Abstract
Keywords
UGT Polymorphism Mycophenolate mofetil Renal transplantation
Introduction
Based on the National Kidney Foundation’s (NKF) guidelines, chronic kidney disease (CKD) is defined as kidney damage and/or reduced kidney function. Therefore CKD is defined as the presence of evidence of kidney damage with an abnormal glomerular filtration rate (GFR) or alternatively, for at least 3 months, by a GFR < 60 ml/min/1.73 m2 (1). End–stage renal disease (ESRD) occurs when GFR decreases to less than 15 ml/min/1.73 m2 and dialysis or transplantation are required to remove uremic toxins and maintain homodynamic stability (2). In comparison to dialysis, transplantation has become the treatment of choice for patients with ESRD, with significant improvements in quality of life and physiologic parameters (1). By development of newer immunosuppressive agents, such as cyclosporine, tacrolimus, mycophenolate mofetil (MMF), polyclonal and specific monoclonal antibodies, the incidence and intensity of acute rejection has been reduced (1). According to several clinical trials, MMF therapy has been associated with a 50% reduction in the incidence of acute rejection in the first year after transplantation and to a lesser extent in progression of chronic rejection (1). MMF is classified as an antiproliferative antimetabolite (1, 3, 4). It is the mopholinoethyl ester prodrug of mycophenolic acid (MPA) that selectively, reversibly and noncompetitively blocks inosine monophosphate dehydrogenase (IMPDH) required for proliferation of T and B lymphocytes. MMF is almost completely hepatically metabolized to its glucuronide derivative, mycophenolic acid glucuronide (MPAG), which undergoes enterohepatic circulation and is ultimately excreted renally (1). While a strong relationship between AUC (Area under plasma concentration-time curve) of MPA and prevention of acute rejection has been reported, such co-relation has not been established between C0 (Predose plasma concentration) of MPA and clinical outcomes (5, 6). Due to high individual variations in pharmacokinetic parameters of MMF and its interactions with other concomitantly used drugs in renal transplant patients serum concentration monitoring is recommended (5). While co-administration of MMF and cyclosporine decreases the AUC of MPA due to inhibition of enterohepatic circulation (7), MMF interaction with tacrolimus results in inhibition of MPA glucuronidation which consequently increases MPA plasma concentration (8). Interaction between MMF and corticosteroids decreases AUC of MPA due to uridine dipphosphate glucuronosyl transferase (UGT) induction (9). In renal transplant patients, interindividual differences of AUC and C0 of MPA have been reported. These differences have been related to gender, time after transplantation, serum albumin concentration, renal function, concomitant drugs and pharmacogenetic factors (6, 10-13). In addition to differences in absorption, distribution and elimination, MMF metabolism is considered to be important in its interindividual differences (14). Several UGT isoenzymes such as UGT1A1, 1A7, 1A8, 1A9 and 1A10 can metabolize MPA to its glucuronide derivative MPAG, among them UGT1A9 has a more important role in hepatic drug metabolism (15). There are great interindividual differences in gene expression and enzymatic activity of UGT in adults (14, 16). Girard and colleagues showed that UGT1A9 protein level varied by 17 fold and glucuronidation activity varied by 9.5 fold in human liver microsomes (17). One of isoforms studied in this regard is UGT1A8 showing that UGT 1A8*3 A173 Y277 mutation significantly decreases the enzyme activity (18). The most extensive reports in this area are related to single neucleotide polymorphism (SNPs) discovered in UGT1A1, UGT1A7, UGT1A9 and UGT2B7. The polymorphisms found in UGT1A9 are I399, M33T, -2152, -665, -331/-440, -275 (14, 17, 19). Among these SNPs the more important ones are C-2152T and T-275A in promoter region of UGT1A9 that have the most consistent relationship with UGT1A9 expression and level (14). The level of UGT1A9 in carriers of T– 275A SNP was 1.4 times and in carriers of T-275A/ C-2152T SNP was 1.6 times more than non-carriers (14, 17). Although UGT1A9 M33T decreases glucuronidation activity of UGT1A9 and increases MPA plasma levels, C-2152T and T-275A polymorphisms decrease the AUC and C0 of MPA as a result of increase in glucuronidation activity of UGT and inhibition of entrohepatic recirculation of drug which in turn decreases deglucuronidation of MPAG (14, 17). Among the discovered polymorphism, UGT1A9 I399 has the prevalence of 15% in Japanese population. However studies showed that this polymorphism cannot explain the interindividual differences in MPA pharmacokinetics (19). Therefore this study was designed to investigate the existence of UGT1A9 M33T C-2152T and T-275A polymorphism in Iranian renal transplant patients and their probable influences on pharmacokinetic parameters of drug.
