Abstract
Keywords
Beta-Amyloid Melissa officinalis Fractions Neuroprotection Oxidative stress
Introduction
Alzheimer’s disease (AD) is a type of degenerative disease of the central nervous system in elderly patients. Neurodegenerative disorder was characterized by cognitive disabilities with severe dementia, memory deficiency, abstract thinking and personality alteration. (1). Two hallmarks in the pathogenesis of AD are neurofibrillary tangles (NFT) and neuritic plaques. The major component of plaques, which are extracellular deposits, is aggregated fibrillar β-amyloid (Aβ) peptide (2). Several microscopic studies of post-mortem human brain tissue of AD patients suggest that extracellular deposition of Aβ peptides plays the most important role in AD pathogenesis (3). Aβ peptide is generated from β-amyloid precursor protein (APP) by sequential cleavage through β-secretase and γ-secretase. Abnormal metabolism of APP results in increased production, accumulation and deposition of Aβ and lead to neuronal cell death in AD (4). Several mechanisms that proposed to explain the neurotoxicity of Aβ includes: production of reactive oxygen species (ROS) and oxidative stress, mitochondrial abnormalities and depletion of cellular ATP, elevated intracellular calcium and excitotoxicity and induction of inflammatory responses. All this mechanisms result in synaptic dysfunction and finally neuronal loss through activation of apoptotic and necrotic cell death pathways (2).
Extensive evidence suggests that the oxidative stress plays an important role in pathogenesis of AD. Oxidative damage induced by ROS, including protein, DNA, RNA oxidation and lipid peroxidation, have been described in the AD brain. It is supposed that the Aβ toxicity, at least partially, is induced by free radicals (5).
Therefore, therapeutic effort for attenuating the free radicals or preventing their production could be beneficial in AD treatment. Hence, antioxidants may be merged as one of therapeutic strategies to attenuate Aβ-induced neurotoxicity and improve neurological outcome in AD (6, 7).
Moreover, gradually loss of cholinergic neurons in basal forebrain is contributed with AD pathology via memory deficiency (8). Drugs approved for AD treatment are acetylcholinesterase (AChE) inhibitors which can improve cognitive impairment but cannot prevent disease progression (9). Because of complex pathology of AD, new attempts are going to produce therapeutic agent that can halt disease progress through different pathways (10). Herbal medicines pose several components with different pharmacological effects and may be more effective in complex diseases. In recent years, several studies were considered as therapeutic effects of herbal medicines in AD therapy (11).
Melissa officinalis (lemon balm) is a medicinal plant from Lamiaceae family. It has been used as folk medicine for long time in Iran (12). Medicinal preparations of this herb were used for treatment of indigestion, anemia, palpitation and mood disorders (13). M. officinalis has effect on nervous disorders including the reduction of excitability, anxiety and stress, and sleep disturbance (14). Moreover, M. officinalis has neurotropic activity (15). Total extract of M. officinalis and different fractions of it have anticholinesterase activity (16). M.officinalis extract shows the potent antioxidant activity and plant extracts could protect cells against oxidative damage induced by different pro-oxidant agents which eventually leads to lipid peroxidation by different process (17). Furthermore, the administration of M. officinalis extract in AD patients can improve symptoms of disease (18). However, the mechanism and constituents involved in its neuroprotective properties are not well known. It is claimed that the main effective components of this plant are polyphenols and terpenoid compounds. The aim of our study was to investigate and compare the neuroprotective effect of total ethanolic extract, acidic fraction which contains polyphenols and non-acidic fraction which is free of polyphenols. In addition, the effects of the extracts on oxidative stress biomarkers and cholinesterase activity were studied as well.
Experimental
Materials
Rat pheochromocytoma (PC12) cell line was purchased from national cell bank of Iran (NCBI, Pasteur Institute of Iran). Aβ(25-35 ),2′7′-dichlorofluorescin diacetate (DCFHDA), 3-(4,5-dimethylthiazol-2-yl)-2,5-diphenyl tetrazolium bromide (MTT), poly-D-lysin (PDL), Glutathione Peroxidase (GSH-Px) activity assay kit, Acetylcholinesterase (AChE, Type V-S, lyophilized powder, 1000 unit/mg protein), 5,5-Dithiobis-(2-nitrobenzoic acid) (DTNB), acetylthiocholine iodide, Malondialdehyde bis (dimethyl acetal) and Thiobarbituric acid were purchased from Sigma. RPMI 1640 medium, penicillin-streptomycin and fetal bovine serum (FBS) were purchased from Gibco.
