Abstract
Keywords
Introduction
Alzheimer disease was diagnosed for the first time in a German patient named Alois Alzheimer (1). It is one of the diseases affecting a large number of people all over the world; being the most common cause of dementia in the elderly (1, 2). The amyloid cascade hypothesis proposes that aggregation of amyloid beta 40 and 42 (Aβ40 and Aβ42) oligopeptides followed by generation of neurotoxic plaques in brain as commonly occurring features in AD (3). Proteolytic cleavage of a large trans-membrane protein, amyloid precursor protein (APP), by two enzymes namely β and γ-secretases results in secretion of Aβ40 and Aβ42 peptides (4,5). β-secretase (Beta-site APP cleaving enzyme or BACE-1) is a type I membrane-associated aspartyl protease (3), which has been an attractive therapeutic target in AD due to the fact that it catalyzes the first step in Aβ production and is mainly expressed in brain.
Therefore, development of specific inhibitors of these key proteases has been regarded as a major therapeutic target in AD treatment and many research groups have focused on development of beta-secretase inhibitors (6, 7). Nowadays, it is generally accepted that biologically potent compounds will not necessarily end in good drugs and that there are several critical parameters along the discovery process such as MW or partition coefficient responsible for the optimal pharmacological outputs (8). These simple property counting rules have been applied successfully to distinguish between drugs and non-drugs (9-11).
Experimental measurement of binding affinities for huge molecular libraries is a time-consuming and non-economic process being a major bottle-neck in the field of drug discovery. To overcome this limitation, virtual screening techniques have found their usefulness in finding potential bioactive compounds prior to synthesis. The concept of interpreting ligand-receptor interaction in terms of the free energy per atom (ligand efficiency, LE) was first proposed by P. Andrews (12). Consequently several efficiency indices have been proposed by other groups (13). These ligand-based efficiency indices are now regarded as undeniable part of modern lead development strategies (14, 15). In this regard, the use of structure-based calculated binding free energies (16) instead of experimental binding affinities may become successful alternative for obtaining LEs. Estimated LEs may offer negligible time and, consequently, reduce time-consuming and expensive biochemical measurements. In an AutoDock based study, BACE-1 inhibitors were used to correlate the estimated and experimental LEs. Results showed an acceptable correlation among the data (17).
Availability of a significant amount of crystallographic data on Protein Data Bank (PDB, http://www.rcsb.org) has facilitated the performance of structure based drug discovery projects aiming at beta-secretase as a molecular target for Alzheimer disease. Holo X-ray crystallographic structures which bear cognate ligands are principal sources for this purpose (18).
In the present work, the PDB database was screened for potential small molecule BACE-1 inhibiting molecules and 83 structures were extracted for our structure-based study. Dissection analysis was performed on two-dimensional structures of the selected compounds. This shape-based analysis resulted in fragments, graph-based frameworks and side chains. Obtained fragments were subjected to docking simulations into BACE-1 active site and results were further discussed for binding energies and binding efficiency indices.
Computational section
Chemical structures of ligands under study were all extracted from Holo PDB files documented in PDB database (83 PDB codes). Our criterion for selected molecules considered chemical structures bearing 10 or less rotatable bonds after dissection into simplified structures (19). Molecular descriptors were calculated by Dragon software version 2.1 (20).
Flexible-ligand docking studies were performed using AutoDock 4.2 program (21). All the pre-processing steps for ligand and receptor crystallographic files were performed within WHAT IF server (European Molecular Laboratory Heidelberg, Germany) and AutoDock Tools 1.5.4 program (ADT) which has been released as an extension suite to the Python Molecular Viewer (22, 23). All hydrogens were properly added to the receptor PDB file using What if server. ADT program was used to merge non-polar hydrogens into carbon atoms of the receptor and Kollman charges were also assigned. For docked ligands, non-polar hydrogens were also added. Gasteiger charges assigned and torsions degrees of freedom allocated by ADT program. Chemical fragments were docked into BACE-1 active site holding their initial conformation unchanged. The Lamarckian Genetic Algorithm (LGA) was applied to model the interaction pattern between BACE-1 and inhibitors. For all docking procedures, 150 independent genetic algorithm (GA) runs were considered for each molecule under study. A maximum number of 5×106 energy evaluations; 27000 maximum generations; a gene mutation rate of 0.02 and a crossover rate of 0.8 were used for Lamarckian genetic algorithm. The grid maps representing BACE-1 in the docking process were calculated using AutoGrid (part of the AutoDock package) with a grid spacing of 0.375 Å (roughly a quarter of the length of a carbon–carbon single bond). A grid of 60, 60, and 60 points in x, y, and z directions was centered on the center of mass of the active site of BACE-1 since the locations of the original ligands (cognate ligands) in the complex were known. Cluster analysis was performed on the docked results using a root mean square (RMS) tolerance of 2 Å. Schematic 2D representations of the ligand-receptor binding maps were generated using LIGPLOT (24).
Results and Discussion
Dissection analysis of BACE-1 inhibitors
In order to better recognize the structural features of BACE1 inhibitors, we used a fragmentation method on the compounds originated from PDB database. BACE1 inhibitors were classified into several constructive units. Chemical structures and their fragments are depicted in a hierarchy model in Figure 1. The advantages of such dissection methods have been well established in previous reports (25).