Experimental
Study protocol
This was a cross sectional study carried out from March 2008 through December 2008 in Imam Khomeini Hospital affiliated to the Tehran University of Medical Sciences in Iran. Based on previous studies and prevalence of polymorphism reported and statistical calculations, 40 de novo Iranian kidney transplant patients were selected for this study (17, 20). Patients, who voluntarily filled a written consent form, received MMF 2 g daily (Cellcept 500 mg tablet, Roche, Switzerland), cyclosporine and prednisolone as their immunosuppressive regimen. A 3 mL blood at 0, 20, 40, 60, 120, 180, 240, 360, 480, 600 and 720 min after taking 1 g of morning dose of MMF was taken from patients. Plasma was separated by centrifugation at 6000 rpm for 10 min. Both plasma and blood cells were kept at -70 °C for pharmacokinetic and pharmacogenetic studies respectively.
Inclusion and exclusion criteria
Patients with at least 3-month history of post renal transplantation, approximately normal renal function (SrCr≤1.4 mg/dL), cyclosporine level between 100-200 ng/mL taking MMF dosage of 1 g every 12 h who were willing to be hospitalized for 12 h and give 11 blood samples were considered eligible. Exclusion criteria were as follows: acute renal failure, current cytomegalovirus (CMV) infection and gancyclovir administration, receiving polyclonal antibodies, elevated liver enzymes (more than 3 times normal upper limit), gastrointestinal adverse effects of MMF such as severe diarrhea needing MMF dose adjustment and diabetes mellitus.
Pharmacogenetic studies
Genomic DNA was extracted from EDTA-treated whole blood by adding distilled water for red blood cell (RBC) lysing; using white blood cell lysing buffer (WBCLB), Tris 10 mM, EDTA 10 mM and NaCl 50 mM ; SDS 10%; proteinase K (Fermentas) and saturated NaCl). After extraction, the DNA concentration was determined by spectrophotometeric method. For identification of UGT1A9*3, UGT1A9 C-2152T, and UGT1A9 T-275A polymorphism, polymerase chain reaction (PCR)-restriction fragment length polymorphism (RFLP) method was used. Forward and reverse primers for C-2152T were 5-TTG AGA CAG AGT CGT GCT GTT T-3 and 5-AGG TCA AGG TGG GCG TAT C-3, respectively. For T-275A, we used 5-TCA GTG CTA AGG GCC TTG TT-3 and 5-CCT GTG CTG CAA TGT TAA GTC TA-3 as forward and reverse primers, respectively. For UGT1A9*3 forward and reverse primers were 5-GTT CTC TGA TGG CTT GCA CA-3 and 5-ATG CCC CCT GAG AAT GAG TT-3, respectively (20). The PCR reaction was performed using 25 L of taq DNA polymerase, RED master mix (Ampliqon-Denmark), 100 ng DNA, 0.5 μM of forward and reverse primers and de-ionized water up to 50 μL.
The following PCR amplification conditions were used for UGT1A9 polymorphisms:
-T-275A: 94°C, 4 min, followed by 30 cycles of 94 °C for 30 sec, 55oC for 30 sec and 72 °C for 45 sec and final 72 °C for 10 min;
-C-2152T: 94 °C for 2 min, then 10 cycles of 94 °C for 30sec,65 °C (-0.5 °C /cycle) for 30 sec and 72 °C for 30sec followed by 35 cycles of 94 °C for 30 sec, 60 °C for 30 sec and 72 °C for 30 sec and finally 72 °C for 10 min.
-UGT1A9*3: 94 °C for 130 sec, then 10 cycles of 94 °C for 30 sec, 60 °C (-0.5 °C /cycle) for 30 sec and 72 °C for 30 sec, followed by 35 cycles of 94 °C for 30 sec, 55 °C for 30 sec and 72 °C for 30 sec and final 72 °C for 10 min.
The PCR products for T-275A, C-2152T and UGT1A9*3 were digested with XbaI (Fermentas), MseI (Fermentas) and StyI (Fermentas), respectively. Digestion conditions were as follows: 10 μL of PCR mixture, 3 μL of buffer (10X), 1 μL of enzyme and 16 μL of de-ionized water to reach the final volume of 30 μL. The reaction was incubated for 4 h in 37 °C for XbaI and StyI enzymes and in 65 °C for MseI enzyme. DNA fragments were separated on 3% agarose gels and visualized by ethidium bromide.