Plant material
The leaves of Melissa officinalis were collected from Gorgan (Golestan province) in Jun 2009 and identified by M. Kamalinejad, botanist from Faculty of Pharmacy, Shahid Beheshti University of Medical Sciences. A voucher specimen was kept in Herbarium of Faculty of Pharmacy, SBMU, Tehran, Iran (NO. 545).
Plant extraction
Total plant extract was obtained by the extraction of dried and milled plant leaves with ethanol 80% (1:10) by using maceration method for 4 days. After every 24 h, the mixture was filtered and new solvent was added to the plant powder. The combined extracts were concentrated to dryness.
In order to prepare acidic fraction of the plant, 50 mL of NaOH 0.1 N was added to 4 g of plant total extract and mixed. The aqueous phase was separated and this process was repeated for two more times. Nonaqueous phase contained nonacidic fraction. Aqueous phase was acidified with HCl 1 N and extracted with ethyl acetate for three times. The combined ethyl acetate layers were concentrated under vacuum pressure to dryness (acidic fraction) (19).
Cell culture and treatment
PC12 cells were cultured on PDL-coated flasks containing RPMI 1640, supplemented with 10% (v/v) heat-inactivated fetal bovine serum, and 1% (v/v) penicillin and streptomycin. Cultures were maintained at 37°C in a humidified atmosphere containing 5% CO2. These cells were seeded at appropriate densities on PDL coated 96- well plates for viability assay or 6-well plates for oxidative stress biomarkers assay. Twenty-four hours after the seeding, cells were preincubated with different concentrations of total extract (0.1-100 μg/mL), acidic fraction (0.001-10 μg/ mL) and non-acidic fraction (0.01-10 μg/mL) for 1 h and incubated with 20μM Aβ peptide for additional 24 h. Stock solution of total extract and acidic fraction were prepared in PBS and further diluted with the medium to appropriate concentrations. Non-acidic fraction was
dissolved in DMSO and diluted with the medium to proper concentrations. Final concentration of
DMSO was 0.1% in medium. Stock solution of Aβ peptide (1 mM) was prepared by dissolving 1 mg in 1 mL sterile distillated water and stored in - 80°C until use. Prior to use, Aβ peptide was
aggregated for 3 days in 37°C.
Cell viability assay
PC12 cells were plated in PDL-coated 96-well plates (104 cells/well) and incubated with Aβ peptide with or without different concentrations of total extract and fractions as described above for 24 h. After the incubation, the medium was replaced with fresh medium containing MTT solution (final concentration 0.5 mg/mL) and incubated for 4 h in 37°C. Then, the medium was removed and 100 μL DMSO was added to each well and mixed properly until the blue formazan product completely dissolved. Absorbance was measured at 540 nm in an automated plate reader (BIOTEK) against 670 nm as the reference wavelength. Results were reported as the percentage of control group (20).
Measurement of lipid peroxidation
Malondialdehyde (MDA), the most abundant lipid peroxidation product from PC12 cells, was measured using the thiobarbituric acid (TBA) colorimetric assay. PC12 cells were plated in PDL-coated 6-well plates (106 cells/ well) and incubated with Aβ peptide with or without total extract (10 μg/mL) and acidic fraction (1 μg/mL) as described above for 24\ h. After the incubation, cells were washed with PBS, and then harvested with 1 mL icecold PBS containing 0.5 mM EDTA and 1.13 mM butyl-hydroxytoluene and sonicated for 20 sec. Twenty μL of cell lysate were removed for protein analysis. One volume of cell lysate was mixed with two volume of TBA reagent (containing 3.75% TCA and 0.0925% TBA) and the mixture was incubated at 90°C
for 60 min. After cooling, the mixture was centrifuged at 1000 g for 10 min and the optical density of supernatant was measured in 540 nm in plate reader. MDA standard curve was established using the stable MDA precursor, Malondialdehyde bis (dimethyl acetal). The results are presented as micromole of MDA/ microgram protein (21, 22). The amount of protein was measured by Bradford method (23).
