Abstract
Keywords
Introduction
Diabetes mellitus (DM), a global public health problem, is now emerging as an epidemic worldwide (1). The term diabetes mellitus describes several diseases of abnormal carbohydrate metabolism that are characterized by hyperglycaemia. It is associated with a relative or absolute impairment in insulin secretion, along with varying degrees of peripheral resistance to the action of insulin. Medicinal plants provide valuable therapeutic antidiabetic agents, in traditional and modern medicine. Herbal medications have been used for a variety of ailments and in recent years, the use of natural health products as complementary or alternative approaches to existing medications is growing in popularity (2). Several ethnopharmacologial studies on medicinal plants having beneficial effects on diabetes have been reported (3). The hypoglycaemic activity of a large number of medicinal plants used in traditionally has been evaluated and confirmed in different animal models (4-6).
Garlic (Allium sativum L., Alliaceae) has been used since ancient times as a folk medicine in diabetes in Iran (7-8), India (9), and Europe (10). Persian shallot (Allium ascalonicum L., Alliaceae) is used traditionally as a hypoglycemic agent in Asia (11-12). These plants have been cultivated in all over Iran for their characteristic flavors and medicinal properties (8). Multiple biological effects of garlic and Persian shallot are related to the presence of thiosulfinates, volatile sulfur compounds that are typical of the Allium species, which are also responsible for their characteristic pungent aroma and taste (13). Sage (Salvia officinalis L., Lamiaceae) has wide range of biological activities (14-15) and especially its hypoglycemic effects in Iranian folk medicine due to its flavonoids such as kaempferol, luteolin and quercetin are reported (16-21). Sage antioxidant properties are attributed mainly to the presence of carnosic acid and rosmarinic acid (22).
Garlic, Persian shallot and sage are used in traditional medicine in Iran for diabetes; however, biochemical and molecular basis of their antidiabetic effects remain unclear. The purpose of the present study was to examine the influence of oral administration of garlic and Persian shallot bulbs and sage leaves methanolic extracts ( ASE, AAE and SOE respectively) on the levels of blood glucose, oral glucose tolerance, Insulin and Glut-4 genes expression and intestine alpha glucosidase enzymes activities in alloxan-induced diabetic Wistar rats.
Experimental
Chemicals and reagents
Glibenclamide, metformin and acarbose were purchased from Tehran Chemistry (Iran), alloxan mono hydrate was purchased from Pharmacia & Upjohn (USA), DEPC water, Taq polymerase and RNA extraction kit were obtained from CinnaGen (Tehran, Iran); DNase I, RNase free kit were purchased from Fermentas (Ontario, Canada), RT kit and Primers from Bioneer (Daejon, Korea), dNTPs from BioFlux (Tokyo, Japan). All other chemicals and solvents were of the highest commercial grade from Merck (KGaA, Germany) or from Sigma (St Louis, MO, USA).
Plants materials
Plants materials used in this study consisted of the bulbs of Allium sativum L. (Alliaceae), Allium ascalonicum L. (Alliaceae) and the leaves of Salvia officinalis L. (lamiaceae). Garlic plants were collected from Caspian sea region in the north of Iran. Persian shallots were obtained from Alborz mountain in the north of Tehran and sage plants were collected from northwest of Iran. They were authenticated by Professor Ahmad Qahraman and voucher specimen as follows: Allium satium L., 35842, Allium ascalonicum L.,35351 and Salvia officinalis L.,37221. They were deposited at the herbarium of University of Tehran, Tehran, Iran.
Preparation of Allium sativum L. (ASE), Allium ascalonicum L. (AAE) and Salvia officinalis L. (SOE) methanolic extracts
Dried and ground bulbs of garlic and Persian shallot (about 200 g) and leaves of sage were submitted to extraction with 300 mL methanol (80%) in a Soxhlet apparatus for 72 h (23). After extraction, the solvent was filtered and then evaporated by Rotavapor. The percentage yields based on the dried starting materials were 20% for garlic, 17% for shallot and 23% for sage. The mehtanolic extracts were stored in the dark at 4°C until used for experiments.
Animals
Male Wistar rats, weighing 200–250 g (Pasteur Institute, Tehran, Iran) were used in this study. Animals were housed six per standard rat cage, in a room with a 12:12 h light/dark cycle (lights on 07:00 h) and controlled temperature (22 ± 1 °C). Commercial rodent pellets and tap water were available ad libitum. They were allowed to adapt to the laboratory conditions for one week before beginning of the study. There were six rats per group in each experiment. The procedures were performed in accordance with institutional guidelines for animal care and use.
Preparation of alloxan-induced diabetic Wistar rats
Diabetes was induced in overnight fasted rats by subcutaneous injection of alloxan monohydrate (100 mg kg−1), dissolved in citrate buffer (pH = 4.5), according to a previously described method (24-25). After one week of administration, survived rats with marked hyperglycemia (postprandial blood glucose > 250 mg/dL) were selected and used for this study.
Experimental design
Rats were randomly divided into the following ten groups, each group consisting of six animals.
