Abstract
Keywords
S-layer protein Lactobacillus acidophilus ATCC4356 Stability Gel electrophoresis Circular dichroism
Introduction
One of the most commonly observed surface structures on the prokaryotic cell envelopes is monomolecular crystalline array of proteinaceous subunits with oblique, square and hexagonal structures termed Surface Layers or S-layers. S-layer monomers can be detached from underlying cell wall polymer and from each other using high concentration of Urea, Guanidine hydrochloride and Lithium chloride (1-5).
S-layer proteins from Lactobacilli have some similarities with other S-layer proteins in terms of the high percentage of hydroxylated and hydrophobic amino acid residues and the low content of sulfur containing amino acids. One of the specific characteristics of S-layer proteins of lactobacilli is the high content of positively charged residues which makes them highly basic protein with isoelectric point ranging from 9.4 to 10.4 and their small molecular mass ranging from 25 KDa to 71 KDa, which is very different from the rest of S-layer proteins, more acidic and with molecular masses up to 200kDa. S-layer protein of Lactobacillus acidophilus ATCC4356 is composed of a single, non-glycosylated protein of around 45 KDa (6-8).
Having small size and being resistant against harsh conditions (low pH, high temperature and pressure and enzymes), S-layer protein of Lactobacillus acidophilus ATCC4356 is an ideal candidate for serving as a protective coat for oral drug delivery carriers. In this sense, it is essential to evaluate its stability in gastrointestinal (GI) conditions.
Sodium dodecyl sulfate poly acryl amide gel electrophoresis (SDS- PAGE) has been used as an important tool for investigating the in-vitro stability of different proteins in simulated gastrointestinal conditions (9-16). Circular dichroism (CD) spectra have been used to analyze acid, heat, enzymes and chemical-induced unfolding (17-20).
In the present work, the S-layer protein of Lactobacillus acidophilus ATCC 4356 was extracted with three different chemical treatments and the extraction yields were compared on the basis of statistic tests. SDS-PAGE was used for the primary analysis of S-layer protein stability in the simulated gastrointestinal fluids (SGIF).
The unfolding process of the S-layer protein incubated in SGIF analyzed via CD provided a measure of secondary structural stability in SGIF.
Experimental
Bacterial strain and growth condition
Lactobacillus acidophilus ATCC 4356 and Lactobacillus casei ATCC393 (as a negative control for isolation of S-layer protein) were obtained from the Persian type culture collection. For the purpose of cultivation, the lyophilized bacteria were reactivated in MRS broth (Merck, Germany), with pH = 6.5 at 37°C overnight. The fresh activated Lactobacillus acidophilus ATCC4356 was used for the extraction of S-layer protein.
Extraction of the S-layer proteins
Extraction of S-layer protein with 4M GHCL
For extraction of Lactobacillus acidophilus ATCC4356 S-layer protein, the bacteria were harvested at the end of log phase (with optical density of 0.7 at 695 nm) by centrifugation LK 90 Optima (Beckman Coulter, USA) (at 15000 ×g for 15 min at 4°C) and washed twice with chilled distilled water. The end log harvested cell pellets were treated with 4M GHCL in 50mM Tris-HCl buffer (pH = 7.2), (1 g of harvested cell pellets was suspended in 10-15 mL of 4M GHCl) for 1 h at 37°C. The extracted S-layer protein was separated from the cell pellets by centrifugation (18000 ×g, 15 min, 4°C). The supernatant containing the S-layer protein was dialyzed over night at 4°C against two liters of 50mM Tris- buffer (pH = 7.2) with three times exchange of medium to remove residual GHCl. The extracted S-layer protein was stored at 4°C in 0.02% Na3N for further use (21, 22).
Extraction of S-layer protein with 1, 5 M LiCl
Ten to fifteen mg of the end log phase harvested cell pellets were suspended per one milliliter of 1, 5 M LiCl separately, kept at 0°C for 15 min, followed by centrifugation (30000×g, 15 min, 4°C). Both LiCl extracts were dialyzed against two liters of distilled water over night at 4°C with three times exchange of medium to remove residual LiCl. The exracted S-layer protein was stored at 4°C in 0.02% Na3N for further use (23-25).
Extraction of S-layer protein with 8M Urea (Mechanical extraction)
The washed harvested cell bacteria at the end of log phase were resuspended 1:1 in a buffer solution of 50mMTris-HCl and 3mM Na3N, pH = 7.2 (standard buffer) . In order to avoid DNA and RNA contamination in the S-protein extraction steps, a few crystals of DNase II and RNase were added. The cells were broken using a high shear fluid processor (M-110S Micro fluidizer processor, Newton, MA) at 4°C, a pressure of 960 bar, and ten passes, which resulted in a complete disintegration. After washing the cell wall fragments two times in standard buffer, the plasma membranes were solubilized in 1%Triton X-100 in standard buffer for 10 min at room temperature. The remaining cell wall fragments were washed twice. Peptidoglycan was lysed by incubating the sample in a standard buffer containing 0.2 mg/mL lysozyme for 6 h at 30°C. The S-layer protein suspension was mixed with 8 M Urea in 50 mM Tris-HCl buffer and pH of 7.4, until the solution became clear. After stirring the solution for 2 h at room temperature, non-protein components were precipitated through centrifugation (12400×g, 60 min and 4°C). The supernatant was dialyzed against two liters 10 mM CaCl2, 3 mM Na3N for 24hr at 4°C. The extracted S-layer protein was stored for further use (26, 27).