Measurement of ROS production
The cellular ROS was quantified as described by Hong et al. (24). The accumulation of intracellular ROS can be detected by using DCFH-DA, which crosses cell membranes and is hydrolyzed enzymatically by intracellular esterases to nonfluorescent dichlorofluorescein (DCFH) that is often used as an indicator of ROS. In the presence of ROS, DCFH is oxidized to highly fluorescent dichlorofluorescein (DCF). After the incubation of PC12 cells with Aβ peptide with or without total extract (10 μg/ mL) and acidic fraction (1μg/mL), the cells were harvested by trypsinization and incubated with 10 μmol DCFH-DA in PBS containing 5.6 mmol glucose at 37ºC for 40 min and then centrifuged and suspend in 1 mL PBS buffer. The fluorescence intensity was measured by flowcytometry (BD, U.S.A.) at an excitation wavelength of 488 nm and an emission wavelength of 525 nm.
Glutathione peroxidase activity
PC12 cells were seeded in PDL coated 6-well plates (106 cells/well) and incubated with Aβ peptide with or without total extract (10 μg/mL) and acidic fraction (1 μg/mL) as described above for 24 h. After the incubation, cells were washed with PBS and harvested with trypsinization and homogenated in PBS. The homogenate was centrifuged at 1000 g for 10 min and supernatant was used for enzyme activity and protein assay. The activities of GSH-Px were measured by using the assay kits in accordance with the instructions supplied by the manufacturers.
In-vitro acetylcholinesterase activity assay
AChE activity assays were carried out using an acetylthiocholine iodide substrate-based colorimetric method, as described by Ellman (25). Briefly, 3 mL phosphate buffer (pH = 8), 100.0 μL Dithiobisnitrobenzoic acid (DTNB) 0.01 M as reagent, 50 μL AChE enzyme (3IU) and 50 μL extract were mixed and immediately after adding, 20 μL acetylthiocholine iodide (0.075 M) as a substrate, changes in absorbance at 412 nm was measured by spectrophotometer, in 30 sec interval during 6 min. a blank containing all components except AChE was run in parallel with sample in order to delete the spontaneous and non-enzymatic break down of acetylthiocholine. The reaction rates were calculated, and the percent inhibition of test compounds was determined.
Statistical analysis
All data were represented as the mean ± SE of three separate experiments. Statistical differences were estimated by using oneway ANOVA followed with Newman-Keuls Multiple Comparison Test.
Results
Cell viability
The protective effect of M. officinalis total extract and acidic and non-acidic fractions were evaluated by MTT assay. Twenty-four hours treatment of PC12 cells with Aβ significantly reduced the cell viability. Pretreatment of cells for 1 h with different concentration of total extract and acidic fraction before adding Aβ peptide protected them from Aβ-induced toxicity (Figures 1A and B). These effects were dose-dependent 0.1 μg/mL of total extract and 0.001 μg/mL acidic fraction could slightly increase the cell viability, however, 10 μg/mL of total extract and 1 μg/mL of acidic fraction completely reversed the Aβ-induced toxicity and increased cell viability up to control level. As represented in Figure 1C, pretreatment of cells with non-acidic fraction could not protect them from Aβ-induced toxicity. Treatment with total extract (0.1-100 μg/mL), acidic fraction (0.001-10 μg/mL) and non-acidic fraction (0.01-10 μg/mL) alone did not decrease the cell viability compared to the control group (data not shown).