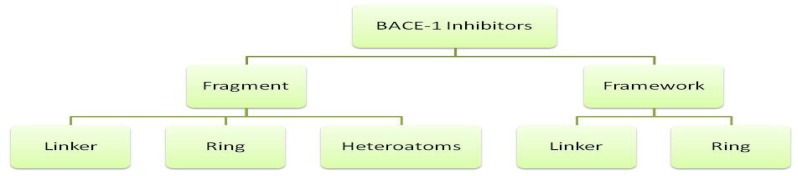
Frameworks are molecular descriptions on the basis of graph theory in which atoms and bonds are depicted as vertexes and edges of a graph ignoring atom types, atomic hybridizations, atomic charges and bond orders (26). In scaffolds, molecular properties such as atom type, atom hybridization and bond order are incorporated. Side chains are non-ring, non-linker atoms. Linker atoms are situated at the pathway connecting ring systems. One distinguished feature in our present study is that we considered linkers without any elimination of functional groups such as carbonyl moieties or other side branches. In this way, detailed structural features of linker groups maybe provided. PDB codes of BACE-1 inhibitors and related fragments are summarized in Table 1.
Calculated descriptors for BACE-1 inhibitors (PDB codes) and their fragments
PDB code | HACa | HDsb | HAsc | MW |
---|---|---|---|---|
ligand / fragment | ligand / fragment | ligand / fragment | ligand / fragment | |
1TQF | 40 / 28 | 4 / 1 | 9 / 6 | 571.7 / 395.1 |
1W51 | 39 / 28 | 3 / 3 | 7 / 4 | 531.7 / 374.2 |
2EWY | 38 / 37 | 3 / 3 | 5 / 5 | 504.6 / 490.2 |
2F3F | 34 / 19 | 5 / 3 | 8 / 3 | 514.7 / 297.1 |
2IQG | 41 / 28 | 3 / 3 | 6 / 6 | 677.6 / 374.2 |
2OF0 | 19 / 17 | 1 / 1 | 4 / 4 | 265.3 / 237.1 |
2OHL | 13 / 10 | 2 / 0 | 2 / 1 | 144.2 / 129.1 |
2OHK | 13 / 10 | 2 / 0 | 2 / 1 | 144.2 / 129.1 |
2OHM | 15 / 14 | 3 / 1 | 3 / 2 | 199.3 / 184.1 |
2OHN | 15 / 13 | 1 / 1 | 1 / 1 | 193.3 / 175.1 |
2OHP | 21 / 17 | 3 / 1 | 3 / 2 | 237.3 / 222.1 |
2OHQ | 23 / 20 | 2 / 0 | 3 / 1 | 304.4 / 259.1 |
2OHR | 23 / 20 | 3 / 1 | 4 / 3 | 276.3 / 261.1 |
2OHS | 24 / 20 | 3 / 1 | 5 / 3 | 306.4 / 261.1 |
2OHT | 24 / 23 | 4 / 2 | 4 / 3 | 314.4 / 299.1 |
2OHU | 32 / 31 | 4 / 2 | 6 / 5 | 421.5 / 406.2 |
2P83 | 44 / 28 | 5 / 3 | 10 / 4 | 610.7 / 374.2 |
2Q11 | 31 / 30 | 2 / 0 | 6/ 5 | 288.4 / 403.2 |
2Q15 | 37 / 36 | 2 / 0 | 6 / 5 | 496.6 / 487.3 |
2QK5 | 42 / 28 | 3 / 3 | 7 / 4 | 581.7 / 374.2 |
2QMD | 44 / 32 | 3 / 3 | 7 / 5 | 607.7 / 430.2 |
2QMF | 43 / 31 | 3 / 3 | 7 / 5 | 593.7 / 416.2 |
2QP8 | 40 / 25 | 3 / 3 | 7 / 4 | 559.7 / 338.2 |
2QU2 | 24 / 17 | 4 / 1 | 5 / 1 | 318.4 / 219.1 |
2QU3 | 29 / 17 | 4 / 0 | 5 / 0 | 427.9 / 236.1 |
2VA5 | 19 / 17 | 4 / 2 | 5 / 3 | 254.3 / 223.1 |
2VA6 | 24 / 18 | 2 / 1 | 5 / 2 | 323.4 / 234.1 |
2VA7 | 26 / 18 | 2 / 1 | 5 / 2 | 341.4 / 234.1 |
2WF4 | 40 / 35 | 2 / 4 | 9 / 7 | 554.7 / 492.2 |
2WJO | 35 / 34 | 3 / 1 | 4 / 5 | 473.6 / 458.2 |
2ZDZ | 35 / 24 | 4 / 1 | 7 / 2 | 486.9 / 311.1 |
2ZE1 | 34 / 26 | 5 / 2 | 7 / 3 | 516.4 / 338.1 |
2ZJH | 20 / 13 | 1 / 0 | 3 / 1 | 292.4 / 175.1 |
2ZJI | 24 / 13 | 1 / 0 | 5 / 1 | 352.5 / 175.1 |
2ZJJ | 20 / 13 | 2 / 1 | 4 / 2 | 297.4 / 176.1 |
2ZJK | 21 / 13 | 2 / 1 | 4 / 2 | 311.4 / 176.1 |
2ZJL | 15 / 13 | 1 / 0 | 5 / 1 | 431.4 / 175.1 |