Pharmacokinetic studies
-HPLC system
HPLC system was chosen based on previous study (21); A Knauer (Knauer Company, Germany) 1001 HPLC pump and a Knauer (Knauer Company, Germany) 2600 UV detector were used. Chromatographic separation was performed on a Hamilton PRP-1 reversed phase column (250 mm × 4.6 mm i.d., particle size 10 μm- Hamilton company, Reno, Nevada) with the suitable Hamilton guard column (25 × 2.3 mm i.d., particle size 12-20 μm- Hamilton Company, Reno, Nevada). The mobile phase ingredients, flow rate and retention times were the same as the previous study done by Ahadi and et al.( 21).
Stock solutions of 1 mg/mL MPA and naproxen and all sample treatments were performed as described previously (21). Eleven standard concentrations ranged from 0.1-60 μg/mL were prepared in plasma and linear least square method was applied for calibration curve).
-Accuracy, precision, limit of quantification (LOQ) and recovery
Accuracy, Intra-day precision, Inter-day precision, limit of quantification and absolute recovery of MPA were assessed for four QC concentrations (0.25, 1, 12, 40 μg/mL).
Statistical analysis: Statistical analyses were performed using the Statistical Package for Social Scientists (SPSS version 15.0) computer software. A 3-step statistical analysis was designed. At the first step, the preliminary analyses of the Mann-Whitney, student t-test, Spearman’s rank correlation, Kendall’s rank correlation and Pearson correlation were used to assess any relationship between dependent variables, i.e. pharmacokinetics parameters, and possible predictive variables including age, sex, bodyweight, UGT1A9 polymorphism, MMF dose, cyclosporine dose, steroid dose, cotrimoxazole dose, CMV infection, serum levels of creatinine, creatinine clearance and time after transplantation. Predictors which had a relationship with the pharmacokinetic variables with a p-value of less than 0.1 in the above mentioned univariate analysis were eligible to be included in the final model building. Thus, at this step, those predictive variables which were highly unlikely to be associated with the dependent variables were determined and excluded from the multivariate analysis. In the second step, any interaction between eligible variables obtained in the first step, was evaluated using appropriate statistical tests. In the case of significant interaction (p < 0.05), a ridge regression analysis was applied instead of ordinary linear multivariate regression analysis to obtain the final model (the 3rd step).
Results and Discussion
-Demographics and transplantation-related results: All demographics, transplantation-related characteristics and biochemical parameters of patients are summarized in Tables 1 and 2.
Demographic characteristics of patients (Data are presented as mean ± SD).
Parameter | Value | Parameter | Value | |
---|---|---|---|---|
Baseline demographics | Biochemical parameters | |||
Number of patients | 40 | Serum Creatinine (mg/dL) | 1.2±0.2 | |
Recipient gender (female/male) | 11/29 | Hemoglobin (g/dL) | 14.3±1.2 | |
Body weight (kg) | 70.7±11.3 | ALT b (U/L) | 27.5±8.5 | |
Age(yr) | 39.5±12.3 | AST c (U/L) | 22.8±5.8 | |
Total Bilirubin (mg/dL) | 1.2±0.6 | |||
Transplantation-related characteristics | FBS d (mg/dL) | 91.3±7.3 | ||
Time after transplantation (mo) | 41.5±35.6 | TG e (mg/dL) | 145.4±57.5 | |
Re-transplantation (no) | 4 | Total cholesterol (mg/dL) | 169.2±36.5 | |
CMV a infection (no) | 10 | MMF daily dose f (g) | 2 | |
Polyclonal antibody treatment (no) | 5 | Steroid dose (mg/day) | 5.2±1.3 | |
Type of donor (living/cadaveric) | 36 /4 | Cyclosporine dose (mg/kg) | 1.8±0.4 |
Comparison of demographic characteristics between carriers of UGT1A9 T-275A SNP and Wild type (Data are presented as mean ± SD).
