Abstract
Keywords
KCL-SDS Precipitation Reaction Flocculation HPMC-SDS Interaction Megestrol Acetate Suspensions
Introduction
Phase separation in drug dispersions and the eventual caking of sediment is the major stability issue encountered in pharmaceutical suspension formulation. Preparation of physically stable suspensions includes the use of structured vehicles and the flocculation of the dispersion suspenoids. It is best to apply both these methods to prepare physically stable suspensions with uniform and elegant appearance (1).
In a deflocculated dispersion, the particle charge exceeds the critical zeta potential, and therefore the repulsive forces between suspenoids supersede the London-Van der Waals attractive forces. The particles with supracolloidal sizes settle due to gravity and the impaction of the sediment by the weight of upper layers will over time lead to formation of compact and non-redispersible sediment called “Cake”. Flocculation has been defined as formation of voluminous and porous network structure of weakly bound particles that is resistant to caking and makes the dispersions easily redispersible (1, 2).
Different agents have been studied for particle flocculation. This includes electrolytes, surfactants, hydrocolloid polymers and polyelectrolytes. Various mechanisms of flocculation in pharmaceutical suspensions have been proposed in the literature (1, 3-4).
Flocculation by zeta potential reduction or charge neutralization is induced by decrease in the electrostatic repulsive barrier originating from the Double layer. The attractive forces of Van der Waals overwhelm and this results in particle aggregation. DLVO theory has been used to interpret this phenomenon in pharmaceutical suspensions (1, 3).
Chemical bridging has been described as aggregation by chemical interaction between the adsorbed ions extending from the particle surface and media precipitation ions and usually happens when a surfactant or macrogol has affinity to an electrolyte (2, 4).
Flocculation can also be brought about by polymer or polyelectrolyte chains in low concentrations through formation of physical bridges. This is called bridging flocculation and it generally happens when high molecular weight polymer adsorbs on the surface without saturating it (5).
In liquid bridging, the flocculation is usually achieved by the use of low HLB surfactants and it can be described as follows: nonionic low HLB surfactants form hazy solutions which contain many large micelles. Upon incorporation of the suspension particles, a saturated layer of surfactants will form around the particles. This surrounding liquid links particles like a bridge, resulting in particle aggregation. It is thought that flocculation of suspensions by nonionic surfactants and polyols is related to dehydration of the particles and subsequent liquid bridging (6, 7).
SDS (sodium dodecyl sulfate) is an anionic surfactant with many applications in science and industry and it may be used as detergent, wetting agent, emulsifier, dispersant, or foaming agent. SDS has good wetting properties and it can act as a dispersant or a flocculating agent in suspensions and emulsions depending on the dispersion system characteristics (8). Its use in the DNA extraction is to aid in lysing cells and solubilizing proteins. In SDS-PAGE electrophoresis technique, it is used to unravel proteins to their primary conformation and impart a uniform charge density to the polypeptides. Aqueous solutions of SDS have also been useful to disperse and suspend nanotubes (9-11, 25).
Megestrol acetate suspensions containing SDS have been seen to deflocculate in concentrations above 0.02% (w/v) making them unstable (12). SDS exhibits different interactions within the dispersion systems with polymers or other dispersion constituents. This may prove useful in certain circumstances. SDS reduces the deleterious effect of magnesium stearate on dissolution rate of the oral formulations which results from physical interaction of SDS and magnesium stearate during the mixing process (13). In SDS-hemoglobin analysis, the hydrophobic part of the SDS acts upon the globin subunit and the interaction of the hydrophilic part with oxidized iron subunit produces a stable reaction product that can be analyzed (14). Interaction of SDS micelles with Fe3+ and Al3+ has also been studied in adsorptive micellar flocculation (AMF) in which pollutant containing micelles can be flocculated and filtered out (15). In DNA extraction, SDS-KCl reaction has been employed to facilitate the separation of the SDS bound proteins (9). Unlike soaps, SDS is compatible with dilute acids and magnesium and calcium ions (8). In concentrations well above its cmc (critical micelle concentration), SDS induces particle aggregation in some dispersions through depletion flocculation (5).