2ZJM | 35 / 24 | 3 / 1 | 9 / 4 | 526.1 / 324.1 |
2ZJN | 36 / 24 | 3 / 1 | 9 / 4 | 540.1 / 324.1 |
3BRA | 10 / 6 | 3 / 0 | 2 / 0 | 137.2 / 78.1 |
3BUF | 11 / 6 | 3 / 0 | 2 / 0 | 151.2 / 78.1 |
3BUG | 12 / 6 | 3 / 0 | 2 / 0 | 165.2 / 78.1 |
3BUH | 16 / 12 | 3 / 0 | 2 / 0 | 219.3 / 160.1 |
3CIB | 44 / 32 | 3 / 3 | 6 / 4 | 605.8 / 428.2 |
3CIC | 45 / 32 | 3 / 3 | 8 / 5 | 620.7 / 427.2 |
3CID | 44 / 31 | 3 / 3 | 8 / 5 | 606.7 / 413.2 |
3DV5 | 35 / 27 | 3 / 4 | 6 / 4 | 487.7 / 372.2 |
3EXO | 25 / 19 | 2 / 1 | 6 / 3 | 340.4 / 247.1 |
3FKT | 36 / 30 | 2 / 1 | 7 / 4 | 491.6 / 400.2 |
3H0B | 28 / 19 | 3 / 1 | 8 / 2 | 386.4 / 248.1 |
3HVG | 11 / 6 | 3 / 1 | 4 / 2 | 153.2 / 80.1 |
3HW1 | 16 / 15 | 2 / 0 | 4 / 3 | 214.3 / 199.1 |
3IGB | 22 / 19 | 2 / 0 | 4 / 3 | 418.5 / 275.1 |
3IN3 | 26 / 23 | 2 / 1 | 6 / 4 | 343.4 / 298.1 |
3IN4 | 30 / 23 | 2 / 1 | 7 / 5 | 400.5 / 299.1 |
3IND | 24 / 21 | 2 / 1 | 4 / 2 | 323.5 / 278.2 |
3INE | 27 / 21 | 2 / 1 | 5 / 2 | 367.5 / 278.2 |
3INF | 29 / 23 | 2 / 1 | 6 / 3 | 386.4 / 297.1 |
3INH | 32 / 23 | 2 / 1 | 7 / 4 | 445.4 / 298.1 |
3IVH | 34 / 28 | 3 / 3 | 4 / 4 | 472.6 / 380.2 |
3IVI | 37 / 31 | 4 / 4 | 6 / 6 | 510.6 / 418.2 |
3KMX | 17 / 6 | 3 / 0 | 3 / 0 | 272.8 / 78.1 |
3KMY | 16 / 14 | 2 / 0 | 2 / 1 | 232.7 / 183.1 |
3KN0 | 24 / 23 | 2 / 0 | 4 / 3 | 321.4 / 306.2 |
3L3A | 32 / 24 | 2 / 0 | 5 / 2 | 442.9 / 310.2 |
3L5B | 20 / 12 | 2 / 1 | 4 / 2 | 293.8 / 158.1 |
3L5C | 32 / 23 | 4 / 3 | 8 / 5 | 432.3 / 306.1 |
3L5D | 28 / 12 | 4 / 1 | 7 / 2 | 387.5 / 158.1 |
3L5E | 39 / 33 | 3 / 2 | 7 / 4 | 535.8 / 446.3 |
3L5F | 23 / 20 | 2 / 2 | 4 / 2 | 319.5 / 274.2 |
3L38 | 33 / 31 | 2 / 0 | 6 / 5 | 453.9 / 404.1 |
3L58 | 42 / 28 | 3 / 3 | 7 / 4 | 581.7 / 374.2 |
3L59 | 17 / 12 | 2 / 1 | 4 / 2 | 251.7 / 158.1 |
3LHG | 28 / 23 | 2 / 1 | 5 / 3 | 378.4 / 297.1 |
3LNK | 45 / 33 | 3 / 3 | 7 / 6 | 620.7 / 443.2 |
3LPI | 46 / 34 | 3 / 3 | 7 / 7 | 656.8 / 479.2 |
3LPJ | 44 / 32 | 3 / 3 | 7 / 6 | 606.7 / 429.2 |
3MSJ | 15 / 9 | 3 / 1 | 4 / 2 | 225.7 / 118.1 |
3MSK | 24 / 22 | 2 / 0 | 5 / 4 | 348.9 / 299.2 |
3MSL | 25 / 23 | 3 / 1 | 5 / 4 | 347.9 / 313.2 |
3MSM | 28 / 26 | 3 / 1 | 5 / 4 | 400.9 / 351.2 |
3PI5 | 29 / 24 | 3 / 1 | 5 / 1 | 480.4 / 337.2 |
3QBH | 38 / 27 | 3 / 2 | 8 / 3 | 544.7 / 380.2 |
It was crucial to retain the atomic hybridizations within simplified structures when side chains were removed, therefore, dot pairs were considered next to the sp2 atoms to designate the related π electrons (25). No acyclic molecule was found and all structures under study possessed at least one ring system. The thing which is worth noting is the definition of ring and cycle. The cycles alone or fused to other cycles represent a unit ring system within each molecule. Graph-based frameworks and simplified structures of small molecule BACE1 inhibitors are shown in Figures 2 and 3.