Parameters | Wild type | Carriers | p-value |
---|---|---|---|
Baseline demographics | |||
Number of patients | 34 | 6 | |
Recipient gender (female/male) | 8/26 | 3/3 | |
Body weight (kg) | 70.9 ± 10.9 | 69 ± 14.7 | 0.9 |
Age (yr) | 38.4±11.7 | 46.3±14.6 | 0.2 |
Transplantation-related characteristics | |||
Time after transplantation (d) | 1155.3±1074.4 | 1762.5±958.8 | |
Re-transplantation (no) | 4 | 0 | |
CMVa infection (no) | 40 | 0 | 0.1 |
Polyclonal antibody treatment (no) | 5 | 0 | |
Type of donor (living/cadaveric) | 31/3 | 5/1 | |
Biochemical parameters | |||
Serum creatinine (mg/dL) | 1.2±0.2 | 1.1±0.3 | 0.7 |
GFRb (ml/min/1.73m2) | 83±17.9 | 72.3+13.4 | 0.6 |
Steroid dose (mg/day) | 5.2±1.3 | 5±1.6 | 0.3 |
Cyclosporine dose (mg/kg) | 1.8±0.4 | 1.7±0.2 | 0.8 |
-Validity of the HPLC method: Eleven point calibration curves for MPA on separate days were linear over the concentration range of 0.1-60 μg/mL. The mean regression line showed the correlation coefficient (r2) of 0.9992 with the equation of y = 0.1403x + 0.0002. Absolute analytical recoveries of the assay in four concentrations were between 86±1.4 and 104±1.5% with a mean of 94.8%. The method proved to be accurate and precise; the accuracy at four concentration levels ranged from 91.6-109%. The within-day and between–days precision ranged from 2.1-7.8% and 1.2-19.3%, respectively.
Identification of UGT1A9 polymorphism: In our study population, only 6 patients (15%) were recognized with UGT1A9 T-275A promoter region single nucleotide polymorphism and all of them were heterozygous for this SNP. Among our forty patients only one heterozygous individual carrying UGT1A9*3 mutation was identified (2.5%). Figures 1 and 2 show these identified polymorphisms.
Impact of UGT1A9 T-275A SNP on pharmacokinetic parameters of MPA: Patients with T-275A polymorphism (6 out of 40) did not differ from wild type in terms of age (p=0.19), bodyweight (p=0.95), steroid dose (p=0.32), cyclosporine dose (p=0.78) and CMV infection (p=0.125).
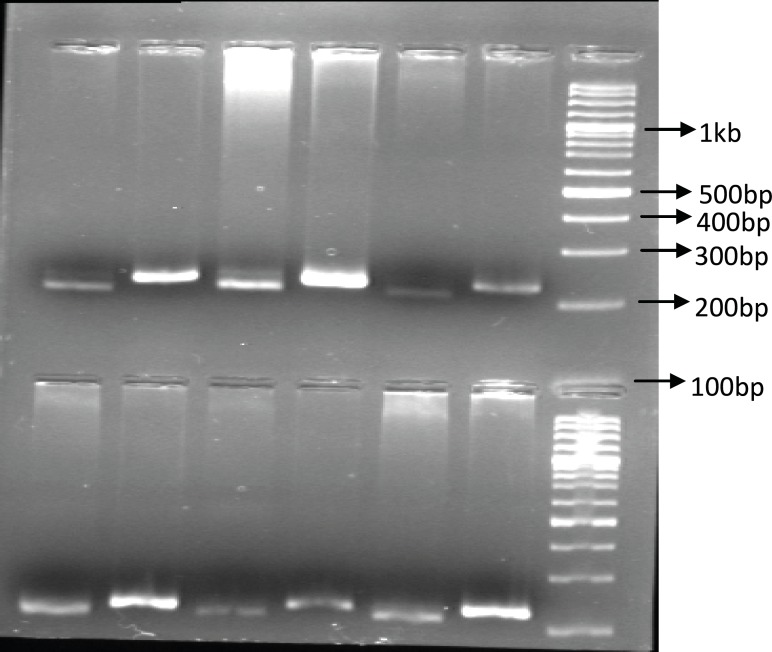
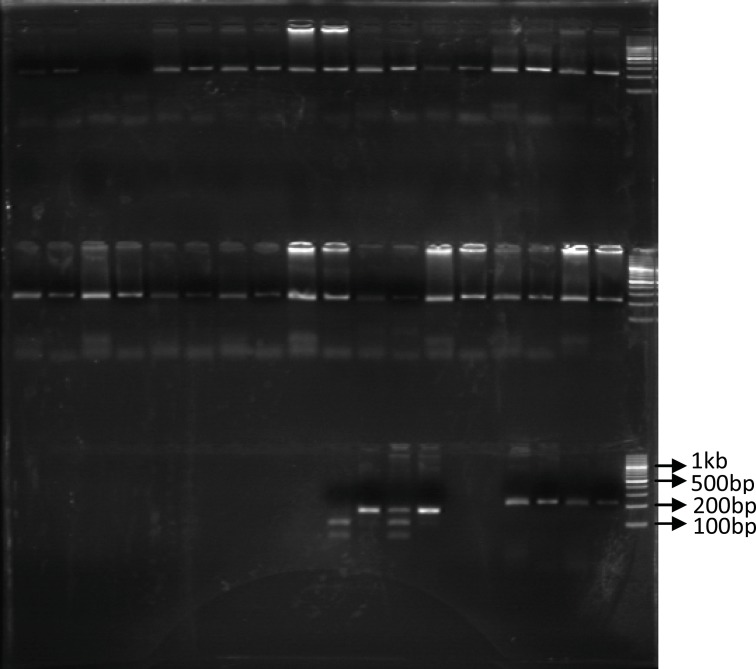
Comparison of MPAa pharmacokinetic parameters between carriers of UGT1A9 (Uridine diphosphate glucuronosyl transferase) T-275A SNPb and noncarriers
Parameters | All | Noncarriers | Carriers | p-value |
---|---|---|---|---|
Number of patients | 40 | 34 | 6 | |
AUC0-12c (mg.h/L) | 104.8±32.3 | 110.4±31.1 | 73.3±17.8* | 0.007 |
AUC6-12d (mg.h/L) | 26.2±9.7 | 26.9±10.2 | 22.4±4.5 | 0.26 |
C0e (mg/L) | 3.8±1.6 | 3.9±1.6 | 3.0±1.2 | 0.11 |
Cmaxf (mg/L) | 34.6±13.4 | 37.2±12.5 | 20.3±9.0* | 0.0002 |
Tmaxg (h) | 1.0±0.5 | 0.9±0.5 | 1.3±0.6 | 0.22 |
CL/F h (L/h) | 19. 7±6.2 | 18.4±5.2 | 26.9±10.2* | 0.002 |
Determinants of MPAa pharmacokinetic parameters (based on ridge regression results).
Dependent variables | Independent variables | r2 | p-value |
---|---|---|---|