Group I (NC): Normal rats treated with vehicle alone; Group II (DC): Diabetic rats treated with vehicle alone; Group III (ASEa+D): Diabetic rats treated with ASE at the dose of 250 mg kg−1 BW; Group IV (ASEb+D): Diabetic rats treated with ASE at the dose of 500 mg kg−1 BW; Group V (AAEa+D): Diabetic rats treated with AAE at the dose of 250 mg kg−1 BW; Group VI (AAEb+D): Diabetic rats treated with AAE at the dose of 500 mg kg−1 BW; Group VII (SOEa+D): Diabetic rats treated with SOE at the dose of 250 mg kg−1 BW; Group VIII: (SOEb+D): Diabetic rats treated with SOE at the dose of 500 mg kg−1 BW; Group IX: (Ac+D): Diabetic rats treated with acarbose (20 mg kg−1 BW) in the 1st phase; Group X: (Glib+D in the1st phase or Met+D in the 2nd phase): Diabetic rats treated with glibenclamide (5 mg kg−1 BW) or metformin (100 mg kg−1 BW).
In the first phase of this study, a single dose of each sample was administered to all rats and postprandial blood glucose levels (blood glucose level after eating a meal) were estimated in a short-term model. In this phase acarbose and glibenclamide were used as the reference drugs. After two days, Oral Glucose Tolerance Test (OGTT) was carried out for all rats and acarbose was used as the reference drug.
In the second phase of this study, two days after OGTT, one dose of samples was administered daily for 21 days (26) to all rats. In this phase metformin was used as the reference drug. Postprandial blood glucose levels were estimated in a long-term model. At the end of 21 days of treatment, rats were anesthetized by ether, then the animals were killed and the pancreases and hearts of NC, DC, ASEb+D, AAEb+D and SOEa+D groups were removed promptly for the estimation of insulin (Ins) and glucose transporter-4 (Glut-4) mRNA expression. In a separate experiment, the inhibitory effects of plants extracts on intestinal α-glucosidases (sucrase and maltase) were measured by an in-vitro method.
Oral administration of the plant extracts
1mL of each plant extract sample was administered orally at 11-12 a.m. using an intragastric tube.
Estimation of hypoglycemic activity
Short-term experimental model
Blood samples were obtained from the tail vein and postprandial plasma glucose levels were estimated using a glucometer (On Call Now, San Diego, USA) after 1, 3, 5, 8 and 24 h following administration of a single dose of samples to rats. The NC and DC groups were treated by the same volume of vehicle (1 mL of distilled water).
Long-term experimental model
During the long-term treatment period with ASE (250 and 500 mg kg−1 BW), AAE (250 and 500 mg kg−1 BW), SOE (250 mg kg−1 BW) and metformin (100 mg kg−1 BW), the levels of postprandial plasma glucose in all rats were estimated at the end of 1, 2 and 3 weeks of treatments using blood samples obtained from tail vein and a glucometer device (On Call Now, San Diego, USA).
Measurement of ASE, AAE and SOE effects on Oral Glucose Tolerance
NC and DC Groups received orally distilled water. ASEa+D and ASEb+D groups received orally ASE at doses of 250 and 500 mg kg−1 BW respectively. AAEa+D and AAEb+D groups received orally AAE at doses of 250 and 500 mg kg−1 BW respectively. SOEa+D group received orally SOE at dose of 250 mg kg−1 BW. To group Ac+D, the reference drug acarbose (20 mg kg−1 BW) was administrated orally. Thirty minutes later, a carbohydrate solution (equal proportion of maltose and sucrose 2 g kg−1 BW) was administered orally to each rat (27). PBG was determined at 0 min, just before carbohydrate solution loading, and at 30, 60 and 120 min after carbohydrate solution loading, using a glucometer (On Call Now, San Diego, USA).
Gene expression analysis
To investigate the mechanism of ASE, AAE and SOE antihyperglycemic action, at the end of 21 days of treatment, the animals in DC, NC, ASEb+D, AAEb+D and SOEa+D groups were analyzed for Ins and Glut-4 mRNAs expression by RT-PCR (Reverse Transcription Polymerase Chain Reaction). β-actin gene was used as internal control. Total RNA of pancreas and heart tissues (28) of each rat was extracted by RNX-Plus kit. In brief, after homogenization of tissue samples (1 mL per 50-100 mg tissue) with RNX-Plus kit, proteins were extracted with chloroform and total RNA was precipitated with isopropanol. The precipitated RNA was washed with 70% ethanol and resuspended in 50 μL of DEPC-treated water. Finally the DNA free RNA was prepared prior to RT-PCR using DNase I, RNase-free kit. Reverse transcription was carried out to obtain cDNA using AccuPower RT PreMix kit, 50 ng/μL template RNA and 25 ng/μL oligo dT18. The primers used were as follows: Ins F, 5′-TTC TTC TAC ACA CCC AAG-3′; Ins R, 5′-GCA GTA GTT CTC CAG TTG-3′ (155-bp); Glut-4 F, 5′-AGG CAC CCT TAC CCT TTT-3′; Glut-4 R 5′-GAC AGA AGG GCA ACA GAA GC-3′ (318-bp) and β-act F, 5′-AGC CAT GTA CGT AGC CAT CC-3′; β-act R,5′-TCT CAG CTG TGG TGG TGA AG-3′ (248-bp). For PCR reaction, 500 ng of the cDNA was added to a PCR reaction mixture consisting of 10XPCR buffer (2.5 μL), 50 mM MgCl2 (0.75 μL), 10 mM dNTPs (0.5 μL), 10 pM of paired primers (0.5 μL of each), 0.25 units of Taq polymerase and distilled water in a total volume of 25 μL. The reaction mixture was loaded in a PCR thermal cycler for 35 cyclic reactions. PCR products were run on 1.5% agarose gels, stained with ethidium bromide and photographed. Images of radiographs were analyzed with TotalLab v1.10 using 1D analysis.
