Abstract
Keywords
Rheum turkestanicum Janischew. Polygonaceae Cytotoxicity Apoptosis Cancer
Introduction
Most cancer chemotherapy agents currently in clinical use have originated from plants or are analogs of plant-derived compounds (1-2). Only 41.4% of the compounds reported in natural product databases, such as Napralert, have been studied from a biological perspective (3). The use of naturally occurring compounds with chemopreventive properties attracted much interest in chemotherapy and treatment of cancers. The anti-cancer properties of a multitude of medicinal herbs mediated through different mechanisms include altered carcinogen metabolism, induction of DNA repair systems, immune activation and suppression of cell cycle progression/induction of apoptosis. While cancer cell death/apoptosis could be considered a convergence point of all anti-neoplastic therapies, direct pro-apoptotic effects have been reported for bioactive phytochemicals (4).
Rheum species (Polygonaceae) have a long history as medicinal plants in traditional Chinese medicine. Rhei Rhizoma (Dahuang) is a Chinese herbal medication that has traditionally been prescribed for its purported purgative and anti-inflammatory properties. According to the Chinese Pharmacopoeia, Rhei Rhizoma is derived from one of three Rheum species, R. palmatum, R. tanguticum, and R. officinale. The main active ingredients of the Rheum species are a series of anthraquinones, dianthrones, glycosides and tannins. The anthraquinone derivatives include emodin, rhein, chrysophanol, physcion, alizarin, citreorosein, and aloe-emodin (5).
Recently, the antitumor activity of these plants has attracted much attention. Roots of R. emodi are reported to have antibacterial, antifungal, laxative, diuretic, and in-vivo inhibitory effects towards P388 leukemia in mice. Aqueous and methanolic extracts of R. emodi showed concentration-dependent cytotoxicity when tested in MDA-MB-435S (human breast carcinoma) and Hep3B (liver carcinoma) cell lines (6).
R. officinale Baill. is one of the herbs commonly used in formulas of Traditional Chinese Medicine (TCM) prescribed to cancer patients. It has been reported to have anti-tumor activity with hepatocarcinoma and to significantly inhibit the proliferation of A549 and MCF-7 cells in vitro, confirmed by the cell viability and colony formation assays. Its cytotoxic activity is likely to be due to the induction of apoptosis (7). The n-hexane extract of R. undulatum L. has been shown to have anti-cancer properties in several cancers in-vivo and in-vitro (8). Numerous reports have shown that emodin, aloe-emodin and rhein have anti-proliferative effects on many kinds of cancer cell lines such as HER-2/neu over expressing breast cancer, leukemia, hepatoma, human myeloma and lung cancer with the involvement of apoptosis or programmed cell death (9-12).
Rheum turkestanicum (Polygonaceae) is a plant that grows widely in central Asia and also in north-east of Iran. Traditionally, people use roots of R. turkestanicum as an anti-diabetic and anti-hypertensive as well as anticancer agent. In spite of the traditional use of R. turkestanicum as an anti-cancer plant and widespread researches about the cytotoxic and antitumor effects of some species of the Rheum genus, there is not any reported literature on R. turkestanicum.
The aim of this study was to investigate the in-vitro cytotoxic activity of ethyl acetate (EtOAc), n-hexane and H2O extracts from Rheum turkestanicum. Therefore, in an attempt is sought to study cytotoxic properties of three different extracts (ethyl acetate (EtOAc), n-hexane and H2O) of R. turkestanicum root on two cancer cell lines, including human cervix carcinoma cell line (HeLa) and human breast cancer cell line (MCF-7) and non-malignant cells (Human Blood Lymphocytes). The role of apoptosis in R. turkestanicum induced cytotoxicity on cancer cell lines has also been reported. In this study, we used Paclitaxel as a positive control (13).
