Abstract
Keywords
Introduction
Cell viability assays are frequently used for drug discovery using high-throughput screening (1), environmental assessment of chemicals (2) and biosensors for monitoring cellular behavior (3). Some biochemical methods, such as the MTT assay are widely used in toxicity screening assays (4, 5).
Rifampin is an important drug in the treatment of human mycobacterial and other infections. It is widely used as an essential drug in the treatment of tuberculosis and leprosy, in combination with other drugs (6). The drug has been shown to produce hepatic toxicity in animal studies (7, 8) and clinical settings (9, 10).
Previously in our laboratory, during the design of a cell-based test distinguishing between the toxicity of compounds per se and their toxicity resulting from their metabolites, we have shown that rifampin is toxic to human hepatoma cell line (HepG2) in concentrations in the range of its Cmax in clinical setting (11). The drug, in agreement with clinical data, did not show any significant toxicity against human larynx carcinoma cell line (Hep2). HepG2 cells are rich in drug metabolizing enzymes (12).
Therefore, it seems that the toxicity of rifampin on HepG2 cells is due to its toxic metabolic products. In order to evaluate this hypothesis, in this study, we investigated the effects of an antioxidant, vitamin C, and a scavenger of active metabolites, NAC, on the toxicity of rifampin on HepG2 cells by MTT assay.
Experimental
Rifampin was kindly provided by Hakim Pharmaceutical Company (Tehran, Iran). The substance was dissolved in DMSO. MTT (tissue culture grade) was purchased from Sigma (USA). DMSO and DMEM powder were purchased from Merck Company (Germany). Gentamicin was purchased as injectable ampoules from Darou Pakhsh Pharmaceutical Company (Tehran, Iran).
Cell cultures and treatments
HepG2 cells were obtained from Pasteur Institute Collection of Cell Cultures (Pasteur Tehran, Iran) (ECACC No. 86121112) and were cultured in DMEM supplemented with 10% FBS and gentamicin (with a final concentration of 100 mg/L) under standard conditions and subcultured in the ratio of 1:3 twice per week. Passages 1-15 were used for experiments. Cells were seeded at a density of 1000 cells/well in 96-well plates (Greiner, UK) and incubations with various concentrations of rifampin were started 24 h after seeding and continued for 48 h.
Cytotoxicity assay
HepG2 cells were cultured in 96-well plates at a concentration of 1 × 103 cells/well, and incubated at 37ºC, in a 5% CO2 incubator. After 24 h, the culture supernatant was replaced with fresh DMEM and different amounts of rifampin were added to produce final concentrations of 10, 20, 50 and 100 μM in culture medium. Appropriate amounts of vitamin C and NAC were also added to produce concentrations of 0.1 and 0.2 mg/mL, respectively. The plates were incubated for 48 h. Then, 20 μL of MTT at a concentration of 5 mg/mL was added to each well. The plates were incubated for 4 h at 37ºC in a 5% CO2 incubator. The growth medium was removed; 200 μL of DMSO and 20 μL of glycine buffer were added and incubated at room temperature for 30 min.
The absorbance of each well was measured by an ELISA reader (Microplate reader MR 600, Dynatech, USA) at a wavelength of 570 nm. Determination of percent of growth inhibition was carried out using the following formula:
Growth inhibition (%) = [(C - T) / C] × 100
Here, C is the mean absorbance of control group and T is the mean absorbance of test group.
Statistical evaluations
The data were expressed as mean ± standard deviation (SD) of 8 independent experiments. Wherever appropriate, the data were subjected to statistical analysis by one-way analysis of variance (ANOVA) followed by Tukey-Kramer test for multiple comparisons. A value of p < 0.05 was considered significant. Instat 3 software was used for the statistical analysis and excel 2003 was used for producing diagrams. IC50 values and corresponding confidence limits (95% CL) were determined by the Litchfield and Wilcoxon method (PHARM/PCS Version 4).