Inhibition assay for rat intestinal sucrase activity
Inhibition of rat intestinal sucrase was assayed using previously reportedmethod (29-30) with a slight modification. 0.2 mL of 56 mM sucrose, as the enzyme substrate, in 0.1 M potassium phosphate buffer (pH 7, 0.2 mL) was mixed with 0.1 mL of the plant extracts in 50% aqueous dimethyl sulfoxide (DMSO). After pre-incubation at 37° C for 5 min, 0.2 mL of rat intestinal α-glucosidase solution prepared from intestine of normal rats (31) was added. Instead of the plant extract, 0.1 mL DMSO was used for the blank sample. After mixing thoroughly, both samples and blank test tubes were incubated at 37 °C for 15 min and then the reaction was stopped by submerging test tubes in boiling water for 4 min. The reaction mixture was passed through a basic alumina column (6 mm × 35 mm h) to eliminate phenolic or acidic compounds. The amount of liberated glucose was determined by the glucose oxidase assay using a commercial test kit. The optical density (OD) of the wells was measured at 505 nm and the inhibitory activities of plants extracts were calculated using following formula:
Inhibitory activity (%) = 100 (1-[ODtest sample/ODcontrol] )
Inhibition assay for rat intestinal maltase activity
Inhibition of rat intestinal maltase was determined by using reported method (29, 32) with a slight modification. The assay was carried out in the same manner as the inhibition assay for rat intestinal sucrase, except for using 3.5 mM maltose, as the enzyme substrate, in 0.1 M potassium phosphate buffer (pH 7, 0.35 mL).
Statistical analysis
All data are presented as mean ± SD. for six animals in each group. Comparisons between groups and between time points were made by one-way analysis of variance (ANOVA) followed by Duncan’s test to analyze the difference. Differences were considered significant when P-values were less than 0.05. All statistical analyses were performed using SPSS (SPSS Inc, Chicago, USA).
Results
Effects of ASE, AAE and SOE on PBG in short term treatment
Table 1 depicts antihyperglycemic effects of two different doses of ASE, AAE and SOE at different intervals of time after a single dose oral administration of each extract, acarbose (20 mg kg−1 BW) and glibenclamide (5 mg kg−1 BW) as reference drugs.
Acute effects of ASE, AAE and SOE on postprandial blood glucose in alloxan-diabetic Wistar rats
Groups | Dose | Postprandial blood glucose (mg/dL) | |||||
---|---|---|---|---|---|---|---|
(mg kg−1BW) | |||||||
0 | 1 | 3 | 5 | 8 | 24 | ||
NC | - | 68.2±2.3b | 71.2±6.4b | 64.8±2.8b | 74.4±3.3b | 75.2±4.5b | 70.34±4.4b |
DC | - | 314.2±10.3c,d | 318.7±14.4d | 309.34±15.2 | 303.22±8.2 | 312.32±12.3 | 322.21±10.3 |
ASEa+D | 250 | 309.53±15.7c,d,e | 307.45±11.4d,e | 289.41±9.4a,b,d | 281.62±12.3a,b | 274.82±8.3a,b | 282.65±9.1a,b |
ASEb+D | 500 | 311.4±12.5c,d,e | 309.61±8.9d,e | 287.34±11.8a,b,d | 259.3±12.6a,b,d | 246.52±9.6a,b | 241.66±9.2a,b |
AAEa+D | 250 | 308.44±14.4c,d,e | 316.4±13.1d,e | 311.64±8.2e | 285.53±10.3a,b | 287.39±8.51a,b | 283.3±14.2a,b |
AAEb+D | 500 | 311.24±13.2c,d,e | 304.81±7.1d,e | 291.5±8.9a,b,d | 273.24±11.7a,b | 270.41±10.7a,b | 274.23±7.4a,b,c |
SOEa+D | 250 | 314.67±11.1c,d,e | 289.6±10a,b | 269.33±9.2a,b,c | 290.33±15.5a | 292.3±9.2a,b | 271±11.5a,b,c |
SOEb+D | 500 | 320.66±19.5c,d,e | 318.66±14d,e | 298.33±13.5a,d,e | 310.5±9e | 320±15.13e | 324.3±14.2e |
Ac+D | 20 | 318.2±10.2c,d,e | 265.2±7a,b | 256.2±7.3a,b | 186.2±14.8a,b | 199.4±7a,b | 266.8±7.8a,b |
Glib+D | 5 | 312.4±14.3c,d,e | 303.11±7.9e | 291.6±12.8a,b | 245.73±9.1a,b | 174.32±9.6a,b | 129.44±8.2a,b |
ASE (250 and 500 mg kg−1 BW ) and AAE (500 mg kg−1 BW) at 3h reduced significantly PBG, 6%, 7% and 5.7% respectively (p < 0.05 vs. DC) and decreased gradually PBG till 24 h (p < 0.05 vs. DC), similar to glibenclamide. SOE (250 mg kg−1 BW) reduced significantly PBG at 1 h 9% and 3h 13% (p < 0.05 as compared with DC). Then gradual rise occurred in PBG till 8 h. Moreover, at 24 h, PBG is reduced 14% (p < 0.05 as compared with Time 0). The effects of SOE (250 mg kg−1 BW) at 3h and 24h were similar to that of acarbose (p > 0.1). SOE (500 mgkg−1 BW) reduced significantly PBG (p < 0.05 as compared with Time 0) at 3h but it showed no notable difference when compared with DC (p > 0.06), thus this dose was eliminated in following experiments. At 24 h, ASE (500 mg kg−1 BW) and glibenclamide (5 mg kg−1 BW) produced major fall in PBG 25% and 60% (p < 0.05 vs. DC) respectively, but AAE (500 mg kg−1 BW) and acarbose (20 mg kg−1 BW) showed noticeable decrease in PBG at 8 h, 13% and 36% (p < 0.05 vs. DC) respectively.