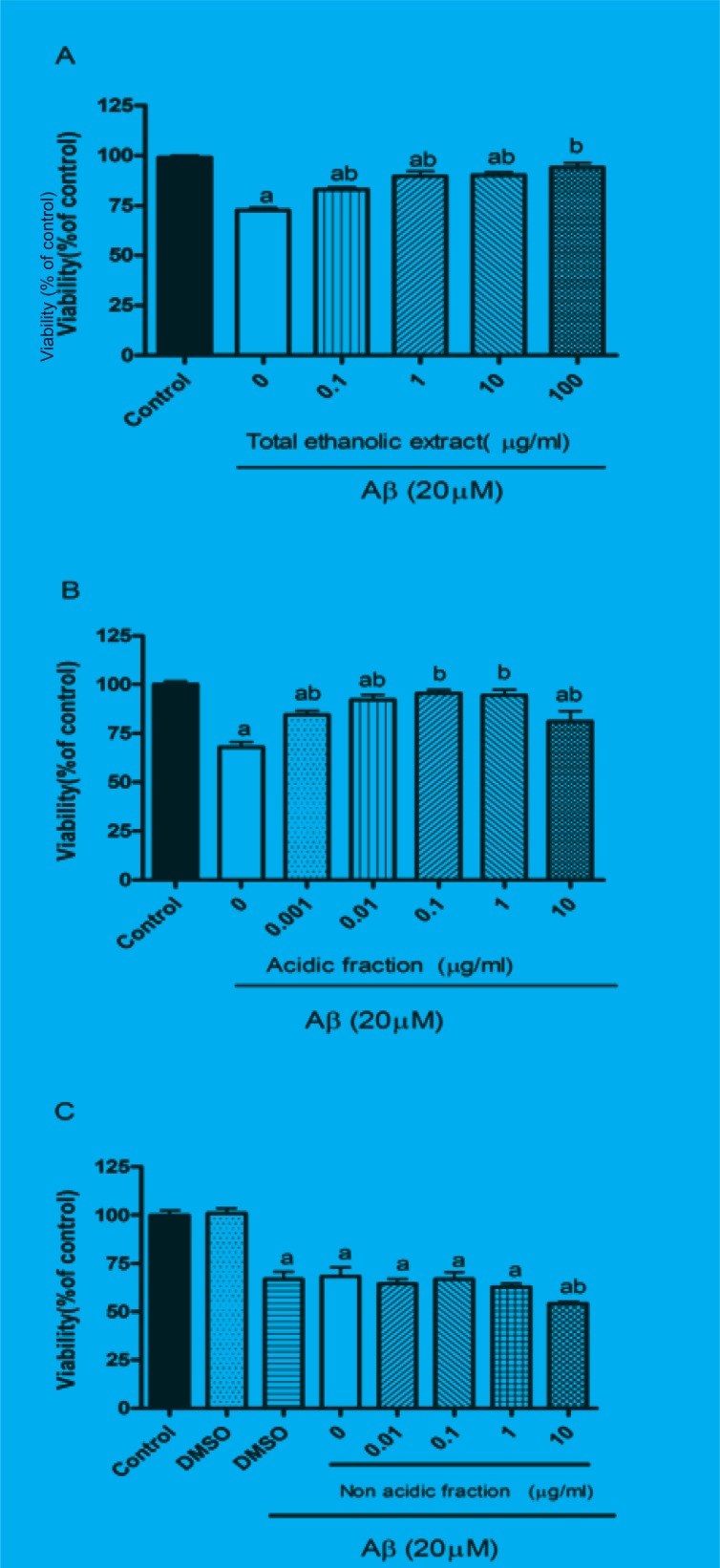
Effect of M. officinalis total extract and acidic fraction on lipid peroxidation
The amount of intracellular MDA which is the product of lipidperoxidation was significantly increased when cells were incubated for 24 h with Aβ. Pretreatment of cells with 10 μg/mL of total extract and 1 μg/mL of acidic fraction significantly attenuated the Aβ-induced MDA production. Treatment of cells with 10 μg/mL of total extract and 1 μg/mL of acidic fraction alone has not any effect on MDA production (Figure 2).
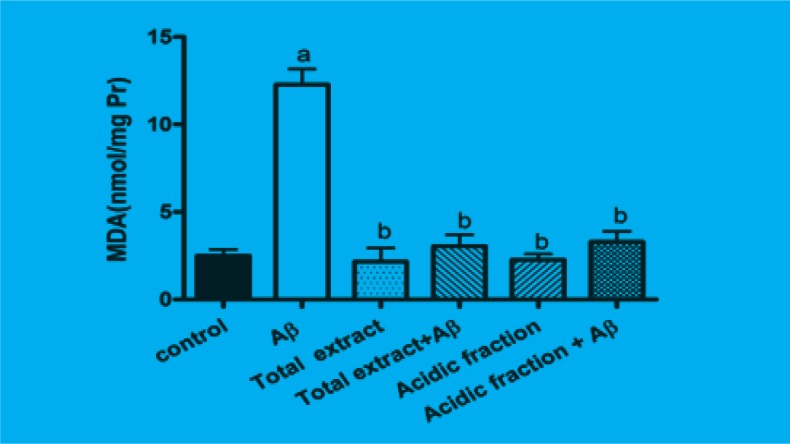
Effect of M. officinalis total extract and acidic fraction on GSH-Px activity
GSH-Px is the most important antioxidant enzyme in cells. As shown in Figure 3, after 24 h of incubating the cells with Aβ, GSH-Px activity was significantly reduced as compared to the control group (p < 0.001). Pretreatment with 10 μg/mL of total extract and 1 μg/mL of acidic fraction attenuated the changes in GSHPx activity induced by Aβ treatment.