Protein assay
Surface layer protein concentrations, extracted under different extraction conditions, were determined by Bradford protein assay protocol using bovine serum albumin as a standard (28).
Sodium dodecyl sulfate poly acryl amide gel electrophoresis (SDS-PAGE):
Gel electrophoresis of S-layer proteins was performed using 12% (w/v) resolving gel and 4% stacking gel as described previously by Loral et al. (29). Molecular mass standard proteins were obtained from Sigma and Cinnagen Company. The gels were run for about 240 min at 150 V, washed with distilled water, stained with Coomassie blue G250 and destained by washing it several times with distilled water.
UV spectrophotometry
In order to find the maximum absorbance of the extracted S-layer protein with three different methods, the diluted protein samples were analyzed on RAY LEIGH UV 2601 spectrophotometer between λ of 220 nm and λ of 400 nm. The data were collected using the UV software. The experiments were done triplicate (n = 3).
Gel electrophoresis evaluation of in-vitro stability of the extracted S-layer protein in simulated gastrointestinal fluid (SGIF)
Considering the stability of S-layer proteins in harsh conditions such as pH, temperature, proteolysis of some kind and high pressures in one side and suitable small size of Lactobacillus surface layers as a protection coat in oral delivery on the other side, it would be necessary to determine S-layer digestion stability facing harsh conditions of gastrointestinal tract. The analytical tool generally used to track the digestion of substrate protein in simulated gastro intestinal fluid is sodium dodecyl sulfate poly acryl amide gel electrophoresis (SDS-PAGE).
Simulated gastrointestinal fluids were prepared as described in the United States pharmacopeia (USP2000) (30). The simulated gastric fluid (SGF) consisted of 2 g/l of sodium chloride (Merck, Germany), containing 3 g/l of pepsin from porcine stomach mucosa, 800- 2500 units/mg, (Sigma-Aldrich, Germany) with pH adjusted to 2 and 3.2 with 37% HCl (30).
The simulated intestinal fluid (SIF) consisted of 6.8 g/L of phosphate potassium monobasic. The pH was adjusted to 6.8 using 0.2 N sodium hydroxide or 0.2N HCl (30).
Fasted state simulated intestinal fluid (FaSSIF) consisted of 3.9 g/L phosphate potassium monobasic, 2.3 g/L sodium taurocholate, 0.56g/l lecithin and 1.1 g/L potassium chloride. The related pH level was adjusted to 6.5 using 0.2N sodium hydroxide (31).
Fed state simulated intestinal fluid (FeSSIF) consisted of 8.24 mL/L acetic acid, 10.2 g potassium chloride, 11.5 g/L sodium taurocholate and 2.8g/L lecithin. The pH level was adjusted to 5 using 0.2 N sodium hydroxide (31).
In-vitro stability assay of S-layer protein in SGF
In order to investigate the stability of surface layer protein of Lactobacillus acidophilus ATCC 4356 in gastric conditions, three types of SGF were prepared including SGF adjusted to pH = 2 without pepsin and SGF adjusted to pH = 3.2 with and without pepsin.
SGF (1900 μL) was incubated at 37°C for 5 min before addition of 100 μL of S-layer protein (5 mg/mL) at time zero. An aliquot (100 μL) of the digest was withdrawn at different time intervals (0, 5, 15, 30, 60, 90 and 120 min) and was immediately terminated by addition of 30μl of 200mM Na2Co3 and 25 μL of sample buffer. The samples were heated at 100°C for 10 min and analyzed by SDS-PAGE.
In-vitro stability assay of S-layer protein in SIF
SIF, FaSSIF and FeSSIF were prepared as previously described. The amount of S-layer protein and the volume of media for protein incubation are the same as that of previous section. An aliquot (100 μL) of the digests was withdrawn at 0 and 240 min of incubation in the mentioned media and added to a separate sampling tube containing 25 μL sample buffer. The tubes were boiled at 100°C for 10 min and the contents were subjected to SDS-PAGE gel.
Evaluation of the secondary structural changes of the S-layer protein incubated in SGIF by Circular Dichroism (CD)
Circular Dichroism (CD) is a valuable spectroscopic technique for studying the changes of protein structure in solution because many common conformational motifs, including α-helixes, β-sheets and turns which possess their own distinctive characteristics in Far-UV (200 -250 nm) CD spectra (17-20).
In order to investigate the secondary structural changes of S-layer protein of Lactobacillus acidophilus ATCC4356 under various experimental conditions, CD measurements were performed using Jasco- 815 Spectropolarimeter (Jasco Corporation, Tokyo, Japan) at room temperature. Far- UV spectra (200-250 nm) were recorded in a 1 mm -path- length cell, with scan speed of 50 nm/min in a continuous mode. Bandwidth of 2 nm was used. The S-layer protein concentration of 0.25 mg/mL was prepared in different simulated media and conditions. The appropriate buffer spectrum was subtracted from each protein absorbance spectrum and the data were normally plotted as mean residue weight ellipticity versus wavelength. For each sample, five scans were accumulated and averaged.