As it would be expected, classification scheme based on frameworks represented less diversity compared to the molecular scaffolds, because atom types, hybridizations and bond orders were not incorporated. Most of the evaluated BACE-1 inhibitors could be represented with 13 independent frameworks (Figure 2).
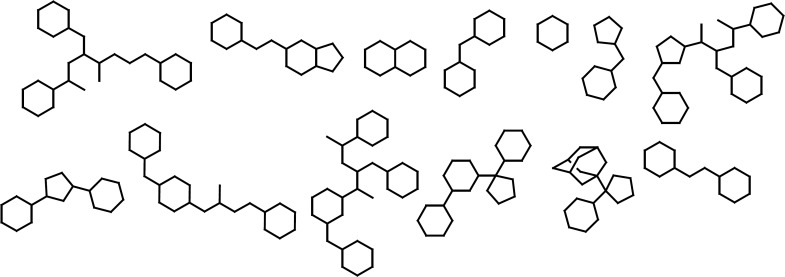
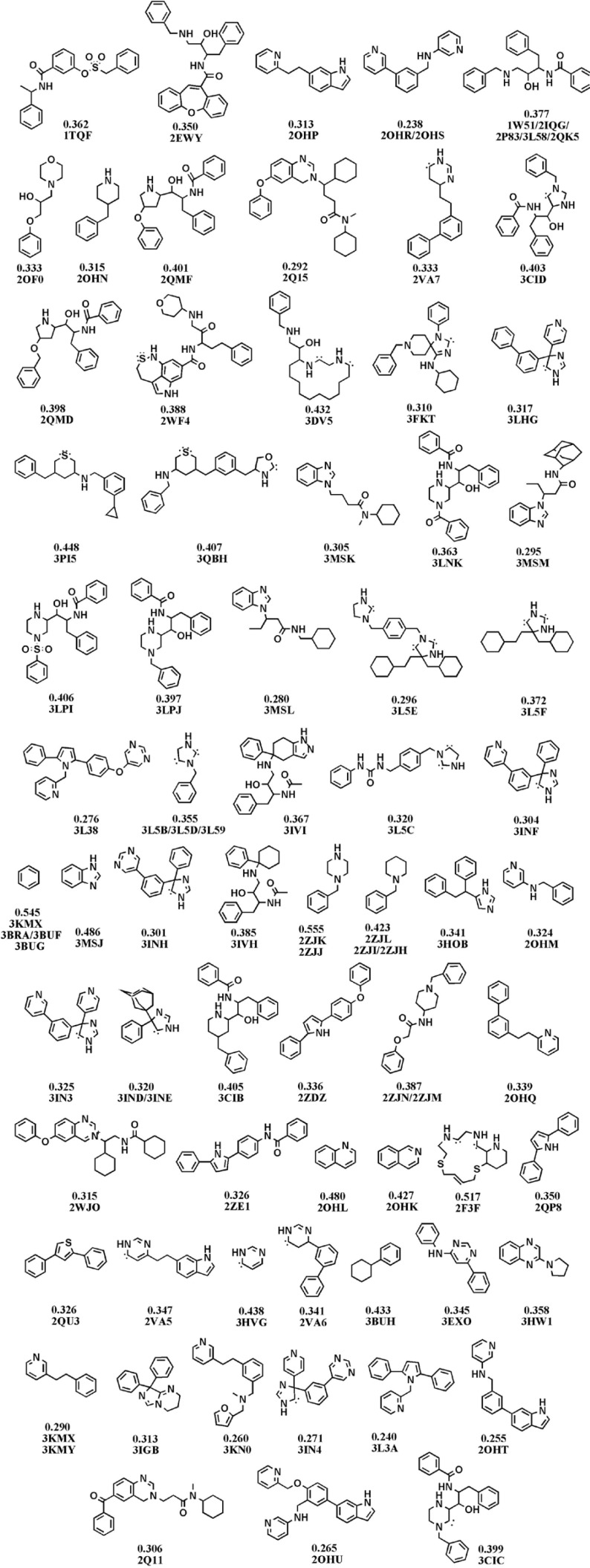
Ligand efficiency indices
Ligand efficiency index can be simply calculated using the Equation 1 (12):
(1)
HAC stands for heavy atom count. Concept ‘efficiency’ of a ligand could be a useful parameter in considering the real potency of a compound and hence optimizing fragments (27). Molecules that exhibit a distinct potency with fewer heavy atoms are by definition more efficient (28). Accordingly, obtained simplified chemical structures were re-ranked depending on their ligand efficiency values to be evaluated more sensibly. Regarding the ligand efficiencies, C.A. Zapatero et al. postulated that molecular weights are prior to the number of non-hydrogen atoms in considering the contribution of heteroatoms from different rows of the periodic table (13). These authors suggested a modified efficiency value entitled “binding efficiency index” (BEI). This index could be easily estimated from Equation 2:
(Equation 2)
The importance of BEI can be emphasized as an increase in molecular weight at the clinical candidate step, which is regarded as an undeniable paradox with a common trend towards lower MWs and better pharmacokinetic profiles in marketable drugs (29).
