Abstract
Keywords
Tinospora cordifolia (Tc) Immunomodulator Antioxidant enzymes Lymphocyte proliferation Real time PCR
Introduction
In recent times, focus on plant research has been intensified all over the world and large amount of evidence has been collected to show the immense potential of medicinal plant used in various traditional systems (1). The immune system is involved in the etiology as well as pathophysiologic mechanism of many diseases (2). The modulation of immune responses to alleviate the diseases has been of interest for many years and the concept of ‘Rasayana’ in Ayurveda is based on the related principles (3). Further immunomodulation using medicinal plants can provide an alternative to conventional chemotherapy for a variety of diseases especially when the host defense mechanism has to be acquired under the conditions of impaired immune responsiveness (4). Indian medicinal plants are rich sources of substances which are claimed to induce para-immunity, the non-specific immunomodulation of especially granulocytes, macro-phages, natural killer cells and competent functions (5).
Among the suppressive synthetic substances, cyclophosphamide has been extensively studied (6). However, the major drawback of this drug is myelosuppression, which is undesirable (7). Immunostimulation and immunosuppression both need to be tackled in order to regulate the normal immunological functioning. Therefore, the stimulatory or suppressive agents have been shown to possess activity to normalize or modulate the pathophysiological processes and are hence called immunomodulatory agents (8).
In recent years, immunostimulatory activity has been reported in a number of Ayurvedic plants like Terminalia chebula (9, 10), Curcuma longa (11), Lawsonia alba (12), and Tinospora cordifolia (5, 13-16). Tc stem is stomachic, diuretic (17), cures jaundice, skin diseases (18), etc.
Tinospora cordifolia (Tc) itself possesses a bitter, pungent and astringent taste but it acts through the virtue of its madhur (sweet) vipak. The bitter taste is said to improve metabolic activity, even at a cellular level. All the three tastes destroy toxic metabolic products and purify the tissues. Rasayana drugs with madhur vipak are considered to be immunostimulants (13). The root and stem of Tc are prescribed in combination with other drugs as an anti-dote to snake bite and scorpion sting (19), dry barks has anti-spasmodic, antipyretic (20), anti-allergic (21), anti-inflammatory (22), anti-leprotic properties (23), Immunomodulatory effect in human Immuno deficiency virus positive patients (24).
In earlier studies, the immunomodulatory activity of Tc was studied through different parameters viz. differential leukocyte count (25), phagocytic activity (26), leukocyte migration inhibition (27), delayed type hypersentivity (28), bone marrow cellularity (29), etc. Although there are many therapeutic effects, no data is available on the immunomodulatory effect of Tc stem (mango plant climber) at cellular level until now. In this context, the objective of the present study was to study the immunomodulator potential of ethanolic extract of Tc at cellular and molecular level using male rats.
Experimental
Plant material and preparation of extracts
The stems of Tc (mango plant climber) were identified by Dr G. C. Joshi (Botanist), Ranikhet, Uttarakhand, India. A voucher specimen has been preserved in the herbarium at the Pharmacognosy laboratory under the voucher specimen number of PHG/H/2132 for the future reference. Then, the stems of Tc were shade dried and coarsely powdered, extracted in soxhlet apparatus with alcohol for 32 h. The extract was then concentrated to dryness under reduced pressure using rotary evaporator at 45°C and preserved in dessicator for further use.
Animals
Male Wister rats weighing 150-180 g were procured from Laboratory Animals Resources, Division of Animal Genetics, Indian Veterinary Research Institute (IVRI), Izatnager, Bairelly, India, Reg No. CPC-196 and acclimatized to laboratory condition at Animal House, IFTM, Moradabad at room temperature with a 12 h/12 h light/dark cycle and relative humidity (60 ± 5%) and had free access to standard pellet diet; water was provided ad libitum. The Institutional Animal Ethical Committee reviewed the animal protocol prior to the experiment. All rats were treated in accordance with the guideline for the Care and Use of Laboratory Animals (NIH Publication No. 86-23, revised 1985) with the permission of Institute Animal Ethical Committee (Proposal No. 11). All experiments were carried out with strict adherence to ethical guidelines and were conducted as approved protocol by the Institutional Animal Ethics Committee (IAEC) and as Indian norms laid down by the Committee for the Purpose of Control and Supervision of Experiments on Animals (CPCSEA), New Delhi; vide approval number CPCSEA/IAEC/837/AC/04.