Experimental
Reagents
MTS (3-(4, 5-Dimethylthiazol-2-yl) -5-(3-carboxymethoxyphenyl) -2- (4-sulfophenyl) -2H-tetrazolium) from Promega (Madison, WI, USA); (RPMI-1640) and FCS from Gibco; Lympholyte®-H from Cedarlane (Canada); β-actin and PARP antibodies, anti- rabbit IgG and HRP linked antibody from Cell Signaling technology (Boston, USA); ECL Western blotting detection reagent from Bio-RaD (USA); the fluorescent probe propidium iodide (PI), protease inhibitor cocktail, phosphatase inhibitor cocktail, sodium citrate, Triton X-100, phenylmethylsulfonyl fluoride and QuantiPro BCA assay kit were purchased from Sigma (Steinheim, Germany).
Plant materials
The root of R. turkestanicum was collected from Chenar, a village in Zavin Rural District, Kalat County, Razavi Khorasan Province, Iran.The plant was identified by M. R. Joharchi, from Ferdowsi University of Mashhad Herbarium. Voucher specimen (No. 42082) was deposited in Ferdowsi University of Mashhad Herbarium. Dried R. turkestanicum root (20 g×3) was ground into fine powder and then was percolated with 100 ml of each solvent (EtOAc, n-hexane and H2O). After 24 h, the solutions were centrifuged at 2700 g for 3 min. The supernatants were collected and the residues were re-extracted for one more time with the same volume of solvents (Figure 1). The whole extract were filtered and the solvents were evaporated under reduced pressure at 40–45 °C, each extract was then stored at -20 °C over night, and then it was concentrated by freeze drier. Again the extract kept at -20 °C. The dried extrcts were dissolved in dimethylsulfoxide (DMSO) and were then screened for tumor cell growth inhibition.
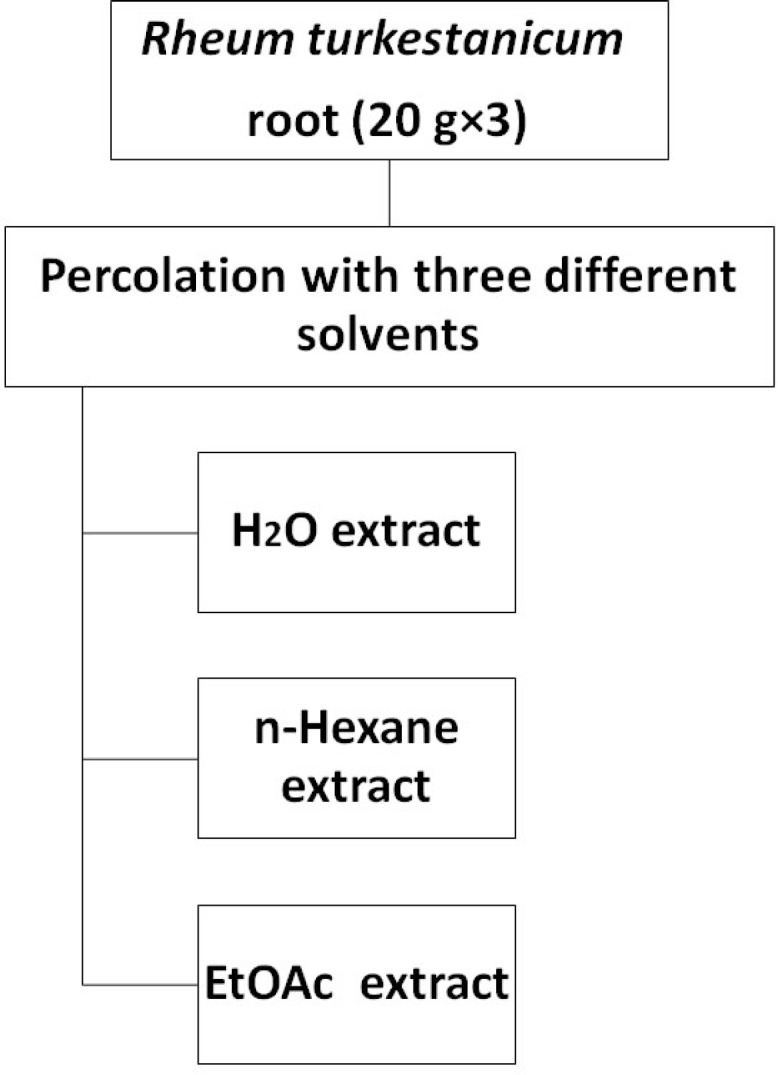
Cell culture
HeLa and MCF-7 cells were obtained from the Pasteur Institute (Tehran, Iran) and lymphocytes isolated from human peripheral blood using the Lympholyte®-H (a density gradient separation medium) according to manufacturer’s protocol and maintained at 37 °C in a humidified atmosphere (90%) containing 5% CO2. Cells were cultured in Roswell Park Memorial Institute-1640 (RPMI-1640) with 10% (v/v) fetal bovine serum, 100 U/mL penicillin, and 100 μg/mL streptomycin. For each concentration and time course study, there was a control sample which remained untreated and received the equal volume of medium.