In view of numerous applications of the SDS and its dual efficacy both as a dispersing and an aggregating agent in the dispersion systems, attempts were made in the present work to study the interaction of the SDS-megestrol acetate dispersions with salts, polyols and polymers to examine the effect of SDS in flocculation of megestrol acetate suspensions.
Experimental
Megestrol acetate was purchased from Pharmabios, Spain as micronized powder. Xanthan gum was obtained as a gift sample from Hakim Pharmaceutical Company, Iran. NaCMC, HPMC, MC, Glycerol, PEG 400, Sorbitol, Sodium Chloride, Potassium Chloride, Sodium Dihydrogen Phosphate, Potassium Dihydrogen Phosphate were all purchased from Merck, Germany.
The suspensions were prepared as follows: polymer, electrolyte and the surfactant stock solution were made one day prior to suspension formulation. Polymer stock solutions were allowed to stand overnight to ensure adequate hydration. Surfactant solution was added to the calculated amount of megestrol acetate to make a concentrated uniform dispersion of megestrol acetate. The dispersion was later moved into a graduated cylinder and the electrolyte solution was added to produce flocculation. The dispersion was then promptly admixed into the polymer solution to obtain a uniform homogenous suspension. Each prepared suspension was moved to another container for stability observation.
General tests performed
Prepared suspensions were evaluated for their appearance, sedimentation volume, sedimentation rate and redispersibility. Sedimentation volume (SV or F) was the main parameter evaluated in this study and was measured as fraction of the total sediment volume. The results were expressed as percentage. Particle size analysis and zeta potential measurements were made using Malvern instruments.
Wetting
Using the sessile drop method, the contact angle of the distilled water on megestrol acetate was measured at well above 90 degrees. This is in agreement with the claimed high hydrophobicity of the megestrol acetate. SDS was used in this study as the wetting agent. Preparations of megestrol acetate dispersion with different concentrations of SDS were made in order to show how SDS affects the sedimentation volume.
Flocculation
NaCl, NaH2PO4 (NaDP), KCl and KH2PO4 (KDP) were used in different concentrations and the Sedimentation Volume (SV) was measured for the SDS-megestrol acetate dispersions after two weeks. Next, SDS was added incrementally in different preparations containing KCl and KH2PO4, and the sedimentation volume of the preparations made (18 formulations) was measured after two weeks.
In another set of experiments, glycerol (G), propylene Glycol (PG), sorbitol and polyethylene glycol (PEG 400) were used to evaluate their effect on megestrol acetate dispersions containing SDS.
Polymers
Hydrocolloid polymers including CMC, HPMC, MC and xanthan gum were incorporated in 0.1-0.6%(w/v) concentrations and the sedimentation volume of the preparations were compared and evaluated.
Results
Wetting
Figure 1 shows the effect of SDS on sedimentation volume of megestrol acetate dispersion. It is evident that increasing the SDS concentration reduces the sedimentation volume. This effect can be attributed to negative charging of the particles that hinders particles from aggregation. The large particles will eventually settle and compact upon long term storage.
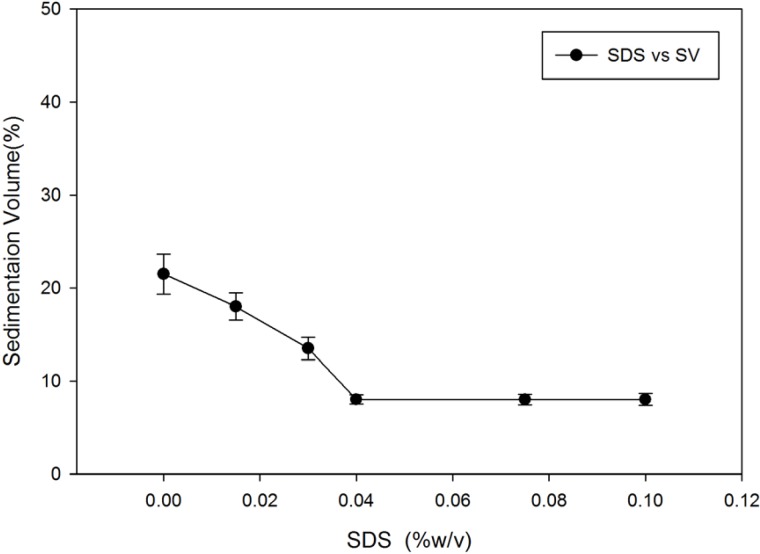
Flocculation
Sedimentation results after two weeks in dispersions containing electrolytes are presented in Table 1. It shows that potassium salts in contrast to sodium salts increase sedimentation volume. SDS was then used in different concentrations in dispersions containing KCl and KH2PO4. Formulations prepared in this step showed good sedimentation behavior and as can be seen in Figure 2, SDS increments noticeably increase the sedimentation volume and it seems to reach plateau at concentrations around 2-2.5%(w/v) of KCl or KH2PO4.