MPA AUC0-12b (mg.h/L) | UGT c1A9 T-275A SNP d | 0.60(pos) | 0.007 |
MPA AUC12-6e(mg.h/L) | no covariate retained in the model | ||
C0f (mg /L) | no covariate retained in the model | ||
Cmaxg (mg /L) | UGT1A9 T-275A SNP | 0.68(pos) | 0.0002 |
tmaxh (h) | no covariate retained in the model | ||
CL/F i (L/h) | UGT1A9 T-275A SNP | 0.64(neg) | 0.002 |
According to our results, patients carrying T-275A SNP had significantly lower AUC0-12 for MPA in comparison to the wild type (73.3 ±17.8 mg/h/L versus 110.4±31.1 mg/h/L respectively, p=0.007). The Cmax (maximum plasma concentration) was significantly lower in the presence of T-275A polymorphism (20.3±9.0 mg/L versus 37.2±12.5 mg/L respectively, p=0.0002), though there was no significant difference in C0 between groups (3.0±1.2 mg/L versus 3.9±1.6 mg/L respectively, p =0.11).
Patients with UGT1A9 T-275A SNP had longer Tmax (time to reach maximum plasma concentration) in comparison with patients with wild type UGT1A9, although this difference was not statistically significant (1.3±0.6 h versus 0.9±0.5 h respectively). Moreover, even though AUC6-12 of MPA in carriers of T-275A SNP, was lower than AUC6-12 in wild type group, it was not statistically significant (22.4±4.5 mg/h/L versus 26.8±10.2 mg/h/L respectively, p=0.26). When we compared CL/F (total body clearance) between two groups there was a statistically significant difference between groups in which carriers of UGT1A9 polymorphism had increased clearance compared with wild type patients (26.9±10.2l/h versus 18.4±5.2 L/h respectively, p=0.002).
Impact of UGT1A9*3 mutations on pharmacokinetic parameters of MPA: We had only one heterozygous carrier of UGT1A9*3, therefore statistical analysis of the data was not possible. Pharmacokinetic parameters of this patient were 85.7 mg/h/L; 28.9 mg/h/L; 2.8 mg/L; 21.2 mg/L; 0.7 h; 21.9 L/h for AUC0-12, AUC6-12, C0, Cmax, Tmax and CL/F of MPA respectively. Data are summarized in Table3.
Table 4 shows determinant of MPA pharmacokinetic parameters in the final model. Since there is great interindividual variability in AUC0-12 of MPA among renal transplant recipients (6,10), many experiments have been designed to examine the effect of different factors such as gender, body weight, pathophysiological factors, comedications, race and pharmacogenetics on MPA pharmacokinetics (11-13). The aim of this study was to evaluate the effect of some reported UGT1A9 SNPs on pharmacokinetic parameters of MPA. Although there are many polymorphisms in other UGTs (18, 19) and UGT1A9 enzyme identified by Girard and Kazuyuki (17, 19) none of them has been reported to increase MPA glucuronidation activity to the same extent as the T-275A and C-2152T mutations. In addition, only Kuypres (20) has reported the frequency of T-275 A and C-2152T SNPs in his study, therefore we could assess only T-275A, C-2152T and UGT1A9*3 in our population.