Leukocyte culture
Human umbilical cord blood samples (50 mL) were collected from a fresh umbilical cord attached to the placenta by gravity flow in a sterile 50 mL syringe containing citrate buffer as an anticoagulant. The sample was diluted with an equal volume of phosphate buffered saline (PBS) and then layered over Lympholyte®-H a density gradient separation solution, and centrifuged at 800 g for 20 min at room temperature. The mononuclear cell layer was removed, washed twice in PBS and resuspended in RPMI 1640 medium supplemented with 2 mM glutamine (Sigma Chemical Co.), antibiotics and 10% FCS. Leukocytes (5×104 cells per well) were incubated with
various concentrations of R. turkestanicum in 96-well plates for 48 h. This study protocol was approved by the ethical committee of Mashhad University of Medical Sciences.
Cell viability
The MTS [3- (4,5-Dimethylthiazol-2-yl)-5-(3-carboxymethoxyphenyl)-2- (4-sulfophenyl) -2H-tetrazolium] growth inhibition assay (14) was performed according to the instructions provided by the manufacturer (Promega). Briefly, the cells were seeded (104 cell/well) onto flat-bottomed 96-well culture plates. The cells were either treated with ethyl acetate, n-hexane and H2O extracts (15-500 μg/mL) over different incubation periods (24, 48 and 72 h), or remained as untreated controls. At the end of each time point, fresh complete medium containing 10 μL of MTS solution was added and further incubated for 2 h. Optical density of each culture was then recorded at 490 nm using an ELISA reader. Each experiment was performed in triplicate and all the extracts were compared with Paclitaxel (0.7 μM) as a positive control. Results are expressed as the percentage growth inhibition with respect to the untreated cells.
Apoptosis
PI staining
Apoptotic cells were detected using PI staining of treated cells followed by flow cytometry to detect the so-called sub-G1 peak (15). Briefly, malignant cells were cultured overnight in a 24-well plate and treated with extracts (EtOAc and n-hexane) for 48 h. Floating and adherent cells were then harvested and incubated at 4 °C overnight in the dark with 750 μL of a hypotonic buffer (50 μg/mL PI in 0.1% sodium citrate plus 0.1% Triton X-100) before flow cytometric analysis using a FACScan flow cytometer (Becton Dickinson). About 10,000 events were acquired with FACS.
Western blotting analysis
HeLa cells were treated with 150 and 200 μg/mL of the EtOAc and n-hexane extracts of R. turkestanicum for 48 h. The cells were harvested and rinsed with ice-cold PBS. The cell pellet was resuspended in a lysis buffer containing 50 mM Tris-HCl (pH 7.4), 150 mM NaCl, 1% triton-X100, 1 mM EDTA, 0.2% SDS, 1% protease inhibitor cocktail, 1% phosphatase inhibitor cocktail and 1 mM phenylmethylsulfonyl fluoride and left on ice for 30 min. After centrifugation at 10000 rpm for 20 min at 4 °C, the cell lysate was collected and protein concentration was determined according to the BCA detection kit (16). Equal amounts of proteins were subjected to 12.5% SDS–PAGE (w/v). The proteins were transferred to a polyvinylidene fluoride (PVDF) membrane and subjected to immunoblotting using β-actin antibody and PARP antibody as primary antibodies and anti- rabbit IgG, HRP linked antibody, as secondary antibodies. PARP cleavage in HeLa cells were detected by enhanced chemi luminescence using the ECL western blotting detection reagent.