Effect of different electrolytes on sedimentation volume of megestrol acetate dispersions with 0.02%(w/v) SDS. SV: Sedimentation Volume, KDP: KH2PO4, NaDP: NaH2PO4. (Values are means of 3 replicates ±SD, n=3).
SV at 1.5% Electrolyte%Concentration | SV at 1% Electrolyte%Concentration | SV at 0.5% Electrolyte%Concentration | Electrolyte |
---|---|---|---|
65±3.0 | 60±3.5 | 52±2.5 | KCl |
50±2.5 | 45±2.5 | 34±4.5 | KDP |
13±1.5 | 13±1.5 | 13±1.5 | NaDP |
13±2.0 | 13±2.0 | 13±2.0 | NaCl |
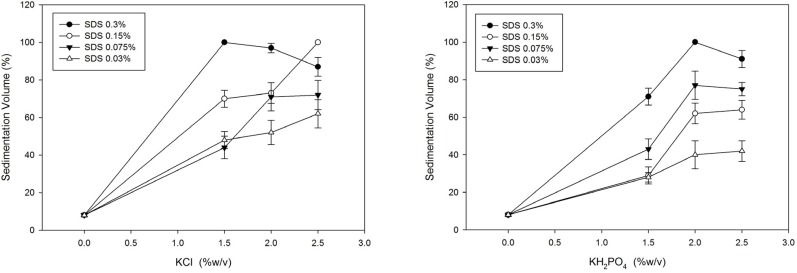
Polyols and polymers
At this step the polyols including glycerol, propylene glycol and sorbitol were used in different concentrations. Use of polyols resulted in high sedimentation volumes in the dispersion. PEG 400 produces deflocculated dispersions resulting in formation of compact sediment.
Hydrophilic polymers including MC, HPMC, NaCMC and xanthan gum were used in two previously prepared formulations containing KCl and SDS to evaluate polymer effects on sedimentation volume and the results have been shown in Table 2. It can be seen that the suspensions with nonionic polymers (HPMC and MC) in contrast to the anionic ones (xanthan gum and NaCMC) are deflocculated with the sedimentation volume at around 8%.
Effect of different polymers on sedimentation volume of megestrol acetate dispersions. SV: Sedimentation Volume. (Values are means of 3 replicates ±SD, n=3). A and B formulations represent the preparations containing different amount of SDS and electrolyte
MC)SV%( | HPMC)SV%( | NaCMC)SV%( | Xanthan Gum)SV%( | Formulation | Polymer Concentration)w/v%( |
---|---|---|---|---|---|
8±0.5 | 8±1.0 | 45±3.5 | 68±3.5 | A | 0.1 |
8±1.5 | 8±1.0 | 22±2.5 | 69±2.5 | B | |
8±0.5 | 8±0.5 | 55±1.5 | 86±3.0 | A | 0.2 |
8±1.0 | 8±1.0 | 33±4.5 | 92±1.5 | B | |
8±0.5 | 8±0.5 | 57±4.5 | 100±0.0 | A | 0.4 |
8±0.5 | 8±1.0 | 41±2.0 | 97±1.5 | B | |
8±1.0 | 8±0.5 | 58±4.5 | 100±0.0 | A | 0.6 |
8±0.5 | 8±1.0 | 47±3.0 | 100±0.0 | B |
Figure 3 shows the better efficacy of xanthan gum in enhancing the dispersion sedimentation volumes compared with NaCMC.