Results and Discussion
Comparison of different extraction S-layer protein methods
Whole cells of Lactobacillus acidophilus were treated in different incubation conditions to extract surface layer protein. The amount of protein isolated by each method was assayed by Bradford protein assay test with the results listed in Table 1.
Extraction of Lactobacillus acidophilus ATCC4356 S-layer protein from whole cell bacteria in different incubation conditions.
Incubation condition of L. acidophilus | S-layer protein extraction agent | S-layer protein extracted (mg/mL) (mean ± SEM*, n = 3) | % CV |
---|---|---|---|
MRS broth (pH = 6.5) , 37°C in anaerobic jar | GHCl (4M) | 0.773 ± 0.012 | 2.75 |
MRS broth (pH = 5) , 37°C in anaerobic jar | GHCl (4M) | 0.816 ± 0.011 | 2.47 |
MRS broth (pH = 6.5) , 37°C in Co2 incubator | GHCl (4M) | 0.512 ± 0.006 | 2.19 |
MRS broth (pH = 5) , 37°C in Co2 incubator | GHCl (4M) | 0.607 ± 0.004 | 1.23 |
MRS broth (pH = 6.5) , 45°C in anaerobic jar | GHCl (4M) | 0.984 ± 0.004 | 0.76 |
MRS broth (pH = 5) , 45°C in anaerobic jar | GHCl (4M) | 1.15 ± 0.032 | 4.93 |
MRS broth (pH = 6.5) , 45°C in CO2 incubator | GHCl (4M) | 0.835 ± 0.008 | 1.44 |
MRS broth (pH = 5) , 45°C in CO2 incubator | GHCl (4M) | 1.007 ± 0.001 | 2.49 |
MRS broth (pH = 6.5) , 37°C in anaerobic jar | Urea (8M) | 0.227 ± 0.006 | 5.13 |
MRS broth (pH = 5) , 45°C in anaerobic jar | Urea (8M) | 0.354 ± 0.007 | 3.57 |
MRS broth (pH = 6.5) , 37°C in anaerobic jar | LiCl (1M) | 0.088 ± 0.004 | 8.64 |
MRS broth (pH = 5) , 45°C in anaerobic jar | LiCl (1M) | 0.111± 0.002 | 3.39 |
MRS broth (pH = 6.5) , 37°C in anaerobic jar | LiCl (5M) | 0.135 ±0.008 | 11.08 |
MRS broth (pH = 5) , 45°C in anaerobic jar | LiCl (5M) | 0.182 ± 0.004 | 4.11 |
It is possible to extract surface layer protein from whole cell of Lactobacillus acidophilus ATCC4356 at a remarkably high concentration with 4M Guanidine hydrochloride compared with other extraction methods. All the extraction procedures were repeated three times. Statistical analysis was performed using SPSS statistics 19 and Excel 2010 software. The amounts of S-layer protein isolated through different methods were compared against each other using Student t-test and one-way ANOVA. The value of p < 0.05 was regarded as statistically significant. According to statistical analysis, the amounts of S-layer protein extracted using three extraction methods were significantly different (p-value = 0.000). The amount of S-layer protein extraction significantly increased by incubation of bacteria in acidic medium at 45°C in anaerobic condition (p-value = 0.001).
These findings are in line with a study conducted by Khaleghi et al. which investigated the effect of environmental stress on S-layer production in Lactobacillus acidophilus ATCC4356 based on slpA gene expression (slpA is the active S-layer protein encoding gene in normal growth condition) (21). They found that environmental conditions influenced the S-layer protein production and slpA gene expression. They showed that S-layer proteins are preferentially expressed in bacteria incubated in MRS broth medium (pH = 5) at 45°C in anaerobic condition.
UV spectrophotometry
Maximum absorbance for all Lactobacillus acidophilus ATCC4356 surface layer proteins extracted via different methods was found to be 280nm (λ max = 280 nm). The maximum absorbance is related to GHCl extracted protein (Figure 1). It is in line with the studies on the UV absorbance of LiCl extracted S-layer protein of Lactobacillus acidophilus NCC2628 fermented in various media, and maximum UV absorbance of Bacillus sphaericus S-layer protein extracted with GHCl (6M) (25, 27).