DNA fragmentation analysis
To evaluate oligonucleosomal fragmentation, genomic DNA was extracted as previously described (17). MCF-7 cells were treated with different concentrations of each EtOAc extract for 48 h in RPMI 1640 supplemented with 10% (v/v) fetal calf serum, 100 U/mL penicillin and 100 mg/ mLstreptomycin.
The formation of high molecular weight and oligonucleosomal DNA fragments was examined by agarose gel electrophoresis. Cells (106 cells) were seeded onto 6-well plates and treated for 48 h. The cells were collected by centrifugation at 1100 rpm for 7 min. The DNA from treated and untreated cells was extracted as explained below: cells were incubated with 50 μL of lysis buffer (20 mM Tris, 20 mM EDTA, 200 mM NaCl and 1% SDS) and 2 μL RNase A (500 μg/mL) for 15 min at 37 ºC. The cells were further incubated at 37 ºC for 15 min after adding 2.5 μL of 10 mg/mL Proteinase K, which had been preheated at 37 ºC for 30 min. The lysate was mixed with 10 mL of loading solution (30% ficoll, and 1% bromophenol blue in TBE), and then the DNA samples were separated in 2% agarose gel electrophoresis at 50 V, 3 h and visualized with ethidium bromide using Gel Documentation System (Far Gene Pouyesh, Tehran, Iran).
Statistical analysis
One way analysis of variance (ANOVA) and Bonferroni’s post hoc were used for data analysis. All results were expressed as mean±SEM and p-values below 0.05 were considered statistically significant.
Results
Cytotoxicity of various extracts of R. turkestanicum
Malignant cells and human blood lymphocytes (as non-malignant control cells) were incubated with various concentrations of EtOAc, n-hexane and H2O extracts of R. turkestanicum (15- 500 μg/mL) for 24, 48 and 72 h. EtOAc and n-hexane extracts decreased cell viability in malignant cells but not in non-malignant cells, as a concentration and time-dependent manner (Figure 2).
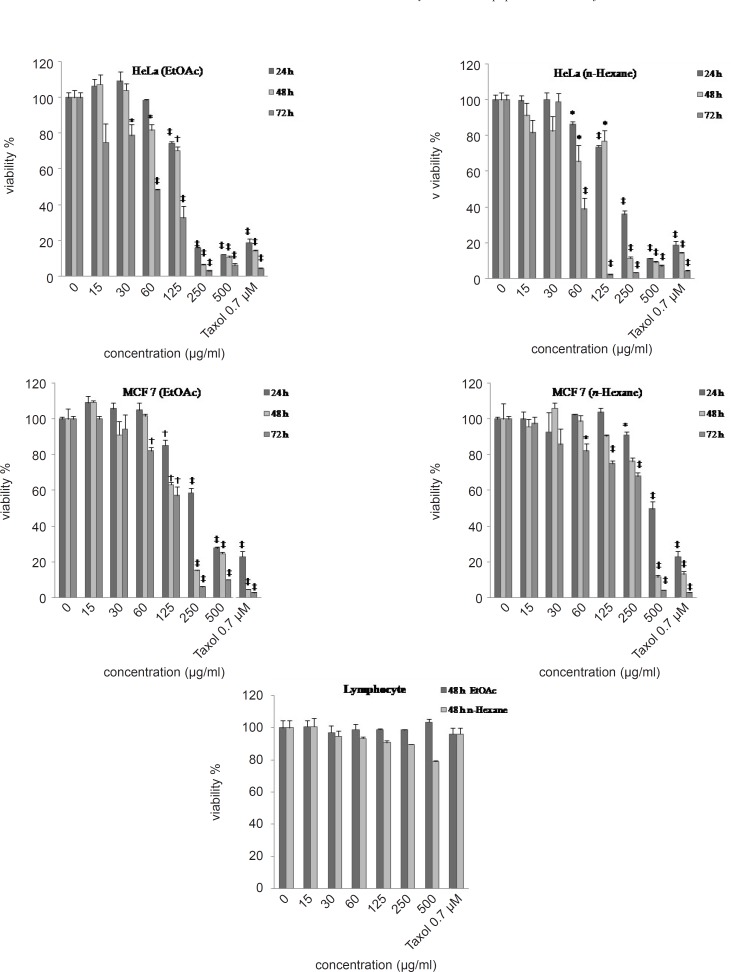
This toxicity was consistent with morphologic changes including reduction in cell volume and rounding (data was not shown). Doses inducing 50% cell growth inhibition (IC50) against HeLa and MCF-7 cells are presented in Table 1.