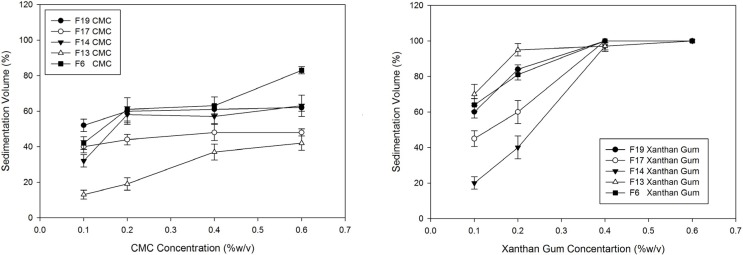
Figure 4 shows the result of the particle size analysis and zeta potential measurement of a selected formulation with SDS 0.15%, KH2PO4 2% and xanthan gum 0.2%.
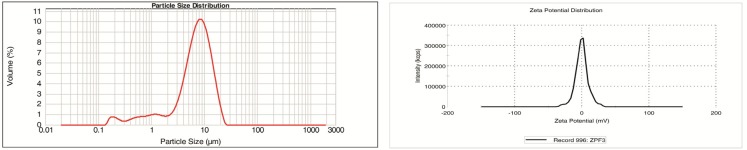
Discussion
Physically stable suspensions that do not form a caked deposit upon standing can be prepared by the use of structured vehicles and flocculation of the dispersion particles. To increase the sedimentation volume of the suspension, it is necessary to make a network structure in the system to prevent settling. This type of structurization can be produced by internal structurization (the structure produced by the dispersion medium or “microstructure”, e.g. flocculation) or external structurization (structure which is formed by the vehicle, e.g. viscosity imparting vehicles).
Due to the hydrophobic nature of megestrol acetate, surfactants can be used to aid easy and uniform dispersion of the particles in the aqueous media. Surfactants may cause deflocculation of the dispersions. Deflocculation in the presence of ionic surfactants is typically due to the acquired high zeta potential of the particles (1).
In the present work, effect of electrolyte, polyols and hydrocolloid polymers on SDS dispersions of megestrol acetate was studied. Considering the chemical structure of megestrol acetate particles in distilled water, H+ ions adsorb on the hydroxyl (–OH) functional groups and therefore the particles will be slightly positively charged. Addition of SDS and its subsequent surface adsorption imparts high negative charge to the particles. This will reduce the particle aggregation and phase separation occurs due the mass effect and settling of the suspenoids.
SDS is incompatible with some alkaloidal salts and precipitates with lead and potassium salts. Calcium and magnesium salts do not show this property (8). SDS-KCl precipitation has proved useful in biological purification and protein analysis procedures. KCl or potassium acetate are employed to extract and purify SDS solubilized proteins and DNA structures (9, 16-17). We tested effect of such K+-SDS interaction in flocculating the SDS-megestrol acetate dispersions. Sodium salts were used as control and it was observed in the experiments that only potassium salts induce flocculation.
Upon addition of KCl, insoluble crystals of potassium dodecyl sulfate (KDS) form. When this happens, the surface charge of the megestrol acetate particles change and they no longer repulse each other due to the high negative charge. In fact the precipitation reaction brings about an alteration in surfactant films about the particles which unlike the surfactant film formed in previous steps, facilitates the linking of the particles allowing for aggregation of the suspenoids.
Addition of polyols and hydrocolloid polymers to the megestrol acetate-SDS dispersions produced different results in terms of stability. The low molecular weight polyols glycerin, propylene glycol and sorbitol demonstrated no incompatibility and their use enhanced suspension appearance and stability. SDS was used at 0.02% in these preparations which is lower than the amount of SDS used in the dispersions containing potassium salts. The sedimentation volume observed was generally around 40-60% and was not as high as the sedimentation volume seen in the previous potassium salt containing suspensions with higher amounts of SDS.
Incorporation of HPMC, MC, and PEG resulted in defloccualtion in the dispersions containing SDS and potassium salts. The dispersions were therefore considered as unstable. On the other hand, K+-SDS dispersions of megestrol acetate with charged polymers of NaCMC and xanthan gum showed no compatibility issue and even the stability of such suspensions increased markedly due to the introduction of such polymers as viscosity enhancers.