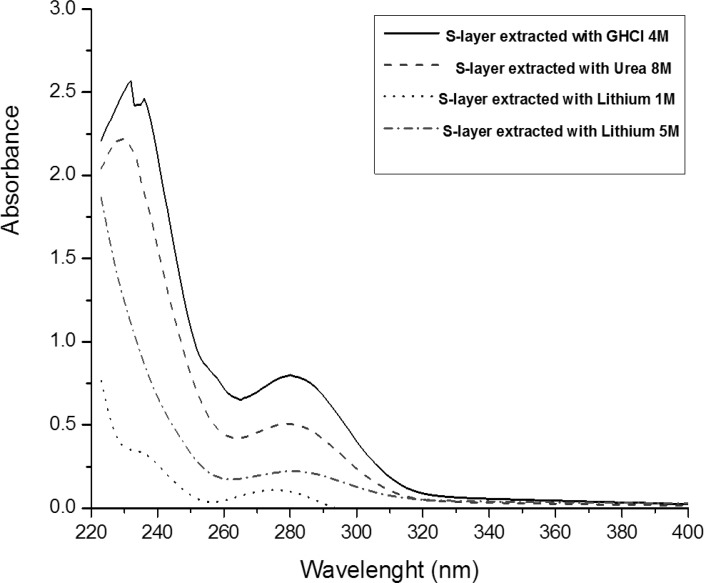
In-vitro stability of S-layer protein in simulated gastrointestinal fluids studied with SDS-PAGE
The present study is the first of its type in-vitro S-layer protein stability pattern using gel electrophoresis and circular dichroism. The standardization of the simulating gastrointestinal conditions has been described in the U.S Pharmacopeia. It is not meant to mimic precisely the fate of proteins in-vivo conditions but rather to evaluate the susceptibility of the protein to digest under fixed conditions in-vitro.
The S-layer protein extracted via different methods revealed a dominant band corresponding to the molecular mass of 43-46 KD (Figure 2). In the first step, the stability of S-layer protein in simulated gastrointestinal fluids was analyzed by SDS-PAGE. It is worth noting that this technique had been formerly used for evaluating the in-vitro gastrointestinal stability of different edible proteins by other researchers (10-17).
In the SGF reaction system (pH = 2, without pepsin), the S-layer protein band completely disappeared after 5 min without formation of any digestion fragments (Figure 2a). The low pH of SGF induces the protonation of carboxylic and amine groups in the hydrophilic shell and hence leads to a new distribution of charges and changes in the S-layer protein structure.
In the SGF reaction system (pH = 3.2), whether with or without pepsin, the original S-layer band persisted until the end of the study (120 min) (Figure 2b, 2c), suggesting that SGF with pH = 3.2 and above it, and also pepsin are ineffective in the degradation of the S-layer protein.
This result is similar to former results found in acidic degradation of Bacillus sphaericus surface layer protein studied by AFM (32). Tocca Herrera et al. have shown that due to exposure of the acidic condition below pH = 3, the original S-layer lattice of Bacillus sphaericusis deformed and the typical structure disappeared (32).
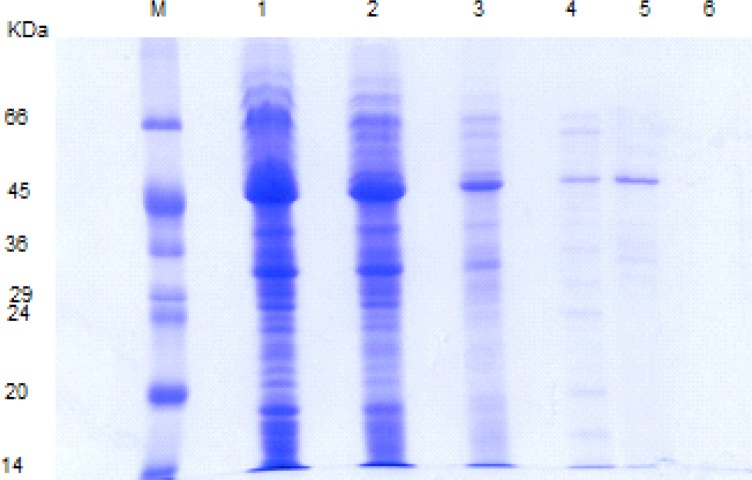
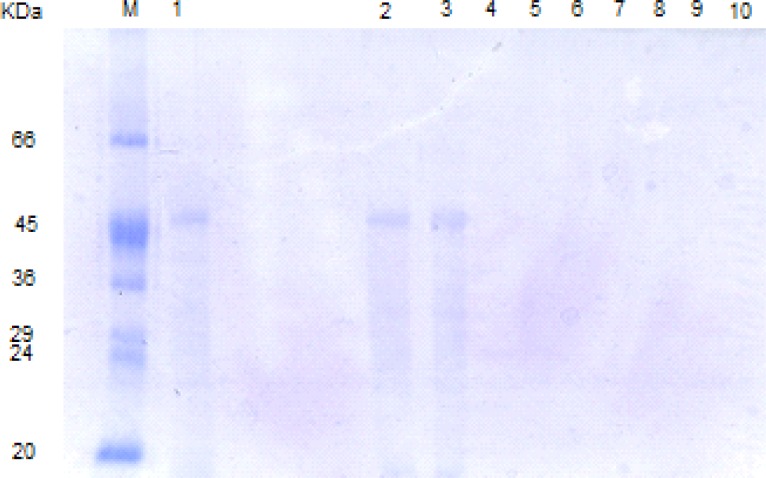
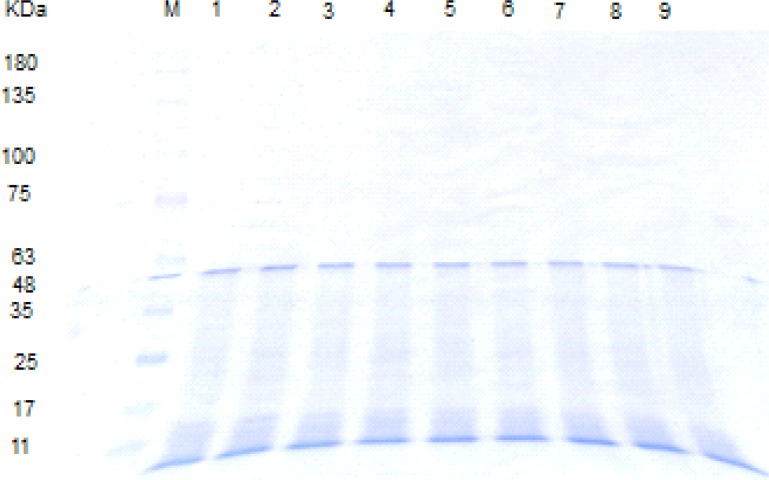
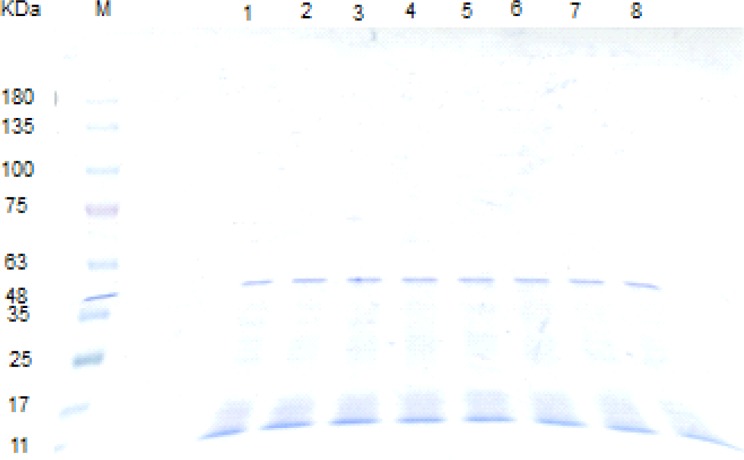
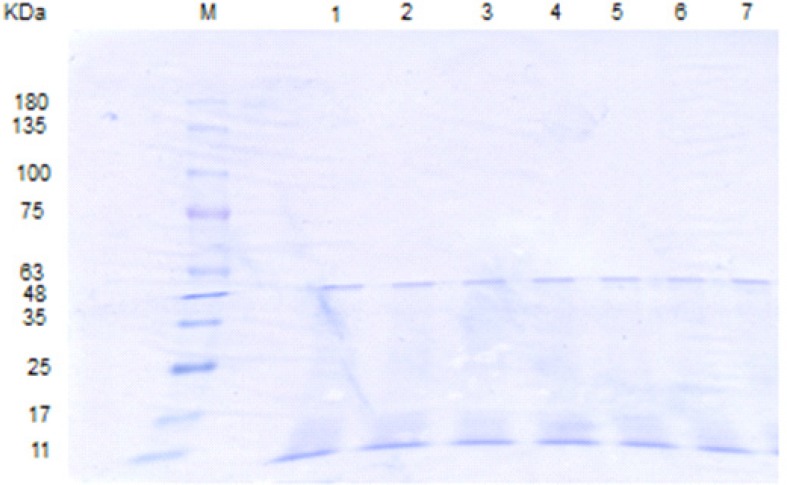