Doses of toxic extracts of R. turkestanicum inducing 50% cell growth inhibition (IC50) against malignant cell lines. Cells incubated with different concentration of extractsfor 48 h. IC50 values were expressed as the mean ± SEM (n = 3).
Cell line/ Extract | n-Hexane | EtOAc |
---|---|---|
MCF-7 | 320 | 155 |
HeLa | 130 | 150 |
Role of apoptosis
PI staining
The proportion of apoptotic cells was measured with PI staining of DNA fragmentation by flow cytometry. EtOAc extract induced a sub- G1peak (one of the reliable biochemical markers of apoptosis) in flow cytometry histogram of treated cells compared to control (Figure 3).
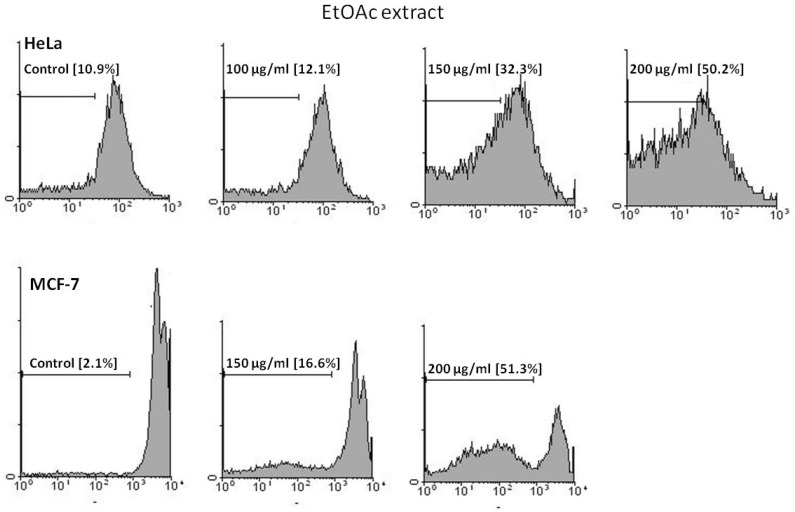
Cleavage of DNA and PARP
We also examined the induction of apoptosis in MCF-7 and HeLa cells through the occurrence of DNA fragmentation and cleavage of PARP proteins respectively. We observed that DNA extracted from untreated MCF-7 cells showed no fragmentation, while DNA from ethyl acetate-treated cells (48 h) showed DNA laddering as a result of endonuclease action at sites between nucleosomes (Figure 4). The suspected involvement of caspase-3 activation in EtOAc induced apoptosis was also validated in HeLa cells through the expression and degradation of a nuclear protein, PARP.
Proteolytic cleavage of full length PARP from a 116 kDa polypeptide to a 24 kDa and 89 kDa segment is a typical marker for the onset of apoptosis (Figure 4). Therefore, the apoptosis inducing effects of EtOAc on malignant cells were confirmed.