Results and Discussion
Rifampin is a valuable drug in the treatment of human tuberculosis and leprosy. A medical concern in the use of this drug is hepatotoxicity that was reported in its clinical use (9, 10). In our previous study, it was shown that rifampin produces toxicity against HepG2 cell line. This toxicity was not produced against Hep2 (Human laryngeal carcinoma) cells. It appears that phase I metabolism is responsible for the production of reactive metabolites that damage cellular macromolecules and produce lipid peroxidation (11), as these cells are rich in phase I and phase II metabolizing enzymes. They express a wide range of phase I enzymes such as cytochrome P450 (CYP) 1A1, 1A2, 2B, 2C, 3A and 2E1, aryl hydrocarbon hydrolase, nitroreductase, n-demethylase, catalase, peroxidase, NAD(P)H : cytochrome c reductase, cytochrome P450 reductase, and NAD(P)H, Quinone oxidoreductase and phase II enzymes such as epoxide hydrolase, sulfotransferase, glutathione S-transferase (GST), uridine glucuronosyltransferase, and n-acetyltransferase (12).
Toxicity of Rifampin
Figure 1 shows the results of MTT assay in HepG2 cells cultured for 48 h in DMEM containing different concentrations of rifampin (see above). Rifampin produced significant dose-dependent reduction in cell number in 10 (p < 0.01), 20, 50 and 100 (p < 0.001) μM concentrations. The drug reduced the number of cells by 12.32%, 37.58%, 55.63% and 76.42% in 10, 20, 50 and 100 μM, respectively. DMSO did not reduce the number of cells to a significant amount and was not toxic to cells. The IC50 value for rifampin was 25.25 μM. The results are in agreement with what we and others have reported in these cells (11) and other hepatocytes (13).
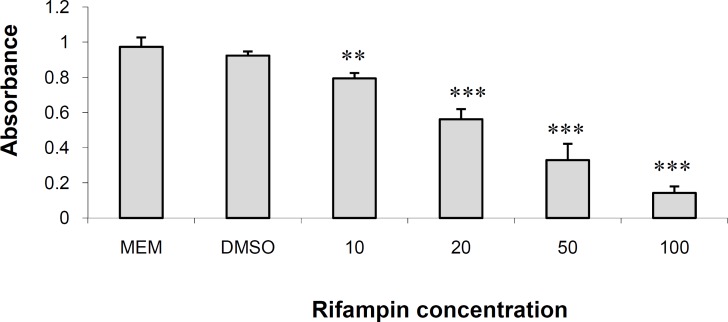
Rifampin is rapidly deacetylated by microsomal oxidative enzymes to the main active metabolite, 25-O-desacetylrifampin. Other metabolites of the drug are rifampin quinine, desacetylrifampin quinine, and 3-formylrifampin (14). In an animal study, rifampin altered and intensity of hepatocyte tight junctions as early as 30 min after a single dose of rifampicin (15). In another study on gel-entrapped rat hepatocytes, toxicity was related to oxidative stress and lipid accumulation, but not via cytochrome P450 involvement (16). They could not observe a significant toxicity in rat hepatocyte monolayer using a concentration of 12 μM of rifampin. This may suggest less sensitivity of rat hepatocyte monolayer to rifampin toxicity, compared with HepG2 cells. In addition, they stopped their experiments in 12 μM concentration, without trying higher concentrations. Besides, in our previous study, damage to cell organelles including nuclear deformation, chromosomal condensation and rough endoplasmic reticulum swelling were observed in HepG2 cells (11). Oxidative stress proposed the mechanism of toxicity in other studies as well (17).
Experiments with vitamin C
As shown in Figure 2, vitamin C at a concentration of 0.1 mg/mL effectively protected HepG2 cells against rifampin-induced toxicity. Vitamin C, at this concentration, completely protected HepG2 cells from rifampin toxicity in rifampin concentrations of 10 and 20 μM. The drug also produced significant protection in rifampin concentrations of 50 and 100 μM (p < 0.001). The IC50 value for rifampin is 30.52 μM and for rifampin plus vitamin C is 99.15 μM.
