vitro cytotoxicity of oxide nanoparticles: comparison to asbestos, silica, and the effect of particle solubility. Environ. Sci. Technol. 2006;40:4374-4381. [PubMed ID: 16903273].
-
14.
Sadeghnia HR, Assadpour E, Boroushaki MT, Ghorbani A. Protective effect of safranal, a constituent of crocus sativus, on quinolinic acid-induced oxidative damage in rat hippocampus. Iran. J. Basic. Med. Sci. 2013;16:73-82. [PubMed ID: 23638295].
-
15.
Doyle KP, SR Stenzel-Poore MP. Mechanisms of ischemic brain damage. Neuropharmacol. 2008;55:310-318.
-
16.
Maier CM, Chan PH. Role of superoxide dismutases in oxidative damage and neurodegenerative disorders. Neuroscientist. 2002;8:323-334. [PubMed ID: 12194501].
-
17.
Greenwald RA. Oxygen radicals, inflammation, and arthritis: pathophysiological considerations and implications for treatment. Semin. Arthritis Rheum. 1991;20:219-240. [PubMed ID: 2042055].
-
18.
Daumer KM, AU Khan, Steinbeck MJ. Chlorination of pyridinium compounds. Possible role of hypochlorite, N-chloramines, and chlorine in the oxidation of pyridinoline cross-links of articular cartilage collagen type II during acute inflammation.J. Biol. Chem. 2000;275:34681-34692.
-
19.
Wang ML, Hauschka PV, Tuan RS, Steinbeck MJ. Exposure to particles stimulates superoxide production by human THP-1 macrophages and avian HD-11EM osteoclasts activated by tumor necrosis factor-alpha and PMA. J. Arthroplasty. 2002;17:335-346. [PubMed ID: 11938511].
-
20.
Tucci M, Baker R, Benghuzzi H, Hughes J. Levels of hydrogen peroxide in tissues adjacent to failing implantable devices may play an active role in cytokine production. Biomed. Sci. Instrum. 2000;36:215-220. [PubMed ID: 10834235].
-
21.
Kang JL, Go YH, Hur KC, Castranova V. Silica-induced nuclear factor-kappaB activation: involvement of reactive oxygen species and protein tyrosine kinase activation. J. Toxicol. Environ. Health A. 2000;60:27-46. [PubMed ID: 10832616].
-
22.
Wang J, Sun P, Bao Y, Dou B, Song D, Li Y. Vitamin E renders protection to PC12 cells against oxidative damage and apoptosis induced by single-walled carbon nanotubes. Toxicol. In-vitro. 2012;26:32-41. [PubMed ID: 22020378].
-
23.
Halliwell B. Role of Free Radicals in the Neurodegenerative Diseases. Drugs Aging. 2001;18:685-716. [PubMed ID: 11599635].
-
24.
Bhabak KP, Mugesh G. Functional mimics of glutathione peroxidase: bioinspired synthetic antioxidants. Acc. Chem. Res. 2010;43:1408-1419. [PubMed ID: 20690615].
-
25.
Contestabile A. Oxidative stress in neurodegeneration: mechanisms and therapeutic perspectives. Curr. Top. Med. Chem. 2001;1:553-568. [PubMed ID: 11895131].
-
26.
Wang J, Sun P, Bao Y, Dou B. Vitamin E renders protection to PC12 cells against oxidative damage and apoptosis induced by single-walled carbon nanotubes. Toxicol. In-vitro. 2012;26:32-41. [PubMed ID: 22020378].
-
27.
Patel VP, Chu CT. Nuclear transport, oxidative stress, and neurodegeneration. Int. J. Clin. Exp. Pathol. 2011;4:215-229. [PubMed ID: 21487518].
-
28.
Circu ML, Aw TY. Reactive oxygen species, cellular redox systems, and apoptosis. Free Radic. Biol. Med. 2010;48:749-762. [PubMed ID: 20045723].
-
29.
Malich G, Markovic B, Winder C. The sensitivity and specificity of the MTS tetrazolium assay for detecting the in-vitro cytotoxicity of 20 chemicals using human cell lines. Toxicol. 1997;124:179-192.
-
30.