Effects of ASE, AAE and SOE on oral Glucose tolerance
Table 2 shows that PBG concentrations increased 30 min after oral administration of carbohydrate solution, in all groups and decreased subsequently. The increase in PBG levels, compared to DC group, diminished significantly in ASEa and ASEb groups at 30 min by 10% and 9%, at 60 min by 9 % and 7% and at 120 min by 11% and 13% and in AAEa and AAEb groups at 30 min by 12 % and 11 %, at 60 min by 6 % and 8 % and at 120 min by 9 % and 12 % (p < 0.05). SOEa group showed diminution in PBG levels at 30 min by 17 %, at 60 min by 32 % and at 120 min by 13 % (p < 0.05). These results show that the reduction of PBG by SOE at dose of 250 mg/kg BW, at 60 min was 32 % (p < 0.05) and this effect is comparable with 36 % reduction of PBG by acarbose (p > 0.1).
Effects of ASE , AAE and SOE on oral glucose tolerance test in alloxan-diabetic rats. The plants extracts and the reference drug was administered to 16 h fasted rats, and 30 min later all groups received a carbohydrate solution (maltose and sucrose each 1 g/kg BW) by gastric intubations. Postprandial blood glucose was monitored at 0, 30, 60 and 120 min after carbohydrate solution administration
Groups | Dose | Blood glucose (mg/dL) | |||
---|---|---|---|---|---|
minute after a single dose administration of extracts and drug | |||||
0 | 30 | 60 | 120 | ||
NC | - | 70.32±3.2b | 139.23±7.3a,b | 119.38±5.1a,b | 87.76±4.3a,b |
DC | - | 186.74±7.4 | 329.56±14.8a | 288.72±11.7a | 254.68±13.3a |
ASEa+D | 250 | 189.72±15.5 | 295.41±9.1a,b | 261.8±7.9a,b | 225.72±10.3a,b |
ASEb+D | 500 | 194.37±13.2 | 298.63±8.2a,b | 267.73±12.2a,b | 219.86±14.1a,b |
AAEa+D | 250 | 192.25±10.1 | 289.92±13a,b | 270.43±8.2a,b | 231.3±11.6a,b |
AAEb+D | 500 | 189.44±8.7 | 292.56±12.6a,b | 263.31±9.2a,b | 223.85±6.8a,b |
SOEa+D | 250 | 173.8±13.7c | 271.82±14.2a,b | 194.42±12.5a,b,c | 220.4±11.7a,b |
Ac+D | 20 | 161.43 ± 7.6b | 244.54 ± 10.8a,b | 183.34 ±7.6a,b | 165.82 ± 6.5b |
Effects of ASE, AAE and SOE on PBG in long term treatment
The levels of PBG in alloxan-diabetic rats are given in Table 3. Daily administration of methanolic plants extracts reduced PBG concentration in all groups at the end of first, second and third weeks. PBG concentrations decreased 37 % in ASEa+D, 36 % in ASEb+D, 13 % in AAEa +D, 22 % AAEb +D and 32 % in SOEa+D groups, (p < 0.05 vs. time 0). Moreover in Met+D group PBG levels dropped off by 37 % (p < 0.05 vs.time0). After 3 weeks of treatment, the hypoglycemic outcome of ASEa+D, ASEb+D and SOEa+D show similar effects to that of Met+D group (p > 0. 1). These results indicate that after long term treatment, all groups showed significant effects in reducing PBG levels comparing to DC group (p < 0.05).
Glycemic control by ASE, AAE and SOE in alloxan-diabetic rats after 3 weeks treatment. PBG was measured at the end of first, second and third weeks of the experimental period.