Vitamin C is an effective antioxidant and free radical scavenger in animal, human and in-vitro studies against oxidative stress and hepatotoxicity (18-20).
In this study, vitamin C could effectively protect HepG2 cells against rifampin-induced toxicity in the concentration of 0.1 mg/mL (Figure 2).
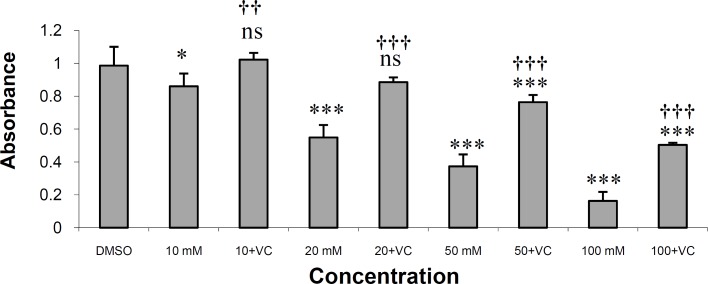
A positive aspect of the effects reported in this study is that vitamin C was effective in the concentrations in the range of its therapeutic levels (21). Some researchers reported a differential negative effect on cancer cell lines for vitamin C compared with the normal cell lines (22, 23). Considering beneficial antimicrobial-enhancing effects of vitamin C (24, 25), in addition to its antioxidant and hepatoprotective effects, makes a promising view of its potential use as a safe protective agent against rifampin-induced hepatotoxicity. Although vitamin C is not used as a prophylaxis and treatment against hepatotoxicity due to various agents, it has been shown that it is an effective prophylactic agent against oxidative stress damage to organs and tissues in animal (13,26) and human atudies (27,19).
Experiments with NAC
Figure 3 shows the result of protection of HepG2 cells from rifampin toxicity by NAC at a concentration of 0.2 mg/mL. N-acetylcysteine at this concentration completely protected HepG2 cells from rifampin toxicity in rifampin concentration of 10 μM. The drug also produced significant protection in rifampin concentrations of 20, 50 and 100 μM (p < 0.001). The IC50 value for rifampin is 25.25 μM and for rifampin plus vitamin C is 73.07 μM.
N-acetylcysteine is also a free-radical scavenger and hepatoprotective agent for the prevention and treatment of clinically significant liver injury in animals (28, 29) and humans (30, 31). Our results indicated that NAC effectively protected HepG2 cells against rifampin-induced toxicity at a concentration of 0.2 mg/mL (Figure 3).
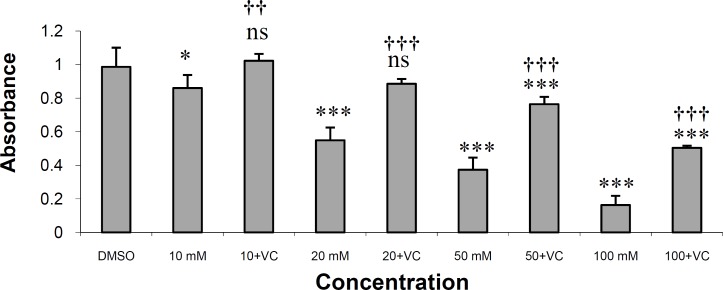
NAC showed a significant protective effect against hepatotoxicity due to the administration of rifampin and isoniazid (32). In addition, Shen et al. showed the reduction of rifampin toxicity by ROS scavenger and NAC in gel-entrapped rat hepatocytes (16). In addition, Liang et al., (2010) and Daraei et al., (2012) found a similar effect for NAC against acetaminophen-induced cytotoxicity on human normal liver L-02 cells and depleted uranium-induced oxidative toxicity in human dermal fibroblast primary cells (33, 34). NAC is a standard drug used for the treatment of acetaminophen toxicity (30). The drug also showed protective effects against mixed antituberculosis drug-induced hepatotoxicity in a human study (35). NAC has different effects on bacterial growth. It shows antibacterial effects against P. aeruginosa and showed synergistic effects with carbenicillin or ticarcillin but antagonizes the effects of gentamicin and tobramycin on this organism (36).