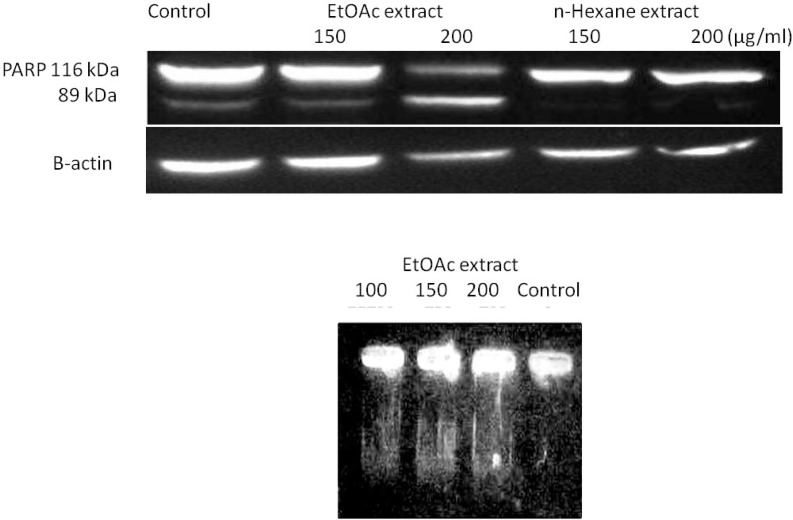
Discussion
We tested ethyl acetate, n-hexane and H2O extracts from R. turkestanicum for its ability to inhibit the growth of HeLa and MCF-7 cells. We also tested the extracts for the ability to induce apoptosis in the mentioned cell lines. Reduced metabolic activity due to the R. turkestanicum extract was demonstrated using MTS. Apoptosis induction was demonstrated by sub-G1 peak in flow cytometry histogram of treated cells compared to control. DNA fragmentation indicating apoptotic cell death was involved in R. turkestanicum-induced toxicity and cleaved PARP fragment was also detected.
In ancient times plants were used to treat various kinds of diseases (10). In traditional cultures herbal plants and plant-derived medicines have been commonly used and today they have become increasingly popular as natural alternatives (18). Nowadays, the origins of 60% of anticancer drugs are from natural products and plant derivatives such as antibiotics. As a result natural products have gained much attention as vital sources for developing of valuble effective anticancer agents (19). There are some reports of the cytotoxic activity of the Rheum species. Although there have been numerous studies on the effects of different Rheum species extracts and isolated phytochemicals on cancer cells, none have studied the effects of R. turkestanicum.
According to our results, the EtOAc and n-hexane extracts of R. turkestanicum caused a concentration and time-dependent inhibition of growth of both malignant cell lines with high IC50 values. The same dose of extract had little, if any, effect on lymphocytes. In fact, 15-500 μg/ mL of R. turkestanicum extract did not induce the same inhibitory effects in lymphocytes that were observed in tested tumor cell lines. The normal cells also failed to undergo the typical morphological changes seen following treatment of the HeLa and MCF-7 cells. This suggests that the R. turkestanicum extract may not affect normal cells, thus warranting in-vivo studies.
Using PI labeling, DNA fragmentation and Western blot analysis for PARP cleavage, we demonstrate that apoptosis is involved in R. turkestanicum-induced cell death. R. turkestanicum extracts exerted their growth arrest on treated cells by increasing the cells at subG1-phase and DNA laddering implying that R. turkestanicum extracts causes separating PARP into a 24 kDa and 89 kDa segment and subsequently initiating DNA fragmentation.
Apoptosis is a critical pathway occurs in development, immunological competence, and homeostasis. Cellular hallmark of apoptosis includes marked changes in morphology, including chromatin condensation, membrane blebbing, nuclear breakdown, and the appearance of membrane-associated apoptotic bodies, internucleosomal DNA fragmentation, as well as cleavage of poly (ADP-ribose) polymerase (PARP) (20-21). PARP catalyzes the poly (ADP-ribosyl) action of a variety of nuclear proteins with NAD (Nicotinamide adenine dinucleotide) as substrate. PARP cleavage into 89- and 24-kDa segments that contain the active site and the DNA-binding domain of the enzyme basically inactivates the enzyme by destroying its ability to respond to DNA strand breaks. Because it is activated by binding to DNA ends or strand breaks, PARP was suggested to contribute to drug-induced apoptosis in a variety of cells by depleting the cell of NAD and ATP after cleavage (22-24).