Molecular fragments were subjected to validated docking study into BACE1 active site. Auto Dock scores were used to rank docked fragments. Experimental biological data (In-vitro FRET Assay) were correlated well with our estimated free binding energies (30). Calculated free binding energies, LE and BEI values for our studied fragment are introduced in Table 2.
Docked binding energies, ligand efficiencies (LEs) and binding energy indices (BEIs) of fragments derived from crystallographic BACE-1 inhibitors
Docked energies (BEs) and related efficiency indices were normalized and plotted (Figure 4). The distribution of LE and BEI patterns were relatively the same while estimated binding energies followed a different pattern (Figure 4). According to the plots, some useful hints may be concluded.
- The analogous distribution pattern of LE and BEI efficiency plots may be attributed to the proximity of varying scaffold heteroatoms (N, O and carbon) in a periodic table.
- As would be expected, the biggest difference between LEs and BEIs occurred in the case of BACE-1 inhibiting fragments bearing two sulfur atoms (2F3F, No.4 in Figure 4). Different BEI and LE values could be predicted in scaffolds possessing heavier heteroatoms such as sulfur or phosphorous in ring(s) or spacer(s).
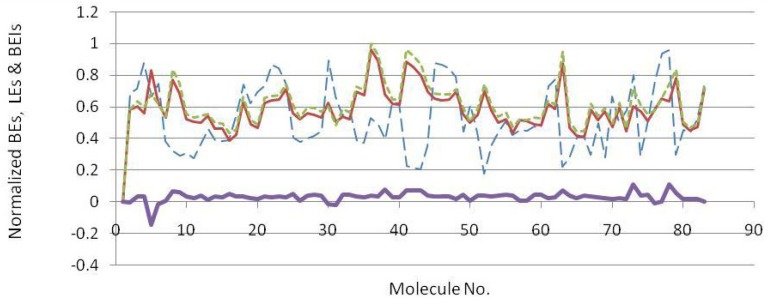
Estimated binding energies could not be often used to define priorities among bioactive compounds while meaningful priorities may be established regarding LEs or BEIs. An appropriate example can be observed in the case of isophthalamide (3CIB, No.44 in Figure 4) and phenoxepine (2EWY, No.3 in Figure 4) derived fragments. In our docking study, these molecules exhibited identical binding energies to the BACE-1 active site, however, higher BEI and LE scores were assigned to isophthalamides. Crystallographic ligands identified by 2VA6, 2QU3 and 2VA7 codes in PDB database are potent BACE-1 inhibitors in micromolar range (http://www.pdb.org). These molecules are based on the diphenylthiophene (2QU3, Figure 5) and biphenyl dihydropyrimidinone (2VA6 & 2VA7, Figure 5) scaffolds exhibiting relatively low interaction energies in our docking evaluation while showing better BEI and LE indices (Table 3, Figure 4). As another example, benzimidazole fragment (3MSJ, No.78 in Figure 4) was found to be a weak BACE-1 inhibitor considering its docked binding energy. However, focus on the BEI value (and to the less extent LE value) demonstrated the efficiency of this scaffold for possible lead development strategies.
Estimated binding/efficiency scores of the isomeric fragments (BACE-1 inhibitors).
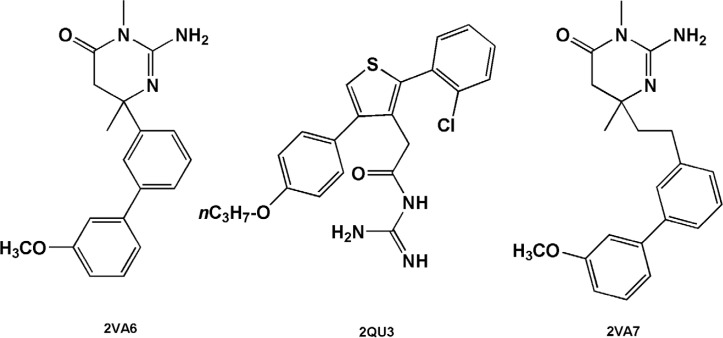
In some cases, high-ranked binding energies, LEs and BEIs were obtained. Chemical fragments possessing this feature provide optimum cases in developing potent BACE1 inhibitors. Some related examples included 3PI5, 3L5C, 3KN0, 2OHT, 3L3A, 2ZE1, 2ZDZ, 2QP8, 3IN4, 2ZJN and 3MSL based fragments. Our results revealed that benzylpiperazines (2ZJJ and 2ZJK) had higher docked energies among evaluated compounds. 3LPJ derived fragment exhibited highest estimated docked energy and also good efficiency indices. 3LPJ derived fragment may be an efficient scaffold to develop potent BACE-1 inhibitors (Table 2). This particular case can also be assessed from another aspect. Based on docking outputs, isophthalamides and benzylpiperazines have been found to be efficient BACE-1 inhibitors and one of the most potent compounds in PDB database (3LPJ) is a chimeric (hybrid) molecule comprising of these two building blocks. Considering efficient building blocks, similar trends may be extended for further hybrid potent BACE-1 inhibiting structures.
The case of 4-amino-benzylpiperidines is noticeable. Chemical structures bearing mercaptobutanamide side chain exhibit higher efficiencies and lower docked energies (2ZJH, 2ZJI and 2ZJL, Figure 6) while 4-amino-benzylpiperidines comprising phenoxyacetamide side chain (2ZJN, 2ZJM, Figure 6) were found to be more potent BACE-1 inhibitors (retaining acceptable BEI and LE values, Table 3).