In summary, both agents could effectively protect HepG2 cells against rifampin-induced toxicity with a relatively more potent effect of vitamin C compared with NAC. Both drugs showed antimicrobial-enhancing effects, although not always for NAC (25, 33). For more informative data, it is better to assess oxidative actions of rifampin using the relative biomarkers. We have no idea about the effects of these two agents on antibacterial activity of rifampin against susceptible organisms. In the case of no effect or enhancing effect, they could be promising agents (especially vitamin C because of a better safety profile) for the prevention of hepatotoxicity due to rifampin.
Acknowledgements
References
-
1.
White RE. High-throughput screening in drug metabolism and pharmacokinetic support of drug discovery. Annu. Rev. Pharmacol. Toxicol. 2000;40:133-57. [PubMed ID: 10836130].
-
2.
Ni Shuilleabhain S, Mothersill C, Sheehan D, O’Brien NM, O’Halloran J, Van Pelt FN, Davoren M. In-vitro cytotoxicity testing of three zinc metal salts using established fish cell lines. Toxicol. In-vitro. 2004;18:365-76. [PubMed ID: 15046785].
-
3.
Ehert R, Baumann W, Brischwein M, Schwinde A, Stegbauer K, Wolf B. Monitoring of cellular behaviour by impedance measurements on interdigitated electrode structures. Biosens. Bioelectron. 1997;12:29-41. [PubMed ID: 8976050].
-
4.
Li AP, Lu C, Brent JA, Pham C, Fackett A, Ruegg CE, Silber PM. Cryopreserved human hepatocytes: characterization of drug-metabolizing enzyme activities and applications in higher throughput screening assays for hepatotoxicity, metabolic stability, and drug-drug interaction potential. Chem. Biol. Interact. 1999;121:17-35. [PubMed ID: 10418968].
-
5.
Heussner, A.H, Dietrich, D.R, O Brien, E. In-vitro investigation of individual and combined cytotoxic effects of ochratoxin A and other selected mycotoxins on renal cells. Toxicol. In-vitro. 2005;20:332-41. [PubMed ID: 16140496].
-
6.
Gumbo T. Chemotherapy of tuberculosis, Mycobacterium avium complex disease and leprosy. In: Brunton LL, Chabner BA, Knollmann BC, editors. Goodman and Gilman’s the pharmacological basis of therapeutics. 12th ed. New York: McGrow-hill companies, Inc; 2011. p. 1549-70.
-
7.
Piriou A, Maissiat R, Jacqueson A, Warnet JM, Claude JR. Ultrastructural changes in the parenchymal liver cells of rats treated with high doses of rifampicin. Br. J. Exp. Pathol. 1987;68:201-7. [PubMed ID: 3580280].
-
8.
Sodhi CP, Rana SV, Mehta SK, Vaiphei K, Attari S, Mehta S. Study of oxidative-stress in isoniazid-rifampicin induced hepatic injury in young rats. Drug Chem. Toxicol. 1997;20:255-69. [PubMed ID: 9292280].
-
9.
Page KR, Sifakis F, Montes de Oca R, Cronin WA, Doherty MC, Federline L, Bur S, Walsh T, Karney W, Milman J, Baruch N, Adelakun A, Dorman SE. Improved adherence and less toxicity with rifampin vs isoniazid for treatment of latent tuberculosis: a retrospective study. Arch. Intern. Med. 2006;166:1863-70. [PubMed ID: 17000943].
-
10.
Menzies D, Long R, Trajman A, Dion MJ, Yang J, Al Jahdali H, Memish Z, Khan K, Gardam M, Hoeppner V, Benedetti A, Schwartzman K. Adverse events with 4 months of rifampin therapy or 9 months of isoniazid therapy for latent tuberculosis infection: a randomized trial. Ann. Intern. Med. 2008;149:689-97. [PubMed ID: 19017587].