The activation of caspase-3 in treated cancer cells during apoptosis results PARP cleavage, and activation of caspase activated DNase (CAD), subsequently causing DNA fragmentation, which is one of characteristics of apoptosis (25, 26). Thus, the presence of DNA ladder on agarose gel is due to elevation of caspases after R. turkestanicum treatments.
This is the first report on the cytotoxic effects of R. turkestanicum in which apoptosis played an important role. It is also worth mentioning that according to the MTS results, Paclitaxel (0.7 μM) leaded to a more intense decrease in cell viability on human cancer cell line. Relatively high IC50 values may be a function of cell type used and short duration of exposure to extracts. Furthermore, anti-proliferative effects may be intensified in an in-vivo model. Further in vitro assays on some other malignant cell lines, phytochemical studies on pure compounds isolated from R. turkestanicum and in-vivo evaluations are suggested to confirm the result obtained in this study.
Acknowledgements
References
-
1.
Altmann KH, Gertsch J. Anticancer drugs from nature-natural products as a unique source of newmicrotubule-stabilizing agents. Nat. Prod. Rep. 2007;24:327-357. [PubMed ID: 17390000].
-
2.
Koehn FE, Carter GT. The evolving role of natural products in drug discovery. Nat. Rev. Drug Discov. 2005;4:206-220. [PubMed ID: 15729362].
-
3.
Cordell GA, Colvard MD. Some thoughts on the future of ethnopharmacology. J. Ethnopharmacol. 2005;100:5-14. [PubMed ID: 16009517].
-
4.
Taraphdar AK, Roy M, Bhattacharya RK. Natural products as inducers of apoptosis, implication for cancer therapy and prevention. Curr. Sci. 2001;80:10-11.
-
5.
Dorsey JF, Kao GD. Aloe (-Emodin) for Cancer? More than Just a Comforting Salve. Cancer Biol. Ther. 2007;6:89-90. [PubMed ID: 17297301].
-
6.
Venkatadri R, Gunjan G, Rangasamy AK. Antioxidant and anti-cancer potentials of Rheum emodi rhizome extracts. Evid Based Complement Alternat Med. 2010;2011. 9 pages.
-
7.
Li WY, Chan SW, Guo DJ, Chung MK, Leung TY, Fu Yu PH. H2O extract of Rheum officinaleBaill. induces apoptosis in human lung adenocarcinoma A549 and human breast cancer MCF-7 cell lines. J. Ethnopharmacol. 2009;124:251-256. [PubMed ID: 19397973].
-
8.
Eun SC, Sung DC, Jae GJ, Nam PC. The Apoptotic effect of the hexane extract of Rheum undulatum L. in oral cancer cells through the down-regulation of specificity protein 1 and survivin. Lab. Anim. Res. 2011;27:19-24. [PubMed ID: 21826155].
-
9.
Fazly Bazzaz BS, Khajehkaramadin M, Shokooheizadeh HR. In-vitro antibacterial activity of Rheum ribes extract obtained from various plant parts against clinical isolates of gram-negative pathogens. Iran. J. Pharm. Res. 2005;2:87-91.
-
10.
Chang YC, Lai TY, Yu CS, Chen HY, Yang JS, Chueh FS, Lu CC, Chiang JH, Huang WW, Ma CY, Chung JG. Emodin induces apoptotic death in Murine Myelomonocytic leukemia WEHI-3 cells in-vitro and enhances phagocytosis in leukemia mice in-vivo. Evid Based Complement Alternat Med. 2011;2011. 13 pages.
-
11.
Lee HZ. Effects and mechanisms of emodin on cell death in human lung squamous cell carcinoma. Br. J. Pharmacol. 2001;134:11-20. [PubMed ID: 11522592].
-
12.