Groups | Dose | Postprandial blood glucose (mg/dL) | |||
---|---|---|---|---|---|
Days after a single dose daily treatment | |||||
0 | 7 | 14 | 21 | ||
NC | - | 79.33±16.19b | 85.5±5.26b | 70.5±8.7b | 75±5.16b |
DC | - | 303.23±12.7 | 309.31±14.2 | 317.33±8.8 | 324.62±12.3a |
ASEa+D | 250 | 287.25±7.8b | 234.76±6.3a,b,c | 207.11±10.2a,b,c | 182.67±6.4a,b,c |
ASEb+D | 500 | 294.56±8.4 | 239.52±7.5a,b,c | 204.73±9.2a,b,c | 186.65±8.2a,b,c |
AAEa+D | 250 | 291.32±11.6 | 276.51±9.7a,b | 255.2±8.4a,b | 253.74±10.2a,b |
AAEb+D | 500 | 284.72±9.2b | 254.2±6.2a,b,c | 215.65±8.9a,b,c | 221.42±5.3a,b |
SOEa+D | 250 | 294.67±11 | 239.1±8.7a,b,c | 228.31±15.6a,b,c | 200.84±10.5a,b,c |
SOEb+D | 500 | - | - | - | - |
Met+D | 20 | 308.28 ± 10.2 | 257.3 ± 7.9a,b | 218.4 ± 9.3a,b | 195.4 ± 6.2a,b |
Effects of ASE, AAE and SOE on Ins and Glut-4 genes expression
Gel electrophoresis images reveal elevated expression of both Ins (Figure 1A) and Glut-4 (Figure 1B) transcripts by ASE (500 mg kg−1 BW), AAE (500 mg kg−1 BW) and SOE (250 mg kg−1 BW). Also, densitometric scanning reveals increase in Ins and Glut-4 genes transcripts by 0.57-fold (p < 0. 05) and 1.21-fold (p < 0.05) by ASE, 0.31 fold (p < 0.05) and 0.71-fold (p < 0.05) by AAE and 0.19-fold (p < 0.05) and 1.05-fold (p < 0.05) by SOE respectively, as compared to DC group. In these assays, β-actin gene was used as internal control.
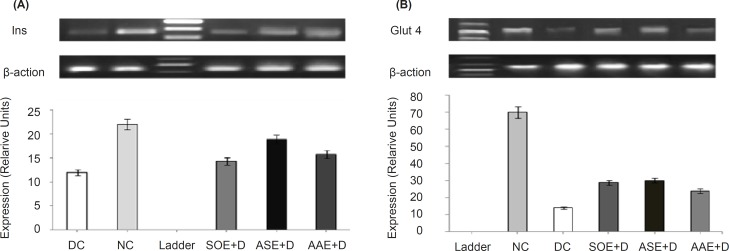
Effect of ASE, AAE and SOE on rat intestinal sucrase and maltase activities
The methanolic extract of Salvia officinalis L. leaves showed strong inhibitory activity for both sucrase and maltase by 48.05 % (±2.87) and 49.21 % (±2.28) respectively. Methanolic extracts of Allium sativum and Allium ascalonicum bulbs exhibited inhibitory activities for sucrase in order of, 24.11 %( ±1.45) and 17.41 %( ±2.81) and for maltase in order of, 19.37 % (±1.81) and 14.62 % (±2.12).
Discussion
The stimulation of experimental diabetes in rat, using chemicals that selectively destroy pancreatic β-cells is very convenient and simple to use. The most usual substances to induce diabetes in rats are alloxan monohydrate and streptozotocin. The effects of alloxan on pancreatic β-cells consist of several processes such as oxidation of essential-SH groups, inhibition of glucokinase, generation of free radicals and disturbances in intracellular calcium homeostasis (33). Alloxan has been observed to cause a massive reduction of the β-cells of the islets of Langerhans and induce hyperglycemia (34). In this study, we have observed that Allium sativum, Allium ascalonicum and Salvia officinalis methanolic extracts decrease blood glucose level in alloxan diabetic rats. The possible mechanism of action of plants extracts could be correlated with the plasma insulin levels (35-36).
It is generally accepted that glibenclamide (a sulfonylurea family drug) causes reduction of blood glucose predominantly via stimulation of insulin release from pancreatic β-cells. Additionally, during long-term treatment, an insulin-independent blood glucose-decreasing mechanism may operate (37). Acarbose, an alpha-D-glucosidase inhibitor (38-39), reversibly inhibits alpha-glucosidases that exist in the brush-border of the small intestinal mucosa (40). Acarbose can inhibit maltose absorption through the inhibition of maltase and alpha-amylase (41).
The present study demonstrates that in short term model, oral administration of ASE and AAE have hypoglycemic action like glibenclamide while SOE acts like acarbose. Previous studies reveal that ASE and its sulfide components like diallyl trisulfide and allicin increase plasma insulin level and insulin sensitivity (42-43). Moreover, total content of phenolic and diallyl disulfide compounds in AAE are higher than in ASE (44). So the hypoglycemic effects of ASE and AAE may be predominantly due to increase in insulin secretion or (and) insulin sensitivity. Our results show that ASE and AAE have inhibitory effects on intestinal maltase and sucrase . This α-glucosidase inhibition property have important role in antihyperglycemic action of ASE and AAE.