-
11.
Vahdati-Mashhadian N, Jaafari MR, Nosrati A. Differential toxicity of rifampin on HepG2 and Hep2 cells using MTT test and electron microscope. Pharmacologyonline. 2007;3:405-13.
-
12.
Knasmüller S, Parzefall W, Sanyal R, Ecker S, Schwab C, Uhl M, Mersch-Sundermann V, Williamson G, Hietsch G, Langer T, Darroudi F, Natarajan AT. Use of metabolically competent human hepatoma cells for the detection of mutagens and antimutagens. Mutat. Res. 1998;402:185-202. [PubMed ID: 9675276].
-
13.
Shen C, Cheng X, Li D, Meng Q. Investigation of rifampicin-induced hepatotoxicity in rat hepatocytes maintained in gel entrapment culture. Cell Biol. Toxicol. 2009;25:265-74. [PubMed ID: 18496758].
-
14.
United States Pharmacopeial Convention Inc. USPDI (volume 1, Drug information for the health care professional). . 2597, Micromedex, Englewood, USA; 2001.
-
15.
Chen X, Zhang C, Wang H, Xu J, Duan ZH, Zhang Y, Yu T, Wei W, Xu DX, Xu JM. Altered integrity and decreased expression of hepatocyte tight junctions in rifampicin-induced cholestasis in mice. Toxicol. Appl. Pharmacol. 2009;240:26-36. [PubMed ID: 19577586].
-
16.
Shen C, Cheng X, Li D, Meng Q. Investigation of rifampicin-induced hepatotoxicity in rat hepatocytes maintained in gel entrapment culture. Cell Biol. Toxicol. 2009;25(3):265-74. [PubMed ID: 18496758].
-
17.
Sodhi CP, Rana SV, Mehta SK, Vaiphie K, Attari S, Mehta S. Study of oxidative-stress in isoniazid-rifampicin induced hepatic injury in young rats. Drug Chem. Toxicol. 1997;20:255-69. [PubMed ID: 9292280].
-
18.
Kalender S, Uzun FG, Durak D, Demir F, Kalender Y. Malathion-induced hepatotoxicity in rats: The effects of vitamins C and E. Food Chem. Toxicol. 2010;48:633-8. [PubMed ID: 19941925].
-
19.
Traber MG, Stevens JF. Vitamins C and E: Beneficial effects from a mechanistic perspective. Free Radic. Biol. Med. 2011. doi:10.1016/j. freeradbiomed.2011.05.017.
-
20.
Türkez H, Aydin E, Aslan A. Effects of Lichenic Extracts (Hypogymnia physodes, Ramalina polymorpha and Usnea florida) on Human Blood Cells: Cytogenetic and Biochemical Study. Iranian J. Pharm. Res. 2012;11:889-96.
-
21.
Padayatty SJ, Sun H, Wang Y, Riordan HD, Hewitt SM, Katz A, Wesley RA, Levine M. Vitamin C pharmacokinetics: implications for oral and intravenous use. Ann. Intern. Med. 2004;140:533-7. [PubMed ID: 15068981].
-
22.
Chen Q, Espey MG, Krishna MC, Mitchell JB, Corpe CP, Buettner GR, Shacter E, Levine M. Pharmacologic ascorbic acid concentrations selectively kill cancer cells: action as a pro-drug to deliver hydrogen peroxide to tissues. Proc. Natl. Acad. Sci. U. S. A. 2005;102:13604-9. [PubMed ID: 16157892].
-
23.
Farah IO, Lewis VL, Ayensu WK, Mahmud O. Assessing the survival of mrc-5 and a549 cell lines upon exposure to ascorbic Acid and sodium ascorbate. Biomed. Sci. Instrum. 2011;47:201-6. [PubMed ID: 21525621].
-
24.