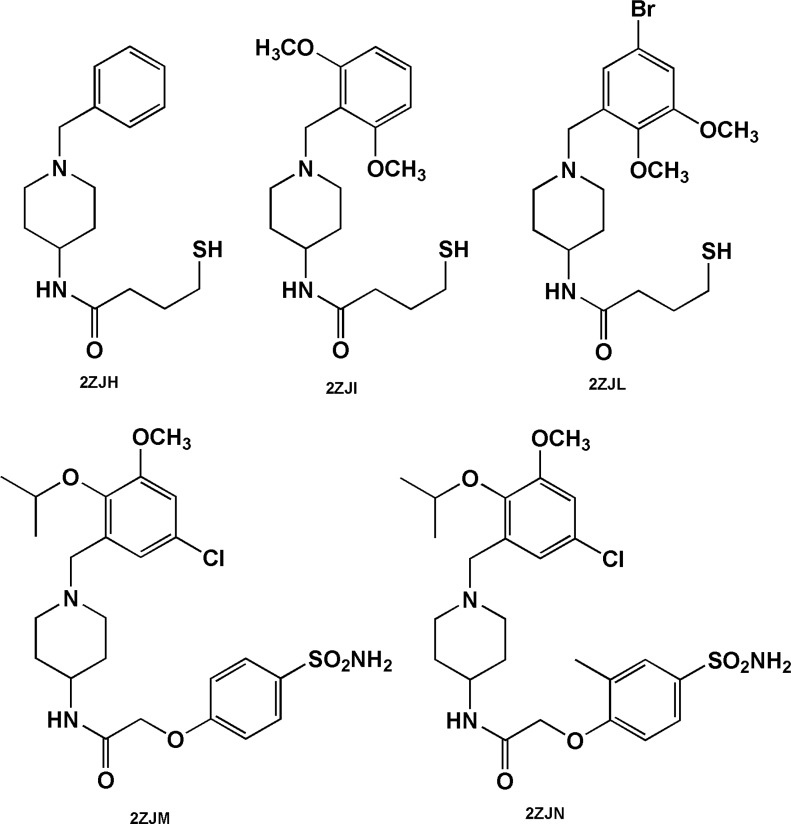
Isosterism and ligand efficiency values
2,5-diphenylpyrrole based fragment (2QU2) proved to be a little more potent and efficient than 2,4-diphenylthiophene (2QU3) (Table 3). Similar situations were observed for the other isosteric molecules (3INH and 3LHG; 3IN3 and 3IN4). Some isosteric substitutions provided potential hydrogen donor/acceptor sites for binding to the receptor (2OHM and 3KMY, 3LPJ and 3CIB, Figure 7). Due to the proximity of carbon and nitrogen atoms in a periodic table (one unit of mass difference) higher efficiency values would be expected for these fragments. In some cases, these isosteric replacements cause significant enhancements in potency results (2ZJK and 2ZJL).
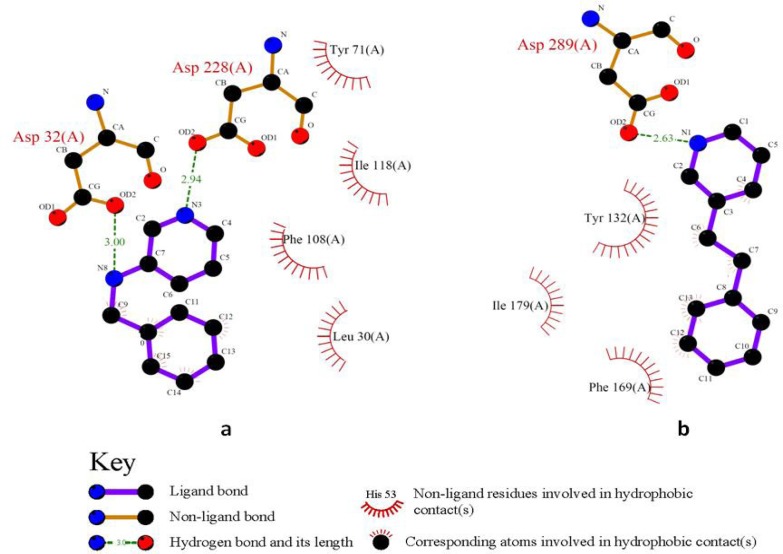
Constitutional isomeric structures
The case of constitutional isomeric fragments is also worth mentioning. As a typical example in our study, we refer to quinoline derivatives. Quinoline (2OHL) exhibited superior potency and more efficiency than its isomer; isoquinoline (2OHK) in binding to BACE-1 active site. Our results confirmed that structural isomers may have a determinant effect on both binding energies and efficiencies. In this particular case, both of the isomers show similar enhancement patterns in their potency and efficiency profiles. For further information, some constitutional isomers and their normalized docking-based energies and efficiencies are depicted in Table 3.
Side chains in designing potent BACE-1 inhibitors
For further evaluation of the side chain effect on ligand potency, we focused on identical isophthalamide simplified structures derived from different BACE-1 inhibitors (31-33). Experimental BACE-1 inhibitory activities for a number of crystallographic ligands and binding characteristics of their docked fragments are summarized in Table 4.