In a dose dependent manner, similar to acarbose, SOE reduced PBG in short term period. Our results show that SOE had strong inhibitory effects on intestinal sucrase and maltase activities. It is known that rosmarinic acid and other phenolic components of Salvia officinalis have α-glucosidase inhibitiory activity (45).
Metformin, a biguanide, which is considered as an insulin sensitizer (46), reduces fasting plasma glucose concentrations by reducing rates of hepatic glucose production (47). Based on our results, in the long-term period, ASE and SOE reduce PBG similar to meformin. In addition to other possible hypoglycemic mechanisms, flavonoids may play important roles in reducing PBG. Some flavonoids such as quercetin and catechin can inhibit glucokinase and glucose 6-phosphatase, the enzymes that control gluconeogenesis (48), and increase the storage of glucose in the liver with reduce glycogen breakdown (49). ASE and AAE are rich in flavonoids such as quercetin 3,4-diglucoside and quercetin 4-glucoside (50). SOE is rich in kaempferol, luteolin and quercetin (17). Additionally, essential oil from sage increases hepatocyte sensitivity to insulin and inhibits gluconeogenesis (51). Altogther, in long-term treatment, ASE and SOE may act by similar hypoglycemic mechanisms as metformin.
OGTT results indicate that the control of PBG level by SOE is possibly mediated by the regulation of glucose uptake from the intestinal lumen, through the inhibition of carbohydrate digestion or absorption (52), resembling the acarbose effect. In order to support this suggestion, our results show that SOE has a strong inhibitory effect on both sucrase and maltase activities. Also, ASE and AAE inhibitory effects on sucrase and maltase activities may be responsible for the control of PBG level.
A primary metabolic effect of insulin is to stimulate the uptake of circulating glucose into muscle and adipose tissue. Conceptually, insulin-mediated glucose transport could occur by either stimulating the activity of existing cell surface glucose transport proteins or by translocation of an intra-cellular transporter to the cell surface in response to insulin. We now know that insulin-stimulated glucose uptake is mediated by the muscle and fat specific, insulin regulatable glucose transporter iso-type 4 (Glut4). Our results demonstrate that ASE, AAE and SOE enhance Ins and Glut-4 mRNA levels in heart muscle. Enhancement in Ins transcripts may be related to the stimulation of the surviving beta cells. Up regulation of Glut-4 expression, an insulin-dependent GLUT, might be an effect of Ins improvement and could cause upgrading in the utilization of blood glucose by muscles. In according to our results, enhancement in expression of Ins and Glut-4 genes may mediate hypoglycemic effects of the plants extracts. Our study is the first to report the up-regulation of Ins and Glut-4 genes expression by the ASE, AAE and SOE in diabetic Wistar rats. More researches are needed to prove the effects of these plants extracts on the enhancement of Ins and Glut-4 genes expression.
In conclusion, in addition to the different mechanisms by which the methanolic extracts of Allium sativum, Allium ascalonicum and Salvia officinalis exert their antihyperglycemic actions, up regulation of Ins and Glut-4 genes expression and inhibition of α-glucosidases activities may play a considerable role in this process.
Acknowledgements
References
-
1.
Wild S, Roglic G, Green A, Sicree R, King H. Global prevalence of diabetes - Estimates for the year 2000 and projections for 2030. Diabetes Care. 2004;27:1047-53. [PubMed ID: 15111519].
-
2.
Allen R, Cushman LF, Morris S, Feldman J, Wade C, McMahon D, Moses M, Kronenberg F. Use of complementary and alternative medicine among Dominican emergency department patients. Am. J. Emerg Med. 2000;18:51-4. [PubMed ID: 10674532].
-
3.
Alarcon-Aguilara FJ, Roman-Ramos R, Perez-Gutierrez S, Aguilar-Contreras A, Contreras-Weber CC, Flores-Saenz JL. Study of the anti-hyperglycemic effect of plants used as antidiabetics. J. Ethnopharmacol. 1998;61:101-10. [PubMed ID: 9683340].
-
4.
Farjou IB, Al-Ani M, Guirgues SY. Lowering of blood glucose in diabetic rabbits by Artemisia extract. J. Faculty Medicine Baghdad. 1987;92:137-141.
-
5.
Jouad, H, Haloui M, Rhiouani H, El Hilaly J, Eddouks M. Ethnobotanical survey of medicinal plants used for the treatment of diabetes, cardiac and renal diseases in the north centre region of Morocco (Fez-Boulemane). Journal of Ethnopharmacology. 1987;77:175-182. [PubMed ID: 11535361].
-
6.
Monavar Feshani A, Monatasser Kouhsari S, Mohammadi S. Vaccinium arctostaphylos, a common herbal medicine in Iran: Molecular andbiochemical study of its antidiabetic effects on alloxan-diabeticWistar rats. J. Ethnopharmacology. 2011;133:67-74.
-
7.
Bailey cj, Day c. Traditional plant medicines as treatments for diabetes. Diabetes Care. 1989;12:553-64. [PubMed ID: 2673695].
-
8.
Zargari A. Medicinal plant. Tehran: Tehran University Press; 1997. p. 59-64.
-
9.