Addition of HPMC, MC and PEG possibly induces an interaction with the SDS in the dispersions. HPMC-SDS interactions have been studied in some recent works (18-20). In summary, in these studies an interaction region with specific concentration range for SDS and HPMC was identified in which such an interaction results in increased viscosity of the system. SDS forms complexes with the polymer chains of HPMC at the cac (critical association concentration) which is lower than cmc of the pure surfactant solution (21). The HPMC-SDS interaction in a preparation of a sunflower oil in water emulsion resulted in better emulsion stability and higher encapsulation efficiency after spray drying to produce the corresponding microcapsules (18). The SDS amounts used in our work were generally lower than the interaction region for HPMC and SDS found by the aforementioned studies; however, the high concentration of salts used in our experiments should also be factored in because the surfactant-polymer binding can be affected by salts. In one report for example, NaBr significantly decreased both the cmc and the cac of the SDS in presence of PVP and a related neutral copolymer (22). It is thought that in both the megestrol acetate suspension and the sunflower oil emulsion, the HPMC/SDS complex forms a more compact layer at the interface. This compact layer is usually considered favorable in emulsions as it hinders aggregation. However in the megestrol acetate dispersions in which the particle aggregation is desirable, such a HPMC/SDS complex formation results in defloccualtion and the subsequent sedimentation settling in the suspensions.
SDS interactions with MC and PEG have also been studied in the literature and similar mechanism appears to be responsible for the observed deflocculation of the dispersions in our study (23, 24). Xanthan gum and NaCMC as expected showed no incompatibility with SDS due to their ionic charge which reduces the chance of such unwanted interactions with anionic surfactants.
Conclusion
As discussed previously, potassium salts can be used to induce flocculation of dispersions pre-wetted by SDS. SDS is soluble in water while KDS is less soluble in the aqueous media. Addition or presence of sodium ions does not induce flocculation while potassium ions cause the megestrol acetate particles to aggregate. We found that such interaction is useful to flocculate megestrol acetate-SDS dispersions. This approach could be universal and its utilization may prove effective in any similar dispersion systems. Another finding of this study is that the uncharged hydrocolloid polymers HPMC and MC reverse the flocculation produced in this manner. This is believed to be caused by formation of SDS/HPMC complex in the form of a compact layer at the interface which hinders particle aggregation.
Acknowledgements
References
-
1.
Nutan MTH, Reddy IK. General Principles of Suspensions. In: Kulshreshta AK, Singh ON, Wall GM, editors. Pharmaceutical suspensions. New York: Springer; 2010. p. 39-67.
-
2.
Martin AN. Coarse Dispersions. In: Sinko PJ, editor. Martin›s Physical Pharmacy and Pharmaceutical Sciences. 5th ed. Philadelphia: Lippincott Williams and Wilkins; 2006. p. 499-530.
-
3.
Nash RA. Pharmaceutical suspensions. In: Liebermann HA, Rieger MM, Banker GS, editors. Pharmaceutical dosage forms: Disperse system. Volume 1. New York: Marcel Dekker; 1988. p. 151-198.
-
4.
Swarbrick J, Rubino JT, Rubino OP. Corase Dispersions. In: Troy DB (ed, editor. Remington The Science and Practice of Pharmacy. 21st ed. Philadelphia: Lippincot Williams & Wilkins; 2005. p. 319-37.
-
5.
Furusawa K, Sato A, Shirai J, Nashima T. Depletion flocculation of latex dispersions in ionic micellar systems. J. Colloid Interface Sci. 2002;253:273-78. [PubMed ID: 16290859].
-
6.
Zatz JL, Lue RY. Flocculation of suspensions containing nonionic surfactant by sorbitol. J. Pharm. Sci. 1987;76:157-61. [PubMed ID: 3572755].
-
7.
Zatz JL, Lue RY. Effect of polyols on physical stability of suspension containing nonionic surfactant. J. Soc. Cosmet. Chem. 1982;33:1749-55.
-
8.
Rowe CR, Sheskey PJ, Owen SC. Handbook of Pharmaceutical Excipients. 5th ed. London, Washington DC: Pharmaceutical Press, American Pharmaceutical Association; 2006. 688 p.
-
9.