Paglia DE, Valentine WN. Studies on the quantitative and qualitative characterization of erythrocyte glutathione peroxidase. J. Lab. Clin. Med. 1967;70:158-169. [PubMed ID: 6066618].
-
31.
Flatebo RS, Johannessen AC, Gronningsaeter AG, Boe OE, Gjerdet NR. Host response to titanium dental implant placement evaluated in a human oral model. J. Periodontol. 2006;77:1201-1210. [PubMed ID: 16805683].
-
32.
Revell P. The biological effects of nanoparticles. Nanotechnol. Percep. 2006;2:283-298.
-
33.
Oberdorster G, Oberdorster E, Oberdorster J. Nanotoxicology: an emerging discipline evolving from studies of ultrafine particles. Environ. Health Perspect. 2005;113:823-839. [PubMed ID: 16002369].
-
34.
Catelas I, Huk OL, Petit A, Zukor DJ, Marchand R, Yahia L. Flow cytometric analysis of macrophage response to ceramic and polyethylene particles: effects of size, concentration, and composition. J. Biomed. Mater. Res. 1998;41:600-607. [PubMed ID: 9697033].
-
35.
Catelas I, Petit A, Zukor DJ, Marchand R, Yahia L, Huk OL. Induction of macrophage apoptosis by ceramic and polyethylene particles in-vitro. Biomaterials. 1999;20:625-630. [PubMed ID: 10208404].
-
36.
Ghorbani A, Rakhshandeh H, Asadpour E, Sadeghnia HR. Effects of Coriandrum sativum extracts on glucose/serum deprivation-induced neuronal cell death. Avicenna J. Phytomed. 2011;2:4-9.
-
37.
Sadeghnia HR, Farahmand SK, Asadpour E, Rakhshandeh H, Ghorbani A. Neuroprotective effect of Lactuca sativa on glucose/serum deprivation-induced cell death. Afr. J. Pharm. Pharmacol. 2012;6:2464-2471.
-
38.
Mortazavian SM, Ghorbani A. Antiproliferative effect of viola tricolor on neuroblastoma cells in-vitro. Aust. J. Med. Herbalism. 2012;24:93-96.
-
39.
Sadeghnia HR, Kamkar M, Assadpour E, Boroushaki MT, Ghorbani A. Protective Effect of Safranal, a Constituent of Crocus sativus, on Quinolinic Acid-induced Oxidative Damage in Rat Hippocampus. Iran. J. Basic. Med. Sci. 2013;16:73-82. [PubMed ID: 23638295].
-
40.
Khan MA, Chen HC, Wan XX, Tania M, Xu AH, Chen FZ, Zhang DZ. Regulatory effects of resveratrol on antioxidant enzymes: A mechanism of growth inhibition and apoptosis induction in cancer cells. Mol. Cells. 2013;35:219-225. [PubMed ID: 23456297].
-
41.
Takahashi M. Oxidative stress and redox regulation on in-vitro development of mammalian embryos. J. Reprod. Dev. 2012;58:1-9. [PubMed ID: 22450278].
-
42.
Yin F, Sancheti H, Cadenas E. Mitochondrial thiols in the regulation of cell death pathways. Antioxid. Redox. Signal. 2012;17:1714-1727. [PubMed ID: 22530585].
-
43.
Olmedo DG, Tasat DR, Evelson P, Rebagliatti R, Guglielmotti MB, Cabrini RL. In-vivo comparative biokinetics and biocompatibility of titanium and zirconium microparticles. J. Biomed. Mater. Res. A. 2011;98:604-613. [PubMed ID: 21721117].
-
44.
Chen X, Mao SS. Titanium dioxide nanomaterials: synthesis, properties, modifications, and applications. Chem. Rev. 2007;107:2891-2959. [PubMed ID: 17590053].
-
45.
Fenoglio I, Greco G, Livraghi S, Fubini B. Non-UV-induced radical reactions at the surface of TiO2 nanoparticles that may trigger toxic responses. Chem. 2009;15:4614-4621.
-
46.
Ismagilov ZR, Shikina NV, Mazurkova NA, Tsikoza LT, Tuzikov FV, Ushakov VA, Ishchenko AV. Synthesis of nanoscale TiO2 and study of the effect of their crystal structure on single cell response. Sci. World J. 2012;2012:498345.