Xin G, Xifeng L, Yinggang C, Mingqi L, Shixiong J, Xishan W. Rhein induces apoptosis of HCT-116 human colon cancer cells via activation of the intrinsic apoptotic pathway. Afr J. Biotechnol. 2011;10:13244-13251.
-
13.
Lee JI, Ha YW, Choi TW, Kim HJ, Kim SM, Jang HJ, Choi JH, Choi MH, Chung BC, Sethi G, Kim SH, Ahn KS, Choi SH, Shim BS, Ahn KS. Cellular uptake of ginsenosides in Korean white ginseng and red ginseng and their apoptotic activities in human breast cancer cells. Planta Med. 2011;77:133-40. [PubMed ID: 20669086].
-
14.
Malich G, Markovic B, Winder C. The sensitivity and specificity of the MTS tetrazolium assay for detecting the in-vitro cytotoxicity of 20 chemicals using human cell lines. Toxicology. 1997;124:179-192. [PubMed ID: 9482120].
-
15.
Parsaee H, Asili J, Mousavi SH, Soofi H, Emami SA, Tayarani-Najaran Z. Apoptosis induction of Salvia chorassanica root extract on human cervical cancer cell line. Iran. J. Pharm. Res. 2013;12:75-83. [PubMed ID: 24250574].
-
16.
Akins RE, Tuan RS. Measurement of protein in 20 sec using a microwave BCA assay. Bio. Techniques. 1992;12:496-499.
-
17.
Monasterio A, Urdaci MC, Pinchuk IV, López-Moratalla N, Martínez-Irujo JJ. Flavonoids induce apoptosis in human leukemia U937 cells through caspase- and caspase- calpain-dependent pathways. Nutr Cancer. 2004;50:90-100. [PubMed ID: 15572302].
-
18.
Shoeb M. Anticancer agents from medicinal plants. Bangladesh J. Pharmacol. 2006;1:35-41.
-
19.
Cragg GM, Newman DJ. Plants as a source of anti-cancer agents. J. Ethnopharmacol. 2005;100:72-79. [PubMed ID: 16009521].
-
20.
Berger NA, Petzold SJ. Identification of minimal size requirements of DNA for activation of poly(ADP- ribose) polymerase. Biochemistry. 1985;24:4352-4355. [PubMed ID: 3931676].
-
21.
Berger NA. Poly(ADP-ribose) in the cellular response to DNA damage. Radiat Res. 1985;101:4-15. [PubMed ID: 3155867].
-
22.
Kaufmann SH, Desnoyers S, Ottaviano Y, Davidson, NE, Poirier GG. Specific proteolytic cleavage of poly(ADP-ribose) polymerase, an early marker of chemotherapy-induced apoptosis. Cancer Res. 1993;53:3976-3985. [PubMed ID: 8358726].
-
23.
Nicholson DW, Ali A, Thornberry NA, Vaillancourt JP, Ding CK, Gallant, M, Gareau Y, Griffin PR, Labelle M, Lazebnik Y, Munday NA, Raju SM, Smulson ME, Yamin TT, Yu VL, Miller DK. Identification and inhibition of the ICE/CED-3 protease necessary for mammalian apoptosis. Nature. 1995;376:37-43. [PubMed ID: 7596430].
-
24.
Tewari M, Quan LT, O’Rourke K, Desnoyers S, Zeng Z, Beidler DR, Poirier GG, Salvesen GS, Dixit VM. Yama/CPP32 beta, a mammalian homolog of CED-3, is a CrmA-inhibitable protease that cleaves the death substrate poly(ADP-ribose) polymerase. Cell. 1995;81:801-809. [PubMed ID: 7774019].
-
25.
Soini Y, Pa¨a¨kko¨ P, Lehto VP. Histopathological evaluation of apoptosis in cancer. The Am. J. Pathol. 1998;154:1041-1053.
-
26.
Fan TJ, Han LH, Cong RS, Liang J. Caspasefamily proteases and apoptosis. Acta Biochim. Biophys. Sin. (Shanghai). 2005;37:719-727. [PubMed ID: 16270150].