Previous researches concerning the SGF digestion of other edible proteins with approximately similar molecular weights have shown that most proteins are unstable in SGF with very acidic pH. Teshima et al. have studied the in-vitro gastrointestinal stability of bovine serum albumin (BSA), ovalbumin (OVA), horse radish protein (HRP), beta lactoglubin (BLG) with molecular weights of 63.1, 45.9, 48.8 and 17.7 KDa respectively. Based on it, in SGF (pH 2), BSA is rapidly digested before 1 min, while BLG remains indigested for 60 min. The original band of OVA rapidly decreases but a fragment remains until the end of the study (60 min). HRP is relatively stable in this medium (10).
In our study, the digestion patterns of S-layer protein in SIF, FaSSIF and FeSSIF were quite similar (Figure 2d). The S-layer protein was resistant in all of the above simulated intestinal fluids and the original band could be detected until the final time point (240 min). That is while Teshima et al. have found that BSA, OVA and HRP were resistant in SIF condition. In contrast, BLG was easily digested in SIF (10).
Secondary structural stability of S-layer protein in simulated gastrointestinal fluids studied with CD
The application of CD to the study of protein folding has been reviewed by Kelly and Prince (33). In order to see whether the conformational changes of S-layer protein occurred under different conditions, Far- UV CD spectra were taken at 25°C by using Jasco 815 spectropolarimeter. Alterations in the secondary structure are measured in the region of 200–250 nm, the so-called Far-UV CD. This region is dominated by contributions of the peptide bonds, although some side chains may also be involved. α- helices display large CD bands with negative ellipticity at 222 and 208 nm and positive ellipticity at 193 nm. β-sheets exhibit a broad negative band near 218 nm and a large positive band near 195 nm while disordered extended chains have a weak broad positive CD band near 217 nm and a large negative band near 200 nm. The spectrum of a protein is basically the sum of the spectra of its conformational elements, and thus CD can be used to estimate secondary structure.