Experimental IC50s for crystallographic BACE-1 inhibitors and structure-based binding indices in related fragments
PDB code | IC50s of | Docked fragments | ||
---|---|---|---|---|
BE | LE | BEI | ||
1W51 | 500 | -10.55 | 0.377 | 20.67 |
2P83 | 11 | -10.96 | 0.391 | 21.47 |
3L58 | 15-80 | -11.84 | 0.377 | 23.20 |
Priority order | 2P83>3L58>1W51 | 3L58>2P83>1W51 | 2P83>1W51≈3L58 | 3L58>2P83>1W51 |
Experimental data showed that the priority order was not retained in docked fragments due to the absence of side chains. Studied fragments possessed 4 heteroatoms in their structures (2 nitrogen atoms and 2 oxygen atoms) and this may also to some extent explain the dissimilar observed priority orders for LE and BEI values. BE and BEI indices are in good agreement with each other. Based on the results, the well-orientated 3L58 derived fragment is possibly an efficient starting point to develop BACE- 1 inhibitors. Different biological activities of cognate structures could be attributed to the absence or presence of side chains in the meta position of isophthalamide ring (Figure 8). It should be added that all the evaluated structures possessed the same stereochemistry.
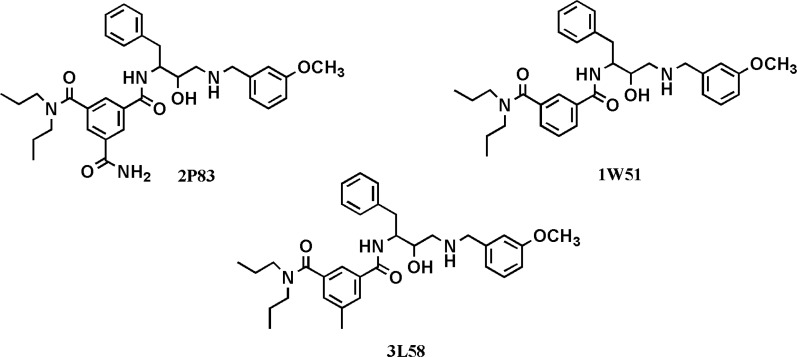
These typical cases may be beneficial in quantification studies of side chain effects on binding potency of biologically active molecules.
Conclusion
Docking simulation was applied in comparative study of BACE-1 inhibitors. We used a simple shape-based dissection method to analyze compounds obtained from PDB source. This classification route was followed by a fragment-based docking strategy to rank the structural features of BACE-1 inhibitors. The merit of such structure-based efficiency indices for various biological targets has been well revealed in other studies. Our case study confirmed that larger BACE-1 inhibitors may require special attention in their design toward efficiency indices. According to this, considering molecular weights rather than number of heavy atoms would produce less biased results. This technique leads to more realistic view at the individual atom participations in binding to the receptor. Comparative studies revealed that N-(4-(benzylamino)-3-hydroxy-1-phenylbutan- 2-yl) benzamide could be an appropriate fragment for further bioactive molecular modifications. Other efficient fragments were arylpiperazine, imidazolidinone, pyrimidinone and benzoimidazole. Our study confirmed that the evaluation of the ligand-receptor interactions on the basis of ligand efficiency indices (binding energy per atom and pKi per MW) rather than free energy of binding (ΔG) could be a helpful strategy in recognizing potential candidates for further bioactive molecular developments.
Acknowledgements
References
-
1.
Querfurth HW LF. Alzheimers disease. N. Engl. J. Med. 2010;362:329-44. [PubMed ID: 20107219].
-
2.
Hirtz D, Thurman DJ, Gwinn-Hardy K, Mohamed M, Chaudhuri AR, Zalutsky R. How common are the “common” neurologic disorders? Neurology. 2007;68:326-37. [PubMed ID: 17261678].
-
3.
Hardy J. The amyloid hypothesis for Alzheimer’s disease: a critical reappraisal. J. Neurochem. 2009;110:1129-34. [PubMed ID: 19457065].
-
4.
Pietrzik C, Behl C. Concepts for the treatment of Alzheimer›s disease: molecular mechanisms and clinical application. Int. J. Exp. Pathol. 2005;86:173-85. [PubMed ID: 15910551].
-
5.
Selkoe DJ. Soluble oligomers of the amyloid (beta)-protein impair synaptic plasticity and behavior. Behav. Brain Res. 2008;192:106-13. [PubMed ID: 18359102].
-
6.
Albert JS. 4 Progress in the Development of (beta)-secretase Inhibitors for Alzheimer›s Disease. Progr. Med. Chem. 2009;48:133-61.
-
7.
Ziora Z, Kimura T, Kiso Y. Small-sized BACE1 inhibitors. Drugs Future. 2006;31:53-63.
-
8.
Lipinski CA. Drug-like properties and the causes of poor solubility and poor permeability. J. Pharmacol. Toxicol. 2000;44:235-49.
-
9.
Lipinski CA, Lombardo F, Dominy BW, Feeney PJ. Experimental and computational approaches to estimate solubility and permeability in drug discovery and development settings. Adv. Drug Deliver. Rev. 1997;23:3-25.
-
10.
Lipinski CA. Lead-and drug-like compounds: the rule-of-five revolution. Drug Discov. Today Technol. 2004;1:337-41.