Li JKK, Johnson T, Yang YY, Shore V. Selective separation of virus proteins and double-stranded RNAs by SDS-KCl precipitation. J. Virol. Methods. 1989;26:3-16. [PubMed ID: 2556424].
-
10.
Smith BJ. SDS polyacrylamide gel electrophoresis of proteins. Methods Mol. Biol. 1994;32:32-34.
-
11.
Bystrzejewski M, Huczko A, Lange H, Gemming T, Büchner B, Rümmeli MH. Dispersion and diameter separation of multi-wall carbon nanotubes in aqueous solutions. J. Colloid Interface Sci. 2010;345:138-142. [PubMed ID: 20171648].
-
12.
Atzinger AE, Bequette RJ, Davis RE, inventors. Bristol-Myers Squibb Company, assignee. Megestrol Acetate Formulation. US Patent. 5338732. 1994.
-
13.
Li-Hua W, Chowhan ZT. Drug-excipient interactions resulting from powder mixing. V. Role of sodium lauryl sulfate. Int. J. Pharm. 1990;60:61-78.
-
14.
Karsan A, Maclaren I, Conn D, Wadsworth L. An evaluation of hemoglobin determination using sodium lauryl sulfate. Am. J. Clin. Pathol. 1993;100:123-26. [PubMed ID: 8356944].
-
15.
Talens-Alesson FI, Hall ST, Hankins NP, Azzopardi BJ. Flocculation of SDS micelles with Fe3+. Colloid Sur. 2004;204:85-91.
-
16.
Huang Y, Vanscheeuwijck P, Regan JW. Precipitation with KCl improves the chemical cross-linking of GST-fusion proteins to agarose after solubilization with SDS. Biotechniques. 1993;15:989-92. [PubMed ID: 8292352].
-
17.
Carraru U, Rizzi C, Sandri M. Effective recovery by KCl precipitation of highly diluted muscle proteins solubillized with sodium dodecyl sulfate. Electrophoresis. 1991;12:1005-10. [PubMed ID: 1815951].
-
18.
Petrovic LB, Sovilj VJ, Katona JM, Milanovic JL. Influence of polymer-surfactant interactions on o/w emulsion properties and microcapsule formation. J. Colloid Interface Sci. 2010;342:333-39. [PubMed ID: 19948340].
-
19.
Sovilj VJ, Petrović LB. Influence of hydroxypropylmethyl cellulose–sodium dodecylsulfate interaction on the solution conductivity and viscosity and emulsion stability. Carbohydr. Polym. 2006;64:41-49.
-
20.
Silva SMC, Antunes FE, Sousa JJS, Valente AJM, Pais AA. New insights on the interaction between hydroxypropylmethyl cellulose and sodium dodecyl sulfate. Carbohydr. Polym. 2011;86:35-44.
-
21.
Lopez-Esparza R, Guedeau-Boudeville MA, Gambin Y, Rodriguez-beas C, Maldonado A, Urbach W. Interaction between poly(ethylene glycol) and two surfactants investigated by diffusion coefficient measurements. J. Colloid Interface Sci. 2006;300:105-110. [PubMed ID: 16678840].
-
22.
Fox GJ, Bloor DM, Holzwarth JF, Wyn-Jones E. Use of isothermal titration microcalorimetry to monitor the adsorption/desorption processes of sodium dodecyl sulfate with neutral polymers. Langmuir. 1998;14:1026-30.
-
23.
Bosco SJ, Zettl H, Crassous JJ, Ballauff M, Krausch G. Interactions between methyl cellulose and sodium dodecyl sulfate in aqueous solution studied by single molecule fluorescence correlation spectroscopy. Macromolecule. 2006;39:8793-98.
-
24.
Guo W, Sun YW, Luo GS, Wang YJ. Interaction of PEG with ionic surfactant SDS to form template for mesoporous material. Colloids Surf A. 2005;252:71-77.
-
25.
Eslami N, Kermanshahi RK, Erfan M. Studying the stability of S-Layer protein of lactobacillus acidophilus ATCC 4356 in simulated gastrointestinal fluids using SDS-PAGE and circular dichroism. Iran J. Pharm. Res. 2013;Suppl:47-56.