Grover JK, Yadav S, Vats V. Medicinal plants of India with anti-diabetic potential. J. Ethnopharmacol. 2002;81:81-100. [PubMed ID: 12020931].
-
10.
Mathew PT, Augusti KT. Studies on the effect of allicin (diallyl disulphide-oxide) on alloxan diabetes. I. Hypoglycaemic action and enhancement of serum insulin effect and glycogen synthesis. Indian J. Biochem Biophys. 1973;10:209-12. [PubMed ID: 4792931].
-
11.
Tan HTW. Herbs and spices of Thailand. Singapore: Times Editions Marshall Cavendish; 2005. 21 p.
-
12.
Jalal R, Bagheri SM, Moghimi A, Rasuli MB. Hypoglycemic effect of aqueous shallot and garlic extracts in rats with fructose-induced insulin resistance. J. Clin. Biochem. Nutr. 2007;3:218-23. [PubMed ID: 18299719].
-
13.
Lanzotti V. The analysis of onion and garlic. J. Chromatogr A. 2006;1112:3-22. [PubMed ID: 16388813].
-
14.
Farag RS, Badei AZMA, M HF, El-Baroty GSA. Antioxidant activity of some spices essencial oils on linoleic acid oxidation in aqueous media. J. American Oil Chemists Society. 1989;66:792-9.
-
15.
Omidbeygi R. Usage and production of medical plant. iran: Tarahan Nashre Press; 1997. p. 210-216.
-
16.
Zargari A. Medicinal plant. Tehran: Tehran University Press; 1997. p. 59-64.
-
17.
Wang MF, Kikuzaki H, Zhu NQ, Sang SM, Nakatani N, Ho CT. Isolation and structural elucidation of two new glycosides from sage (Salvia officinalis L.). J. Agr. Food Chem. 2000;48:235-8. [PubMed ID: 10691621].
-
18.
Bnouham M, Mekhfi H, Legssyer A, Ziyyat A. Medicinal plants used in the treatment of diabetes in Morocco. Int. J. Diabetes & Metabolism. 2002;10:33-50.
-
19.
Eidi M, Eidi A, Zamanizadeh H. Effect of Salvia officinalis L.leaves on serum glucose and insulin in healthy and streptozotocin-induced diabetic rats. J. Ethnopharmacol. 2005;100:310-3. [PubMed ID: 16125023].
-
20.
Alarcon-Aguilar FJ, Roman-Ramos R, Flores-Saenz JL, Aguirre-Garcia F. Investigation on the hypoglycaemic effects of extracts of four Mexican medicinal plants in normal and alloxan-diabetic mice. Phytotherapy Research. 2002;16:383-6. [PubMed ID: 12112298].
-
21.
Naghibi F, Mosaddegh M, Mohammadi Motamed S, Ghorbani A. Labiatae Family in folk Medicine in Iran: from Ethnobotany to Pharmacology. Iran. J. Pharm. Res. 2005;2:63-79.
-
22.
Cuvelier M, Richard H, Berset C. Antioxidative activity and phenolic composition of pilot-plant and commercial extracts of sage and rosemary. J. American Oil Chemists’ Society. 1996;73:645-52.
-
23.
Eidi M, Eidi A, Bahar M. Effects of Salvia officinalis L. (sage) leaves on memory retention and its interaction with the cholinergic system in rats. Nutrition. 2006;22:321-326. [PubMed ID: 16500558].
-
24.
Kameswara Rao B, Kesavulu MM, Giri R, Appa Rao C. Antidiabetic and hypolipidemic effects of Momordica cymbalaria Hook. fruit powder in alloxan-diabetic rats. J. Ethnopharmacology. 1999;67:103-9.
-
25.
Kumar S, Kumar D, Deshmukh RR, Lokhande PD, More SN, Rangari VD. Antidiabetic potential of Phyllanthus reticulatus in alloxan-induced diabetic mice. Fitoterapia. 2008;79:21-3. [PubMed ID: 17855019].
-
26.
Tang LQ, Wei W, Chen LM, Liu S. Effects of berberine on diabetes induced by alloxan and a high-fat/high-cholesterol diet in rats. J. Ethnopharmacology. 2006;108:109-15.
-
27.
Ortiz-Andrade RR, García-Jiménez S, Castillo-España P, Ramírez-Ávila G, Villalobos-Molina R, Estrada-Soto S. α-Glucosidase inhibitory activity of the methanolic extract from Tournefortia hartwegiana: an anti-hyperglycemic agent. J. Ethnopharmacology. 2007;109:48-53.
-
28.
Huang T, Peng G, Kota B, Li G, Yamahara J, Roufogalis B, Li Y. Anti-diabetic action of punica granatum flower extract: Activation of PPAR-gamma and identification of an active component. Toxicology and Applied Pharmacology. 2005;207:160-169. [PubMed ID: 16102567].
-
29.
Bhandaria MR, Jong-Anurakkuna N, Honga G, Kawabata J. α-Glucosidase and α-amylase inhibitory activities of Nepalese medicinal herb Pakhanbhed (Bergenia ciliata, Haw). Food Chemistry. 2008;106:247-52.
-
30.