In accordance with Kuypres study, we concluded that patients carrying T-275A SNP had significantly lower MPA AUC0-12 compared with the noncarriers, and 15% frequency of T-275A obtained in this study was approximately similar to reported frequency of 16.8% in Kuypres study (20). While reported frequency of C-2152T was 12.6% in study reported by Kuypers et al. (20), we did not detect this SNP in our population. Lack of detection of some of these mutations has been reported in Japanese and Chinese populations, in whom none of these SNPs were found (13, 22, 23).
In addition to lower MPA AUC0-12, due to higher glucuronidation activity of UGT1A9 in carriers of T-275A mutation, Cmax was significantly lower with no significant difference in C0. Since C0 is not a good predictor of drug efficacy (24), its influence by polymorphism seems to be of less value than other parameters such as AUC and CL/F. We also failed to show the effect of polymorphism on AUC6-12 of MPA as it was reported in Kuypres study (20). The difference between two studies may be related to the type of calcineurin inhibitors received by patients. In Kuypres study patients received tacrolimus which has no effect on enterohepatic recirculation of drug and it only inhibits the glucuronidation of MPA (25-27). On the other hand, our patients received cyclosporine which could interfere with enterohepatic recirculation of drug by the inhibition of MRP-2, and thus diminished the effect of polymorphism on inhibiting enterohepatic recirculation (28-31).
Apparent clearance of drug (CL/F) was significantly higher in T-275A SNP carriers compared to the wild type and there was a positive relationship between the body weight and apparent clearance (r = 0.39, p = 0.01) as reported in other studies (32).
Our patients had higher AUC 0-12 for MPA (104.8±32.3 mg/h/L) compared to other studies. This could be partly because of the effects of other genetic factors such as other concurrent polymorphisms of UGT1A8 and UGT2B7 or SNPs in MRP-2 and non genetic factors such as low steroid dose, ethnicity (33, 34), nutritional habits and concomitant drugs (14). Based on the above results, UGT 1A9 polymorphism can be partly responsible for interindividual differences among the stable renal transplant patients, although most of our patients had acceptable MPA plasma level. Our findings may indicate that routine therapeutic drug monitoring is not necessary in stable renal transplant patients taking MMF.
Conclusion
Polymorphism could have a significant impact on the pharmacokinetics of MPA, in particular the AUC0-12 and Cmax. Both parameters were significantly lower while CL/F was significantly higher in the carriers of UGT1A9 polymorphism. Nevertheless, the small sample size of the patients with the UGT1A9 T-275A promoter region single nucleotide polymorphism should be acknowledged in interpretation of the results obtained in this study. More longitudinal studies with a larger number of patients carrying the desired polymorphism are recommended.
Acknowledgements
References
-
1.
Chandraker A, Iacomini JJ, Sayegh MH. Transplantation Immunobiology. In: Brenner BM, Rector FC, editors. Brenner and Rector’s the Kidney. 8th ed. Philadelphia: Elsevier; 2007. p. 2103-2165.
-
2.
Hamilton CW. Chronic kidney disease. In: Dipiro JT, Talbert RL, Yee GC, Hamilton CW, editors. Pharmacotherapy a Pathophysiologic Approach. 6th ed. United States of America: The McGrawHill companies, Inc; 2005. p. 781-796.
-
3.
Lacy CF, Armstrong LL, Goldman MP, Lance LL. Drug Information Handbook. 13th ed. Ohio: Lexi-Comp Inc; 2007. p. 1038-1041.
-
4.
AHFS Drug Information. Maryland: American Society of Health-System Pharmacists, Inc; 2007. p. 3770-3775.
-
5.
Pou L, Brunet M, Cantarell C, Vidal E, Oppenheimer F, Monforte V, Vilardell J, Roman A, Martorell J, Capdevila L. Mycophenolic acid plasma concentrations: influence of comedication. Ther. Drug. Monit. 2001;23:35-38. [PubMed ID: 11206040].
-
6.
Jeong H, Kaplan B. Therapeutic monitoring of mycophenolate mofetil. Clin. J. Am. Soc. Nephrol. 2007;2:184-191. [PubMed ID: 17699403].
-
7.
Shipkova M, Armstrong VW, Kuypers D, Perner F, Fabrizi V, Holzer H, Wieland E, Oellerich M. MMF Creeping Creatinine Study Group, Effect of cyclosporine withdrawal on mycophenolic acid pharmacokinetics in kidney transplant recipients with deteriorating renal function: preliminary report. Ther. Drug. Monit. 2001;23:717-721. [PubMed ID: 11802109].