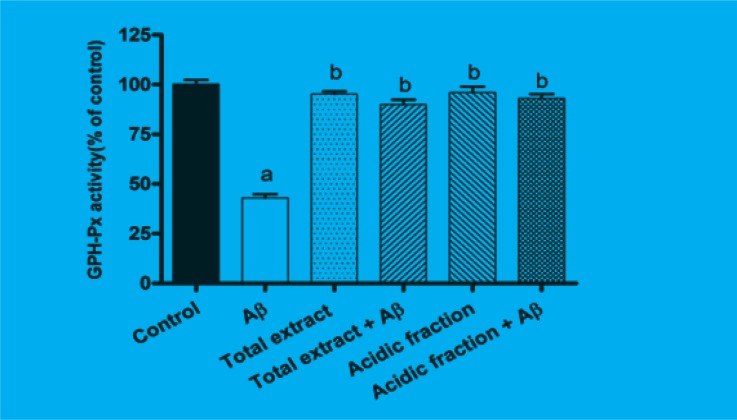
Effect of M. officinalis total extract and acidic fraction on ROS production
The treatment of PC12 cells with Aβ was found to increase the intracellular ROS levels (19). Exposure of PC12 cells to Aβ caused an increase in DCF fluorescence which is indicator of ROS production. When the cells were pretreated with 10 μg/mL of total extract and 1 μg/mL of acidic fraction before the addition of Aβ, the mean fluorescence intensities were lower than that of Aβ treatment group (Figure 4).
Effect of M. officinalis total extract and acidic fraction on AChE activity
Table 1 represents the percentage of enzyme activity inhibition in present of different concentrations of total extract and acidic fraction. Both total extract and acidic fraction inhibited enzyme activity about 50% in high concentrations (500 μg/mL); no enzyme activity inhibition was observed for 10 μg/mL of total extract and 1 μg/mL of acidic fraction.
Inhibitory Activities of M. officinalis total extract and acidic fraction against AChE activity in-vitro.
Compounds | Concentrations(μg/mL) | Inhibition of AChE activity (%) |
---|---|---|
M. Officinalis total extract | 10 | N* |
100 | 21.08 ± 2.5 | |
500 | 40.47 ± 3.2 | |
Acidic fraction | 10 | N |
100 | 33.25 ± 1.98 | |
500 | 53.51 ± 1.7 |
Discussion
PC12 cells have been widely used as an experimental model to study cellular neurotoxicity (27). Studies have shown that Aβ25-35 induced cytotoxicity in PC12 cells, which was accompanied with excessive ROS production, mitochondrial dysfunction (28) apoptosis and cell death (29), therefore, several studies use PC12 cells for studying agents against Aβ toxicity. Results of our study show that M. officinalis total extract can attenuate the Aβ-induced toxicity and oxidative stress. This plant has potent antioxidant activity with direct free radical scavenging activity, and it is reported that M. officinalis total extract can protect PC12 cells against hydrogen peroxide-induced cell death and oxidative stress (30). Oxidative stress is the mechanism that was assumed
for Aβ toxicity and Alzheimer’s etiology (6). Antioxidant can ameliorate disease progression (7), so it is supposed that antioxidant activity may be contributed to neuroprotective effect of M. officinalis against Aβ toxicity. In addition, we showed that the acidic fraction of extract is potent
and the total extract and non-acidic fraction have not protective effect. These findings led us to this fact that the effective components of extract were concentrated in acidic fraction. These components are polyphenols, flavonoids and terpenoids. The polyphenols that were found in M. officinalis extract are Caffeic acid derivatives and among them, rosmarinic acid is the major polyphenol component. Several biological activities have been described for rosmarinic acid, including antioxidative, anti-inflammatory, anti-mutagenic, antibacterial and antiviral activities (31). Rosmarinic acid is a potent antioxidant with direct free radical scavenging activity. Neuroprotective activity was also reported for this compound. It is reported that rosmarinic acid can protect Aβ-induced memory impairment in mice and this effect is due to the direct peroxynitrite scavenging activity (32). Moreover, pretreatment of PC12 cells with rosmarinic acid can protect them from Aβ- induced toxicity. It is suggested that rosmarinic acid inhibits oxidative stress and apoptosis (33). Another property reported for rosmarinic acid in literature is anti-acetylcholinesterase activity. Dastmalchi and et al. studied the anticholinesterase activity of M officinalis ethanolic extracts and fractions; they reported that one fraction of extract which contains rosmarinic acid has high anti-cholinesterase activity even more than total ethanolic extract (16).