The CD spectrum of fresh extracted Lactobacillus acidophilus ATCC4356 S-layer protein in Tris-HCl buffer in the Far- UV (200-250 nm) showed ellipticity with relatively large intensity between 210 and 230 nm, being characteristic of the α/ β conformation.
Fresh extracted Lactobacillus acidophilus ATCC 4356 S-layer contained 26% of alpha-helix, 5% of beta-helix, 33% of turn and 36% of random structures. The CD spectrum of S-layer protein incubated in SGF (pH = 2), up to 5 min, is similar to the CD spectrum of the fresh S-layer protein in Tris-HCl buffer (native spectra). It demonstrates that the S-protein retains its secondary structure in pH = 2 for 5 min. After that, the CD spectra significantly altered relative to that for the native spectra, gained content in β-helical structure (from 5% to 30%) and lost content in all other structures with the shifting of peak maxima to the shorter wavelengths in Far- UV (Figure 3).
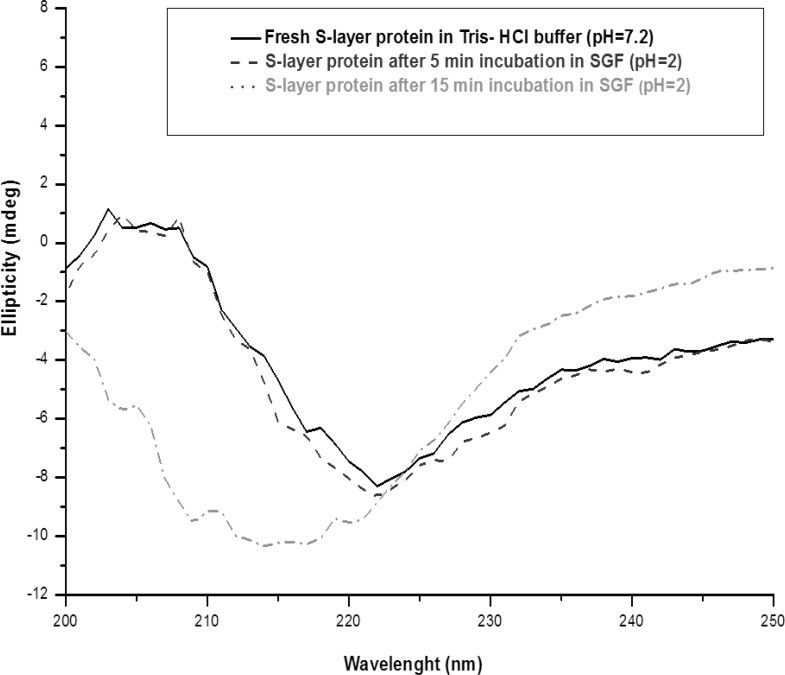
The changes of the secondary structure after 5 min observed with S-layer protein in SGF (pH = 2) can be explained by acid denaturation of the protein. The structure of S-layer protein is pH sensitive and the protein undergoes a structural transition to form a partially unfolded state. Under harsh acidic condition (pH = 2), all of the ionizable protein groups are protonated and the charge repulsion drives the changes of the secondary structure (18, 19, 32). The Far -UV CD spectra of S-layer protein incubated in SGF (pH = 3.2) is similar to the native spectra, which suggests that S-layer protein is stable in that medium (Figure 4).
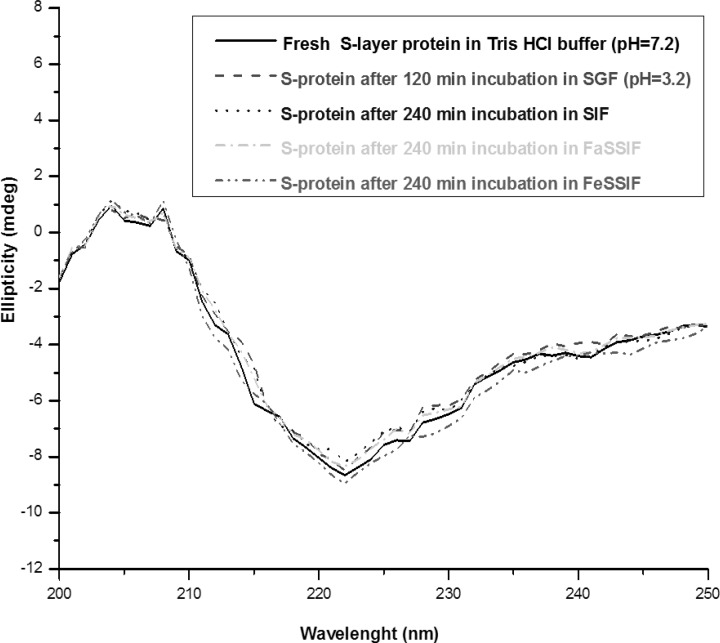
The Far- UV CD spectras of S-layer protein incubated in SIF, FaSSIF and FeSSIF are superimposable to the native CD spectra, implying that S-layer protein’s secondary structure is also stable in these simulated conditions (Figure 4).