-
8.
Naito T, Shinno K, Maeda T, Kagawa Y, Hashimoto H, Otsuka A, Takayama T, Ushiyama T, Suzuki K, Ozono S. Effects of calcineurin inhibitors on pharmacokinetics of mycophenolic acid and its glucuronide metabolite during the maintenance period following renal transplantation. Biol. Pharm. Bull. 2006;29:275-280. [PubMed ID: 16462031].
-
9.
Cattaneo D, Perico N, Gaspari F, Gotti E, Remuzzi G. Glucocorticoids interfere with mycophenolate mofetil bioavailability in kidney transplantation. Kidney Inter. 2002;62:1060-1067.
-
10.
David-Neto E, Pereira LM, Kakehashi E, Sumita NM, Mendes ME, Castro MC, Romano P, Mattos RM, Batista VR, Nahas WC, Ianhez LE. The need of mycophenolic acid monitoring in long-term renal transplants. Clin. Transplant. 2005;19:19-25. [PubMed ID: 15659129].
-
11.
Knight SR, Morris PJ. Does the evidence support the use of mycophenolate mofetil therapeutic drug monitoring in clinical practice? A systematic review. Trans. 2008;85:1675-1685.
-
12.
de Winter BC, Mathôt RA, van Hest RM, van Gelder T. Therapeutic drug monitoring of mycophenolic acid: Does it improve patients outcome? Expert Opin. Drug Metab. Toxicol. 2007;3:251-261.
-
13.
Jiao Z, Ding JJ, Shen J, Liang HQ, Zhong LJ, Wang Y, Zhong MK, Lu WY. Population pharmacokinetic modeling for enterohepatic circulation of mycophenolic acid in healthy Chinese and the influence of polymorphism in UGT1A9. Br. J. Clin. Pharmacol. 2008;65:893-907. [PubMed ID: 18279479].
-
14.
Hesselink DA, Van Gelder T. Genetic and nongenetic determinants of between-patient variability in the pharmacokinetics of mycophenolic acid. Clin. Pharmacol. Ther. 2005;78:317-321. [PubMed ID: 16198650].
-
15.
Bernard O, Guillemette C. The main role of UGT1A9 in the hepatic metabolism of mycophenolic acid and the effects of naturally occurring variants. Drug Metab. Dispos. 2004;32:775-778. [PubMed ID: 15258099].
-
16.
Fisher MB, Paine MF, Strelevitz TJ, Wrighton SA. The role of hepatic and extrahepatic UDP- glucuronosyltransferases in human drug metabolism. Drug Metab. Rev. 2001;33:273-297. [PubMed ID: 11768770].
-
17.
Girard H, Court MH, Bernard O, Fortier LCH, Villeneuve L, Qin H, Greenblatt DJ, Von Moltek LL, Perussed L, Guillemette C. Identification of common polymorphisms in the promoter of the UGT1A9 gene: evidence that UGT1A9 protein and activity levels are strongly genetically controlled in the liver. Pharmacogenetics. 2004;14:501-515. [PubMed ID: 15284532].
-
18.
Glander P, Hambach P, Braun KP, Fritsche L, Giessing M, Mai I, Einecke G, Waiser J, Neumayer HH, Budde K. Pre-transplant inosine monophosphate dehydrogenase activity is associated with clinical outcome after renal transplantation. Am. J. Transplant. 2004;4:2045-2051. [PubMed ID: 15575908].
-
19.
Kazuyuki I, Masatomo M, Shigeru S, Kagaya H, Saito M, Habuchi T, Suzuki T. Influence of UGT1A7 and UGT1A9 intronic I399 genetic polymorphisms on mycophenolic acid pharmacokinetics in Japanese renal transplant recipients. Ther. Drug Monit. 2007;29:299-304. [PubMed ID: 17529886].
-
20.
Kuypers DRJ, Naesens M, Vermeire S, Vanrenterghem Y. The impact of uridine diphosphate-glucuronosyltransferase 1A9 (UGT1A9) gene promoter region single-nucleotide polymorphism T-275A and C-2152T on early mycophenolic acid dose-interval exposure in de novo renal allograft recipients. Clin. Pharmacol. Ther. 2005;78:351-361. [PubMed ID: 16198654].
-
21.
Ahadi Barzoki M, Rouini MR, Gholami KH, Lesan Pezeshki M, Rezaei S. Determination of mycophenolic acid in human plasma by high-performance liquid chromatography. DARU. 2005;13:120-126.