Animal grouping
For experimental procedure, male rats were divided into four groups of each six as below: Group I: Negative control: Rats treated with 2 mL of 1% gum acacia solution in distilled water. Group II: Positive control: Sensitized rats (through administrating 1×108 SRBCs, IP) treated with 1% gum acacia solution orally. Group III: Rats treated with cyclophosphamide 100 mg/Kg/p.o. Group IV: Sensitized rats treated with Tc ethanolic extract 100 mg/Kg/p.o.
The administrations of drugs were in the following regimens:
a) Four days prior to the sensitization (days -3, -2, -1, 0)
b) Seven days after the sensitization (days +1, +2, +3, +4, +5, +6, +7)
Preparation of sheep red blood cells (SRBC)
Blood of healthy sheep was collected from local butcher house and mixed with sterile Alsever’s solution (1 : 1). Then, it was thoroughly mixed and centrifuged at 3000 rpm for 5 min. Supernatant was discarded and SRBC pellets were washed twice with sterilized phosphate buffer saline (pH = 7.2). Then, the SRBC pellets were prepared in phosphate buffer saline (pH = 7.2), total SRBC was counted using Neubauer chamber and finally 1×108 SRBCs (0.5 mL) were injected intraperitoneally for sensitization and challenging the rats (30).
Immunological activity
The immunostimulating activity was examined through the selection of in-vivo and in-vitro immunological tests. Six hours after the last dose, the animals were sacrificed and their livers, pineal glands, spleens, kidneys, bladders and adrenal glands were separated and the following parameters were determined.
Estimation of liver mitochondrial antioxidant marker enzymes
For the isolation of mitochondria, 1 g of liver tissue was weighed and homogenized with 5 mL of 0.35 M sucrose buffer (pH = 7.0) at 4°C and centrifuged at 10,000 g for 5 min. The supernatant was discarded and resultant mitochondrial pellets were suspended in a mixture of 1 mL of 10 mM Tris-HCl (pH = 7.4) solution and 0.2 mL of 1 mM EDTA, finally volume was made up to 2 mL with 0.25 M sucrose solution (31). This solution was used for determination of various mitochondrial antioxidant enzymes.
Estimation of lipid peroxidation (LPO) by homogenization of liver tissue
After sacrificing the animals, the liver was isolated, weighed, washed with chilled ice-cold sterile 0.9% NaCl solution. Then, the tissue homogenates were prepared in a ratio of 1 g of wet tissue to 9 mL of 1.15% KCl by using a Teflon Potter-Elvehjem homogenizer (32).
Assay procedure
A volume of 0.2 mL tissue homogenate was added to the mixture of 0.2 mL of 1% SDS, 1.5 mL of 20% acetic acid solution adjusted to the pH of 3.5 with NaOH, and 1.5 mL of 0.8% aqueous solution of thiobabituric acid. The mixture was made to 4.0 mL with distilled water and then heated in an oil bath at 95°C for 60 min using a glass ball as a condenser. After cooling in tap water, 1.0 mL of distilled water, and 5.0 mL of the mixture of n-butanol and pyridine (15:1 v/v) were added to the mixture and shaken vigorously. Then, it was centrifuged at 4000 rpm for 10 min, the organic layer was taken and the absorbance at 532 nm was measured. 1, 1, 3, 3- tetramethoxypropane (TMP) was used as a standard and the level of LPO was expressed as nmol of malondialdehyde (MDA) (33).
Reduced glutathione (GSH)
Mitochondrial GSH was estimated through adding 0.2 mL of mitochondrial enzyme solution to 1.8 mL distilled water followed by the addition of 3.0 mL precipitating mixture (0.0501 g metaphosphoric acid, 0.006 g EDTA and 0.9 gm NaCl in 3 mL distilled water). It was centrifuged at 5000 g for 5 min, 1.0 mL of the supernatant was collected and 1.5 mL of the phosphate solution was added to it and then, 0.5 mL of 5,5’-Dithio-Bis (2-nitrobenzoic acid) (DTNB) reagent was added. The optical density was measured at 412 nm spectrophotometrically (Schimadzu 1601). The absorbance of reduced GSH was noted and represented as percentage μMol/gm Hb (34).
Catalase (CAT)
A volume of 0.2 mL mitochondrial enzyme solution was added to a cuvette containing 2.0 mL of phosphate buffer (pH = 7.0) and 1.0 mL of 30 mM H2O2. Catalase activity was measured at 240 nm for 1 min using spectrophotometer. The molar extinction coefficient of H2O2, 43.6 M cm-1, was used to determine the catalase activity. One unit of activity is equal to 1 mmol of H2O2 degraded per minute and is expressed as units/mg of protein (35).