The caffeic acid, a cinnamic acid derivative, and the ursolic acid, a triterpenoid, may contribute
with protective activity of M. officinalis. The neuroprotective effects for these compounds were
reported. Ursolic acid and Caffeic acid can rescue Aβ-induced oxidative stress in PC12 cells (34,
35). Ursolic acid is a triterpenoid with hydroxyl radical scavenging activity and it increases the activities of antioxidant enzymes such as superoxide dismutase, catalase and glutathione peroxidase (34). Besides, ursolic acid isolated from oregano (Origanum majorana L.) inhibited the enzyme acetylcholinesterase (35).
In the present study, we assessed the effect of total extract and acidic fraction on acetylcholine esterase activity. We found that both total extract and acidic fraction could inhibit acetylcholine esterase activity and acidic fraction was more potent compared to the total extract, which was in agreement with the previous study as rosmarinic acid is concentrated in acidic fraction. But this effect was observed in high concentrations and in concentrations which total extract and acidic fraction had protective effect against Aβ- induced toxicity. Anti-cholinesterase activity was not observed in the total extract and acidic fraction, leading to the fact that protective effect of extract and acidic fraction were not attributed to inhibition of cholinesterase enzyme.
It is reported that the M. officinalis extract contains compounds with acetylcholine receptor affinities and that the affinity for nicotinic receptor is more than muscarinic receptor. IC50-values for [3H] nicotine displacement for ethanolic extract of M. officinalis were lower than 100 μg/mL (36). Several studies have been performed to show the role of nicotinic receptors in Aβ-induced toxicity. In-vitro studies showed that nicotine has protective effect on Aβ-induced toxicity and preincubation of neurons with nicotine attenuated the Aβ-induced oxidative stress and apoptosis. The protective effect of nicotine was antagonized with mecamylamine, a nicotinic receptor antagonist. Results of these studies indicated that the nicotinic receptor stimulation can protect neurons against Aβ- induced toxicity (37, 38). According to these studies, it can be suggested that the protective effect of M. officinalis and acidic fraction against Aβ-induced toxicity may be exerted through the nicotinic receptors. The nature of compounds in M. officinalis extract which have nicotinic receptor affinity, were unknown. Our finding ruled out the existence of basic nitrogenous compounds, as these compounds, in case of existence, would be concentrated in basic fraction, and our result showed that the basic fraction of extract did not have any protective effect against the Aβ-induced toxicity.
Conclusion
Our finding represents that the acidic fraction of M. officinalis has significant protective effect on Aβ-induced toxicity and oxidative stress. These effects do not contribute to the anti-acetylcholinesterase activity of extract. According to our finding and previous study, we concluded that the protective effect of this plant contribute to the polyphenols and triterpenoids
and may be exerted through antioxidant mechanism or nicotinic receptor stimulation. These hypotheses need further investigation, and we will study it in our future research.
References
-
1.
Blennow K, de Leon MJ, Zetterberg H. Alzheimer's disease. Lancet. 2006;368(9533):387-403. [PubMed ID: 16876668].
-
2.
Hardy J, Selkoe DJ. The amyloid hypothesis of Alzheimer›s disease: progress and problems on the road to therapeutics. Science. 2002;297:353-6. [PubMed ID: 12130773].
-
3.
Gandy S. The role of cerebral amyloid beta accumulation in common forms of Alzheimer disease. J. Clin. Invest. 2005;115:1121-9. [PubMed ID: 15864339].
-
4.
Nathalie P, Jean-Noël O. Processing of amyloid precursor protein and amyloid peptide neurotoxicity. Curr. Alzheimer Res. 2008;5:92-9. [PubMed ID: 18393795].
-
5.
Butterfield DA, Drake J, Pocernich C, Castegna A. Evidence of oxidative damage in Alzheimer›s disease brain: central role for amyloid beta-peptide. Trends.Mol. Med. 2001;7:548-54. [PubMed ID: 11733217].