Trust et al. investigated the secondary structure changes of S-layer protein of a pathogenic Aeromonas hydrophila strain (Mw = 52) under various experimental conditions. The CD spectra of this S-layer protein was incubated in pH = 2 and pH = 7.4, showing that no major conformational changes took place at low pH, implying that the protein was not drastically altered by the low pH (35). In contrast to the instability of Lactobacillus acidophilus ATCC 4356 S-layer protein in low pH, the S-layer of pathogenic Aeromonas hydrophila strain is stable in this condition.
Alcocer et al. measured the digestibility of Sunflower albumin and Brazil nut 2S albumin in simulated gastrointestinal fluids by SDS-PAGE and CD. For each protein, spectra obtained at pH = 2.2, were superimposable to those recorded at 6.8, confirming that these proteins are highly resistant to acidic pH (36).
Conclusion
S-layers are crystalline monomolecular assemblies of protein or glycoprotein, which represent one of the most common cell surface structures in Bacteria. Using the S-layer proteins as a protective coat in drug delivery and drug targeting, it is necessary to evaluate their in-vitro gastrointestinal stability to predict their behavior facing harsh conditions of gastrointestinal system. In this work, the in-vitro gastrointestinal stability of Lactobacillus acidophilus ATCC 4356 S-layer protein was studied by gel electrophoresis and circular dichroism.
The results confirmed that Lactobacillus acidophilus ATCC 4356 S-layer protein is readily digested under simulated gastric fluids (pH = 2) as demonstrated by both SDS-PAGE and CD spectra. It is also confirmed that the S-layer protein is stable in simulated gastric fluids (pH > 3) with and without pepsin and also stable in simulated intestinal fluids in both fasted and fed states. Our results showed that the S-layer protein of Lactobacillus acidophilus ATCC 4356 has the potential to be used as a protective coat for unstable oral drug delivery carriers.
Acknowledgements
References
-
1.
Sleytr UB, EgelseerE, Pum D, Schuster B. S-layers as a basic building block in a molecular construction kit. Federation of European Biochemical Societies Journal. 2007;274:323-334. [PubMed ID: 17181542].
-
2.
Mobili P, Gerbino E, Tymczyszyn E, Zavaglia A. Structural characteristics and putative role of S-layers in surface and probiotic properties of whole bacteria. Applied Microbiology and Microbial Biotechnology. 2010;51:1224-1234.
-
3.
Sara M, Sleytr UB. S-layer proteins. Journal of Bacteriology. 2000:859-868. [PubMed ID: 10648507].
-
4.
Sleytr UB, Sara M, Pum D, Schuster B. Characterization and use of crystalline bacterial cell surface layers. Progress in Surface Science. 2001;68:231-278.
-
5.
Sleytr UB, Egelseer E, Iik N, Pum D, Schuster B. S-layers as a basic building block in a molecular construction kit. Federation of European Biochemical Societies J. 2006;274:323-334.
-
6.
Jaskelainen S, Palva A. Lactobacillus Surface layers and their applications. Federation of European Microbiological Societies Review. 2005;29:511-529.
-
7.
Smit E, Oling F, Demel Rudy, Martinez B, Pouwels PH. The S-layer protein of Lactobacillus acidophilus ATCC4356: Identification and characterization of domains responsible for S-protein assembly and cell wall binding. J. Molecular Biology. 2001;305:245-257.
-
8.
Smit E, Jager D, Martinez B, Tielen F, Pouwels P. Structural and Functional Analysis of the S-layer protein Crystallization Domain of Lactobacillus acidophilus ATCC4356: Evidence for Protein- Protein Interaction of two sub domains. J. Molecular Biology. 2002;324:953-964.
-
9.
Yu H, Cao M, Cai Q, Liu GM. Effect of different processing methods on digestibility of Scylla paramamasain allergen (tropomyosin). Food and Chemical Toxicology. 2011;49:791-798. [PubMed ID: 21130825].
-
10.
Takagi K, Teshima R, Okunuki H, Sarada J. Comparative study of in-vitro digestibility of food proteins and effect of preheating on the digestion. Biological and Pharmaceutical Bulletin. 2003;26:969-973. [PubMed ID: 12843620].
-
11.
Haung Y, Liu G, Cai Q, WengW, Maleki S, Su W, Cao M. Stability of major allergen tropomyosin and other food proteins of mud crab by in-vitro gastrointestinal digestion. Food and Chemical Toxicology. 2010;48:1196-1201. [PubMed ID: 20156517].
-
12.
Yongchao G, Zhenxing L, Hong L. The effect of simulated gastrointestinal digestion on shrimp penaeusvannameiallergenicity. Chinese J. Oceanology and Limnology. 2009;27:703-707.
-
13.
Bannon G, Fu T, Kimber L, Hinton D. Protein digestibility and relevance to allergenicity. EnviromentalHealth Perspectives. 2003;111:1122-1124.
-
14.
Murtagh G, Archer DB, Dumoulin M, Arshad SH, Alcocer M. In-vitro stability and immunoreactivity of the native and recombinant food 2S albumins and SFA-8. Clinical and ExperimentalAllergy. 2003;33:1147-1152.