Mahjan SS, Kamath VR, Ghatpande SS. Synergistic antimalarial activity of ketones with rufigallol and vitamin C. Parasitology. 2005;131:459-66. [PubMed ID: 16174410].
-
25.
Gould SW, Fielder MD, Kelly AF, El Sankary W, Naughton DP. Antimicrobial pomegranate rind extracts: enhancement by Cu(II) and vitamin C combinations against clinical isolates of Pseudomonas aeruginosa. Br. J. Biomed. Sci. 2009;66:129-32. [PubMed ID: 19839222].
-
26.
Ambadath V, Venu RG, Madambath I. Comparative study of the efficacy of ascorbic acid, quercetin, and thiamine for reversing ethanol-induced toxicity. J. Med. Food. 2010;13:1485-9. [PubMed ID: 20946019].
-
27.
Young VR, Newberne PM. Vitamins and cancer prevention: issues and dilemmas. Cancer. 1981;Suppl 5:1226-40. [PubMed ID: 7237379].
-
28.
Kaya H, Koc A, Sogut S, Duru M, Yilmaz HR, Uz E, Durgut R. The protective effect of N-acetylcysteine against cyclosporine A-induced hepatotoxicity in rats. J. Appl. Toxicol. 2008;28:15-20. [PubMed ID: 17461432].
-
29.
Sathish P, Paramasivan V, Palani V, Sivanesan K. N-acetylcysteine attenuates dimethylnitrosamine induced oxidative stress in rats. Eur. J. Pharmacol. 2011;654:181-6. [PubMed ID: 21114986].
-
30.
Bebarta VS, Kao L, Froberg B, Clark RF, Lavonas E, Qi M, Delgado J, McDonagh J, Arnold T, Odujebe O, O›Malley G, Lares C, Aguilera E, Dart R, Heard K, Stanford C, Kokko J, Bogdan G, Mendoza C, Mlynarchek S, Rhyee S, Hoppe J, Haur W, Tan HH, Tran NN, Varney S, Zosel A, Buchanan J, Al- Helial M. A multicenter comparison of the safety of oral versus intravenous acetylcysteine for treatment of acetaminophen overdose. Clin. Toxicol. (Phila). 2010;48:424-30. [PubMed ID: 20524832].
-
31.
Klein-Schwartz W, Doyon S. Intravenous acetylcysteine for the treatment of acetaminophen overdose. Expert Opin. Pharmacother. 2011;12:119-30. [PubMed ID: 21126198].
-
32.
Attri S, Rana SV, vaiphei K, sodhi CP, katyal R, Goel RC, Nain CK, Singh K. Isoniazid- and rifampicin-induced oxidative hepatic injury-protection by N-acetylcysteine. Hum. Exp. Toxicol. 2000;19:517-22. [PubMed ID: 11204554].
-
33.
Liang Q, Sheng Y, Ji L, Min Y, Xia Y, Wang Z. Acetaminophen-induced cytotoxicity on human normal liver L-02 cells and the protection of antioxidants. Toxicol. Mech. Methods. 2010;20:273-8. [PubMed ID: 20465405].
-
34.
Daraei B, Pourahmad J, Hamidi-Pour N, Hosseini MJ, Shaki F, Soleimani M. Uranyl acetate induces oxidative stress and mitochondrial membrane potential collapse in the human dermal fibroblast primary cells. Iranian J. Pharm. Res. 2012;11:495-501.
-
35.
Baniasadi S, Eftekhari P, Tabarsi P, Fahimi F, Raoufy MR, Masjedi MR, Velayati AA. Protective effect of N-acetylcysteine on antituberculosis drug-induced hepatotoxicity. Eur. J. Gastroenterol. Hepatol. 2010;22:1235-8. [PubMed ID: 20461008].
-
36.
Parry MF. Neu HC Effect of N-acetylcysteine on antibiotic activity and bacterial growth in-vitro. J. Clin. Microbiol. 1977;5:58-61. [PubMed ID: 401831].