-
6.
Christen Y. Oxidative stress and Alzheimer disease. Am. J. Clin. Nutr. 2000;71:621S-9S. [PubMed ID: 10681270].
-
7.
Aliev G, Obrenovich ME, Reddy VP, Shenk JC, Moreira PI, Nunomura A, Zhu X, Smith MA, Perry G. Antioxidant therapy in Alzheimer›s disease: theory and practice. Mini. Rev. Med. Chem. 2008;8:1395-406. [PubMed ID: 18991755].
-
8.
Schliebs R. Basal forebrain cholinergic dysfunction in Alzheimer›s disease--interrelationship with betaamyloid, inflammation and neurotrophin signaling. Neurochem. Res. 2005;30:895-908. [PubMed ID: 16187224].
-
9.
Lane RM, Kivipelto M, Greig NH. Acetylcholinesterase and its inhibition in Alzheimer disease. Clin. Neuropharmacol. 2004;27:141-9. [PubMed ID: 15190239].
-
10.
Carreiras MC, Marco JL. Recent approaches to novel anti-Alzheimer therapy. Curr. Pharm. Des. 2004;10:3167-75. [PubMed ID: 15544506].
-
11.
Anekonda TS, Reddy PH. Can herbs provide a new generation of drugs for treating Alzheimer›s disease? Brain. Res. Rev. 2005;50:361-76.
-
12.
Zargari AI. Medicinal plants. 1. Tehran: Tehran University Press; 1990. p. 77-81.
-
13.
Naghibi F, Mosaddegh M, Mohammadi motamed S, Ghorbani A. Labiatae family in folk medicine in Iran: from ethnobotany to pharmacology. Iranian J.Pharm. Res. 2009;4:63-79.
-
14.
Dobetsberger C, Buchbauer G. Actions of essential oils on the central nervous system: An updated review. Flavour. Frag. J. 2011;26:300-16.
-
15.
Soulimani R, FleurentinL J, Mortier F, Misslin R, Derrieu G, Pelt JM. Neurotropic action of the hydroalcoholic extract of Melissa officinalis in the mouse. Planta Med. 1991;57:105-9. [PubMed ID: 1891490].
-
16.
Dastmalchi K, Ollilainen V, Lackman P, Boije af Gennas G, Dorman HJ, Jarvinen PP, Yli-Kauhaluoma J, Hiltunen R. Acetylcholinesterase inhibitory guided fractionation of Melissa officinalis L. BioorgMed. Chem. 2009;17:867-71.
-
17.
Pereira RP, Fachinetto R, de Souza Prestes A, Puntel RL, Santos da Silva GN, Heinzmann BM, Boschetti TK, Athayde ML, Bürger ME, Morel AF, Morsch VM, Rocha JB. Antioxidant effects of different extracts from Melissa officinalis, Matricaria recutita and Cymbopogon citratus. Neurochem. Res. 2009;34:973-83. [PubMed ID: 18853256].
-
18.
Akhondzadeh S, Noroozian M, Mohammadi M, Ohadinia S, Jamshidi AH, Khani M. Melissa officinalis extract in the treatment of patients with mild to moderate Alzheimer›s disease: a double blind, randomised, placebo controlled trial. J. Neurol. Neurosurg Psychiatry. 2003;74:863-6. [PubMed ID: 12810768].
-
19.
Hajimehdipour H, Amanzadeh Y, Sadat Ebrahimi SE, Mozaffarian V. Three Tetraoxygenated Xanthones from Swertia longifolia. Pharm. Biol. 2003;41:497-499.
-
20.
Shi da H, Wu JH, Ge HM, Tan RX. Protective effect of hopeahainol A, a novel acetylcholinesterase inhibitor, on hydrogen peroxide-induced injury in PC12 cells. Environ. Toxicol. Pharmacol. 2009;28:30-6. [PubMed ID: 21783979].
-
21.
Pavlica S, Gebhardt R. Protective effects of flavonoids and two metabolites against oxidative stress in neuronal PC12 cells. Life Sci. 2010;86:79-86. [PubMed ID: 19891977].
-
22.
Li RC, Pouranfar F, Lee SK, Morris MW, Wang Y, Gozal D. Neuroglobin protects PC12 cells against beta-amyloid-induced cell injury. Neurobiol Aging. 2008;29:1815-22. [PubMed ID: 17560688].
-
23.