-
22.
Kagaya H, Inoue K, Miura M, Satoh S, Saito M, Tada H, Habuchi T, Suzuki T. Influence of UGT1A8 and UGT2B7 genetic polymorphisms on mycophenolic acid pharmacokinetics in Japanese renal transplant recipients. Eur. J. Clin. Pharmacol. 2007;63:279-288. [PubMed ID: 17211619].
-
23.
Saeki M, Saito Y, Jinno H, Sai K, Ozawa S, Kurose K, Kaniwa N, Komamura K, Kotake T, Morishita H, Kamakura S, Kitakaze M, Tomoike H, Shirao K, Tamura T, Yamamoto N, Kunitoh H, Hamaguchi T, Yoshida T, Kubota K, Ohtsu A, Muto M, Minami H, Saijo N, Kamatani N, Sawada JI. Haplotype structures of the UGT1A gene complex in a Japanese population. Pharmacogenomics. 2006;6:63-75.
-
24.
Kaplan B. Mycophenolic acid trough level monitoring in solid organ transplant recipients treated with mycophenolate mofetil: Association with clinical outcomes. Curr. Med. Res. Opin. 2006;22:2355-2364. [PubMed ID: 17257450].
-
25.
Hubner GI, Eismann R, Sziegoleit W. Drug interaction between mycophenolate mofetil and tacrolimus detectable within therapeutic mycophenolic acid monitoring in renal transplant patients. Ther. Drug Monit. 1999;21:536-539. [PubMed ID: 10519451].
-
26.
Zucker K, Rosen A, Tsaroucha A, de Faria L, Roth D, Ciancio G, Esquenazi V, Burke G, Tzakis A, Miller J. Unexpected augmentation of mycophenolic acid pharmacokinetics in renal transplant patients receiving tacrolimus and mycophenolate mofetil in combination therapy, and analogous in vitro findings. Transpl. Immunol. 1997;5:225-232. [PubMed ID: 9402690].
-
27.
Zucker K, Tsaroucha A, Olson L, Esquenazi V, Tzakis A, Miller J. Evidence that tacrolimus augments the bioavailability of mycophenolate mofetil through the inhibition of mycophenolic acid glucuronidation. Ther. Drug. Monit. 1999;21:35-43. [PubMed ID: 10051052].
-
28.
Kobayashi M, Saitoh H, Tadano K, Tadano K, Takahashi Y, Hirano T. Cyclosporin A, but not tacrolimus, inhibits the biliary excretion of mycophenolic acid glucuronide possibly mediated by multidrug resistance-associ-ated protein 2 in rats. J. Pharmacol. Exp. Ther. 2004;309:1029-1035. [PubMed ID: 14978191].
-
29.
Van Gelder T, Klupp J, Barten MJ, Christians U, Morris RE. Comparison of the effects of tacrolimus and cyclosporine on the pharmacokinetics of mycophenolic acid. Ther. Drug. Monit. 2001;23:119-128. [PubMed ID: 11294511].
-
30.
Deters M, Kirchner G, Koal T, Klaus R, Volkhard K. Influence of cyclosporine on the serum concentration and biliary excretion of mycophenolic acid and 7-O-mycophenolic acid glucuronide. Ther. Drug. Monit. 2005;27:132-138. [PubMed ID: 15795641].
-
31.
Hesselink DA, van Hest RM, Mathot RA, Van Hest RM, Mathot RAA, Bonthuis F, Weimar W, De Bruin RWF, Van Gelder T. Cyclosporine interacts with mycophenolic acid by inhibiting the multidrug resistance-associated protein 2. Am. J. Transplant. 2005;5:987-994. [PubMed ID: 15816878].
-
32.
Staatz CE, Duffull SB, Kiberd B, Fraser AD, Tett SE. Population pharmacokinetics of mycophenolic acid during the first week after transplantation. Eu. J. Clin. Pharmacol. 2005;61:507-516.
-
33.
Neylan JF. Immunosuppressive therapy in high-risk transplant patients: Dose dependent efficacy of mycophenolate mofetil in African-American renal allograft recipients. Transplantation. 1997;64:1277-1282. US Renal Transplant Mycophenolate Mofetil Study Group. [PubMed ID: 9371668].
-
34.
Chen H, Peng C, Yu Z, Shen B, Deng X, Qiu W, Fei Y, Shen C, Zhou G, Yang W, Li H. Pharmacokinetics of mycophenolic acid and determination of area under the curve by abbreviated sampling strategy in Chinese liver transplant recipients. Clin. Pharmacokinet. 2007;46:175-185. [PubMed ID: 17253887].