-
15.
Ubaidulla U, Khar RK, Ahmad F, Sultana Y, Panda A. Development and characterization of chitosan succinate microspheresfor the improved oral bioavailability of insulin. J. Pharmaceutical Sciences. 2007;96:3010-3023.
-
16.
Estey T, Kang J, Carpenter J. BSA degradation under acidic conditions:A model for protein instability during release from PLGA delivery systems. J. Pharmaceutical Sciences. 2006;95:1626-1639.
-
17.
Correa D, Ramos C. The use of circular dichroism spectroscopy to study protein folding, form and function. African J. Biochemistry Research. 2009;3:164-173.
-
18.
Mazzini A, Polverini E, Parisi M, Sorbi R, Favilla R. Dissociation and unfolding of bovine odorant binding protein at acidic pH. J.Structural Biology. 2007;159:82-91.
-
19.
Charalambous K, Bullough P, Walla BA. Thermal and chemical unfolding and refolding of eukaryotic sodium channel. BiochimicaetBiophysicaActa. 2009;1788:1279-1286.
-
20.
Griko Y, Sreerama N, Davis P, Woody R, Woody. Thermal and urea induced unfolding in T7 RNA polymerase: Calorimetry, Circular Dichroism and Florescence study. Protein Science. 2001;10:845-853. [PubMed ID: 11274475].
-
21.
Khaleghi M, Kermanshahi RK. Effect of environmental stresses on S-layer production in Lactobacillus acidophilus ATCC4356. Advances in Applied Biotechnology. 2012;21:1-17.
-
22.
Howorka S, Margit S, Werner L, Beatrix K. Self-assembly product formation of the Bacillus Stearothermopillus PV72/P6 S-layer protein SbsA in the course of autolysis of Bacillus Subtilis. Federation of European Microbiological Societies. 1999;172:187-196.
-
23.
Lortal S, Heijenoort J, Gruber K, Sleytr UB. S-layer of Lactobacillus helveticus ATCC 1204: Isolation, chemical characterization and reformation after extraction with Lithium Chloride. J. General Microbiology. 1992;138:611-618.
-
24.
Ventura M, Jankovic I, Walker DC, Pridmove R, Zink R. Identification of novel surface proteins in Lactobacillus Johnsonii and Lactobacillus gasseri. Applied and Enviromental Microbiology. 2002;68:6172-6181.
-
25.
Zammaretti P, Dillmann M, Amico N, Affolter M, Ubink J. Influence of fermentation medium composition on physicochemical surface properties of Lactobacillus acidophillus. Applied and Enviromental Microbiology. 2005;71:8165-8173.
-
26.
Iik N, Pum D, Sleytr UB, Palva A. Identification and characterization of domains responsible for self- assembly and cell wall binding of the surface layer protein of Lactobacillus brevis ATCC 8287. BMC Microbiology. 2008;8:1-15. [PubMed ID: 18173832].
-
27.
Fahmy K, Merroun M, Pollmann K, Raff J, Savchuk O, Henning C, Powell S. Secondary structure and Pd coordination in S-layer proteins from Bacillus sphaericusstudied by Infrared and X-ray absorption spectroscopy. Biophysical Journal. 2006;91:996-1007. [PubMed ID: 16698775].
-
28.
Bradford MM. A rapid and sensitive method for the quantitation of microgram quantities of protein utilizing the principle of protein-dye binding. Analytical Biochemistry. 1976;72:248-54. [PubMed ID: 942051].
-
29.
Laemmli UK, Favre M. Gel electrophoresis of proteins. Journal of Molecular Biology. 1973;80:575-599. [PubMed ID: 4204102].
-
30.
United States Pharmacopeia, National Formulary USP 24/ NF 19. 24. Rockville: The United States Pharmacopeial Convention; 2000. p. 2235-36.
-
31.
Charman WN, Porter C, Mithani S, Dressman JB. Physicochemical and physiological mechanism for the effects of food on drug absorption, the role of lipid and pH. J. pharmaceutical science. 1997;86:269-282.
-
32.
Toca- Herrera J, Moreno S, Friedmann J, Pum D. Chemical and thermal denaturation of crystalline bacterial S-layer proteins: An atomic force microscopy study. Microscopy research and technique. 2004;65:226-234. [PubMed ID: 15630688].
-
33.
Kelly S, Prince NC. The application of circular dichroism to study of protein folding and unfolding. BiochemicaetBiophysicaActa. 1997;1338:161-185.
-
34.
Swint L, Robertson AD. Thermodynamics of unfolding for turkey ovomucoid third domain: Thermal and chemical denaturation. Protein Science. 1993;2:2037-2049. [PubMed ID: 8298454].
-
35.
Dooley J, Cubbin W, Kay C, Trust T. Isolation and biochemical characterization of the S-layer protein from pathogenic Aeromonashydrophila strain. J. Bacteriology. 1998;170:2631-2638.
-
36.
Murtagh G, Archer DB, Dumoulin M, Alcocer MJ. In-vitro stability and immunoreactivityof the native and recombinant plant food 2S albumins,Ber e1 and SFA-8. J.Clinical and Experimental Allergy. 2003;33:1147-1152.