Bradford MM. A rapid and sensitive method for the quantitation of microgram quantities of protein utilizing the principle of protein-dye binding. Analytical biochemistry. 1976;72:248-254. [PubMed ID: 942051].
-
24.
Wang H, Joseph JA. Quantifying cellular oxidative stress by dichlorofluorescein assay using microplate reader. Free Radic Biol. Med. 1999;27:612-6. [PubMed ID: 10490282].
-
25.
Ellman GL, Courtney KD, Andres VJr, Feather- Stone RM. A new and rapid colorimetric determination of acetylcholinesterase activity. Biochem Pharmacol. 1961;7:88-95. [PubMed ID: 13726518].
-
26.
Ge YS, Teng WY, Zhang CD. Protective effect of cyclophilin A against Alzheimer›s amyloid betapeptide (25-35)-induced oxidative stress in PC12 cells. Chin. Med. J. 2009;122:716-24. [PubMed ID: 19323941].
-
27.
Koh SH, Kwon H, Park KH, Ko JK, Kim JH, Hwang MS, Yum YN, Kim OH, Kim J, Kim HT, Do BR, Kim KS, Kim H, Roh H, Yu HJ, Jung HK, Kim SH. Protective effect of diallyl disulfide on oxidative stress-injured neuronally differentiated PC12 cells. Brain. Res. Mol. Brain Res. 2005;133:176-86. [PubMed ID: 15710234].
-
28.
Gao X, Tang XC. Huperzine A attenuates mitochondrial dysfunction in beta-amyloid-treated PC12 cells by reducing oxygen free radicals accumulation and improving mitochondrial energy metabolism. J. Neurosci. Res. 2006;83:1048-57. [PubMed ID: 16493671].
-
29.
Lin YH, Liu AH, Wu HL, Westenbroek C, Song QL, Yu HM, Ter Horst GJ, Li XJ. Salvianolic acid B, an antioxidant from Salvia miltiorrhiza, prevents Abeta(25-35)-induced reduction in BPRP in PC12 cells. Biochem. Biophys. Res. Commun. 2006;348:593-9. [PubMed ID: 16890202].
-
30.
López V, Martín S, Gómez-Serranillos MP, Carretero ME, Jäger AK, Calvo MI. Neuroprotective and neurological properties of Melissa officinalis. Neurochemical Res. 2009;34:1955-1961.
-
31.
Petersen M, Simmonds MS. Rosmarinic acid. Phytochemistry. 2003;62:121-5. [PubMed ID: 12482446].
-
32.
Alkam T, Nitta A, Mizoguchi H, Itoh A, Nabeshima T. A natural scavenger of peroxynitrites, rosmarinic acid, protects against impairment of memory induced by Abeta (25-35). Behav Brain Res. 2007;180:139-45. [PubMed ID: 17420060].
-
33.
Iuvone T, De Filippis D, Esposito G, D›Amico A, Izzo AA. The spice sage and its active ingredient rosmarinic acid protect PC12 cells from amyloid-beta peptide-induced neurotoxicity. J. Pharmacol. Exp.Ther. 2006;317:1143-9. [PubMed ID: 16495207].
-
34.
Liu J. Pharmacology of oleanolic acid and ursolic acid. J. Ethnopharmacol. 1995;49:57-68. [PubMed ID: 8847885].
-
35.
Novotny L, Vachalkova A, Biggs D. Ursolic acid: an anti-tumorigenic and chemopreventive activity. Minireview. Neoplasma. 2001;48:241-6. [PubMed ID: 11712672].
-
36.
Wake G, Court J, Pickering A, Lewis R, Wilkins R, Perry E. CNS acetylcholine receptor activity in European medicinal plants traditionally used to improve failing memory. J. Ethnopharmacol. 2000;69:105-14. [PubMed ID: 10687867].
-
37.
Kihara T, Shimohama S, Sawada H, Kimura J, Kume T, Kochiyama H, Maeda T, Akaike A. Nicotinic receptor stimulation protects neurons against betaamyloid toxicity. Ann. Neurol. 1997;42:159-63. [PubMed ID: 9266724].
-
38.
Liu Q, Zhao B. Nicotine attenuates beta-amyloid peptide-induced neurotoxicity, free radical and calcium accumulation in hippocampal neuronal cultures. Br. J. Pharmacol. 2004;141:746-54. [PubMed ID: 14757701].