-
11.
Muegge I. Selection criteria for drug-like compounds. Med. Res. Rev. 2003;23:302-21. [PubMed ID: 12647312].
-
12.
Andrews PR, Craik DJ, Martin JL. Functional group contributions to drug-receptor interactions. J. Med. Chem. 1984;27:1648-57. [PubMed ID: 6094812].
-
13.
Abad-Zapatero C, Metz JT. Ligand efficiency indices as guideposts for drug discovery. Drug Discov. Today. 2005;10:464-69. [PubMed ID: 15809192].
-
14.
Tanaka D TY, Shiyama T, Nishimura T, Chiyo N, Tominaga Y, Sawada N, Mimoto T, Kusunose N. A Practical Use of Ligand Efficiency Indices Out of the Fragment-Based Approach: Ligand Efficiency-Guided Lead Identification of Soluble Epoxide Hydrolase Inhibitors. J. Med. Chem. 2011;54:851-57. [PubMed ID: 21192659].
-
15.
Reynolds CH TB, Bembenek SD. Ligand binding efficiency: Trends, Physical Basis, and Implications. J. Med. Chem. 2008;51:2432-38. [PubMed ID: 18380424].
-
16.
Hetényi C, Paragi G, Maran U, Timár Z, Karelson M, Penke B. Combination of a modified scoring function with twodimensional descriptors for calculation of binding affinities of bulky, flexible ligands to proteins. J. Am. Chem. Soc. 2006;128:1233-39. [PubMed ID: 16433540].
-
17.
Hete´ nyi C, Maran U, Garcı´a-Sosa AT, Karelson M. Structure-based calculation of drug efficiency indices. Bioinformatics. 2007;23:2678-85. [PubMed ID: 17804436].
-
18.
McGovern SL, Shoichet BK. Information decay in molecular docking screens against holo, apo, and modeled conformations of enzymes. J. Med. Chem. 2003;46:2895-907. [PubMed ID: 12825931].
-
19.
Chang MW, Ayeni C, Breuer S, Torbett BE. Virtual Screening for HIV Protease Inhibitors: A Comparison of AutoDock 4 and Vina. PLoS ONE. 2010;5:e11955.
-
20.
-
21.
Morris GM, Huey R, Lindstrom W, Sanner MF, Belew RK, Goodsell DS, Olson AJ. AutoDock4 and AutoDockTools4: Automated docking with selective receptor flexibility. J. Comput. Chem. 2009;30:2785-91. [PubMed ID: 19399780].
-
22.
Vriend G HR, van Aalten T. WHAT IF manual. Heidelberg Germany: European Molecular Biology Laboratory; 1997.
-
23.
Baxevanis AD. Current protocols in bioinformatics. John Wiley & Sons Inc; 2002. 6 p.
-
24.
Wallace AC, Laskowski RA, Thornton JM. Ligplot-a program to generate schematic diagrams of protein ligand interactions. Protein Eng. 1995;8:127-34. [PubMed ID: 7630882].
-
25.
Bemis GW, Murcko MA. The properties of known drugs. 1. Molecular frameworks. J. Med. Chem. 1996;39:2887-93. [PubMed ID: 8709122].
-
26.
Hansen PJ, Jurs PC. Chemical applications of graph theory. Part I. Fundamentals and topological indices. J. Chem. Educ. 1988;65:574-79.
-
27.
Hopkins AL, Groom CR, Alex A. Ligand efficiency: a useful metric for lead selection. Drug Discov. Today. 2004;9:430-31. [PubMed ID: 15109945].
-
28.
Orita M, Ohno K, Niimi T. Two () Golden Ratio’indices in fragment-based drug discovery. Drug Discov. Today. 2009;14:321-28. [PubMed ID: 19028598].
-
29.
Wenlock MC, Austin RP, Barton P, Davis AM, Leeson PD. A comparison of physiochemical property profiles of development and marketed oral drugs. J. Med. Chem. 2003;46:1250-56. [PubMed ID: 12646035].
-
30.
Razzaghi-Asl N, Ebadi A, Edraki N, Mehdipour A, Shahabipour S, Mir R. Response surface methodology in docking study of small molecule BACE-1 inhibitor. J. Mol. Mod. 2012.
-
31.
Patel S, Vuillard L, Cleasby A, Murray CW, Yon J. Apo and Inhibitor Complex Structures of BACE ((beta)-secretase). J. Mol. Biol. 2004;343:407-16. [PubMed ID: 15451669].
-
32.
Zhu Z, Sun ZY, Ye Y, Voigt J, Strickland C, Smith EM, Cumming J, Wang L, Wong J, Wang YS. Discovery of cyclic acylguanidines as highly potent and selective -site amyloid cleaving enzyme (BACE) inhibitors: part i inhibitor design and validation (1). J. Med. Chem. 2010;53:951-65. [PubMed ID: 20043696].
-
33.
Kortum SW, Benson TE, Bienkowski MJ, Emmons TL, Prince DB, Paddock DJ, Tomasselli AG, Moon JB, LaBorde A, TenBrink RE. Potent and selective isophthalamide S2 hydroxyethylamine inhibitors of BACE1. Bioorg. Med. Chem. Lett. 2007;17:3378-83. [PubMed ID: 17434734].