Nishioka T, Kawabata J, Aoyama Y. Baicalein, an alpha-glucosidase inhibitor from Scutellaria baicalensis. J. Nat. Prod. 1998;61:1413-5. [PubMed ID: 9834167].
-
31.
Kessler M, Acuto O, Storelli C, Murer H, Müller M, Semenza G. A modified procedure for the rapid preparation of efficiently transporting vesicles from small intestinal brush border membranes. Their use in investigating some properties of D-glucose and choline transport systems. Biochimica et Biophysica Acta (BBA). Biomembranes. 1978;506:136-54.
-
32.
Toda M, Kawabata J, Kasai T. α-Glucosidase inhibitor from Clove (Syzgium aromaticum). Bioscience, Biotechnology, and Biochemistry. 2000;64:294-8.
-
33.
Szkudelski T. The mechanism of alloxan and streptozotocin action in B cells of the rat pancreas. Physiol Res. 2001;50:537-46. [PubMed ID: 11829314].
-
34.
Yamamoto H, Uchigata Y, Okamoto H. Streptozotocin and alloxan induce DNA strand breaks and poly(ADP-ribose) synthetase in pancreatic islets. Nature. 1981;294:284-6. [PubMed ID: 6272129].
-
35.
Latha M, Pari L. Antihyperglycaemic effect of Cassia auriculata in experimental diabetes and its effects on key metabolic enzymes involved in carbohydrate metabolism. Clin Exp. Pharmacol P. 2003;30:38-43.
-
36.
Venkateswaran S, Pari L. Effect of Coccinia indica on blood glucose, insulin and key hepatic enzymes in experimental diabetes. Pharm. Biol. 2002;40:165-70.
-
37.
Lebovitz H. cartographer Oral hypoglycemic agents. New York: Elsevier; 1990. p. 554-574.
-
38.
Coniff R, Krol A. Acarbose: A review of US clinical experience. Clin. Ther. 1997;19:16-26. [PubMed ID: 9083705].
-
39.
Jünger C, Rathmann W, Giani G. [Prescribing behavior of primary care physicians in diabetes therapy: effect of drug budgeting]. Dtsch Med Wochenschr. 2000;125:103-9. [PubMed ID: 10705882].
-
40.
Clissold SP, Edwards C Acarbose. A preliminary review of its pharmacodynamic and pharmacokinetic properties and therapeutic potential. Drugs. 1988;35:214-43. [PubMed ID: 3286212].
-
41.
Luo H, Wang LF, Imoto T, Hiji Y. Inhibitory effect and mechanism of acarbose combined with gymnemic acid on maltose absorption in rat intestine. World J. Gastroenterol. 2001;7:9-15. [PubMed ID: 11819725].
-
42.
Augusti KT, Sheela CG. Antiperoxide effect of S-allyl cysteine sulfoxide, an insulin secretagogue, in diabetic rats. Experientia. 1996;52:115-20. [PubMed ID: 8608811].
-
43.
Liu CT, Hse H, Lii CK, Chen PS, Sheen LY. Effects of garlic oil and diallyl trisulfide on glycemic control in diabetic rats. Eur. J. Pharmacol. 2005;516:165-73. [PubMed ID: 15936752].
-
44.
Leelarungrayub N, Rattanapanone V, Chanarat N, Gebicki JM. Quantitative evaluation of the antioxidant properties of garlic and shallot preparations. Nutrition. 2006;22:266-74. [PubMed ID: 16500553].
-
45.
Kwon YI, Vattem DA, Shetty K. Evaluation of clonal herbs of Lamiaceae species for management of diabetes and hypertension. Asia Pac. J. Clin. Nutr. 2006;15:107-18. [PubMed ID: 16500886].
-
46.
Hundal RS, Inzucchi SE. Metformin - New understandings, new uses. Drugs. 2003;63:1879-94. [PubMed ID: 12930161].
-
47.
Cusi K, DeFronzo RA. Metformin: a review of its metabolic effects. Diabetes Rev. 1998;6:89-131.
-
48.
Gasparin FRS, Spitzner FL, Ishii-Iwamoto EL, Bracht A, Constantin J. Actions of quercetin on gluconeogenesis and glycolysis in rat liver. Xenobiotica. 2003;33:903-11. [PubMed ID: 14514440].
-
49.
Valsa AK, Sudheesh S, Vijayalakshmi NR. Effect of catechin on carbohydrate metabolism. Indian J. Biochem. Bio. 1997;34:406-8.
-
50.
Bonaccorsi P, Caristi C, Gargiulli C, Leuzzi U. Flavonol glucosides in Allium species: A comparative study by means of HPLC-DAD-ESI-MS-MS. Food Chemistry. 2008;107:1668-73.
-
51.
Lima C, Azevedo M, Araujo R, Fernandes-Ferreira M, Pereira-Wilson C. Metformin-like effect of Salvia officinalis (common sage): is it useful in diabetes prevention? British J. Nutrition. 2006;96:326-33.
-
52.
Shim YJ, Doo HK, Ahn SY, Kim YS, Seong JK, Park IS, Kim BH. Inhibitory effect of aqueous extract from the gall of Rhus chinensis on alpha-glucosidase activity and postprandial blood glucose. Journal of Etnhopharmacology. 2003;85:283-7.