Superoxide dismutase (SOD)
Fifteen μL of the mitochondrial enzyme solution was added to a mixture of 0.5 mL of 75 mM Tris-HCl buffer (pH = 8.2), 1 mL of 30 mM EDTA and 1 mL of 2 mM pyrogallol. The absorbance was recorded at 420 nm for 3 min using spectrophotometer. One unit of enzyme activity is calculated as the 50% inhibition of pyrogallol autooxidation rate as determined through the change of absorbance/min at 420 nm. The activity of SOD is expressed as units/mg protein (36).
SDS-PAGE
Protein fractions were isolated from the pineal glands of animals from each group and subjected to the SDS-PAGE analysis for the detection of melatonin secretion. Therefore, the resolving gel mixture and the stacking gel mixtures used were 12% and 5% respectively. The gels were stained using Coomassie Brillant Blue R-250 solution for 4 h. Further gels were kept in destaining solution for 8 h until the background gets colourless. Finally, after taking the photographs, the gels were stored in distilled water containing 20% glycerol (37).
Lymphocyte proliferation assay
Splenocyte single cell suspension was prepared through up-downing 4 mL of RPMI-1640 in spleen and after omitting RBCs using 0.75% NH4Cl in Tris buffer (0.02%, pH = 7.2) (adding 6 mL buffer to 2 mL cell suspension after 3 min of centrifugation at 1000 g for 2 min). The concentration was adjusted to 2×106 Cells/mL in RPMI-1640 supplemented by 10% fetal calf serum, 2 mM L-Glutamine, 25 mM (4-(2-hydroxyethyl)-1-piperazineethanesulfonic acid) (HEPES). A hundred μL of diluted cell suspension was dispensed into a 96-well flat bottom culture plate. Mitogen phytohemmaglutinin A (PHA) was added at 5 μg/mL of final concentration to each well and the volume was adjusted to 0.2 mL. After incubating for 72 h at 37ºC and 5% CO2 humid atmosphere air, the cell proliferation was determined using MTT assay method (37). The 10% of (3-(4, 5-dimethyl-2-thiazolyl) 2,5-diphenyl-2H-tetrazolium) (MTT) (5 mg/mL) was added to each well and the plates were incubated at 37ºC in CO2 humid atmosphere for 4 h. The blue formazan precipitate was dissolved in acidic isopropanol and its optical density was measured at 570 nm using Elisa Reader. The stimulation index was calculated as follows (39):
Stimulation index = Optical density of stimulated cells/Optical density of unstimulated cells.
Real-time PCR for the determination of IL-2, IL-10 and TNF-α expression in spleen cells
Spleen cell suspensions were prepared and used for the determination of cytokines such as IL-2, IL-10 and TNF-α. The sequences of primers used in RT-PCR are given in Table 1.
The sequences of primers used in RT-PCR.
Primer | Sequence (5’ to 3’) | Length (bases) |
---|---|---|
IL-10Rat-F | ACCAGCTGGACAACATACTGCTGA | 24 |
IL-10Rat-R | CCTTGATTTCTGGGCCATGGTTCT | 24 |
IL-2Rat-F | CTGCAGCGTGTGTTGGATTTGACT | 24 |
IL-2Rat-R | TTGCTGGCTCATCATCGAATTGGC | 24 |
BactRat-F | TGAGAGGGAAATCGTGCGTGACAT | 24 |
BactRat-R | ACCGCTCATTGCCGATAGTGATGA | 24 |
TNF Rat-F | CTGGCCAATGGCATGGATCTCAAA | 24 |
TNF Rat-R | ATGAAATGGCAAATCGGCTGACGG | 24 |
RT-BGF | CATGTTTGTGATGGGCGTGAACCA | 24 |
RT-BGR | TAAGTCCCTCCACGATGCCAAAGT | 24 |
RNA isolation
Trizol LS reagent (Invitrogen cat No. 10296-010) was used for the RNA isolation and the method prescribed by the manufacturer was followed. Briefly, spleen cells were washed with PBS lysed through adding Trizol LS reagent onto them and repeated pipetting. The organic and aqueous phases were separated with the help of chloroform and centrifugation. Total RNA from the aqueous phase was precipitated with isopropyl alcohol, washed in ethanol, air dried and diluted in nuclease free water.
cDNA preparation
Revert Aid M-MuLV Reverse Transcriptase (Fermentas cat No. EP0441) was used for this purpose. About 100 ng of RNA and 0.5 μg of oligo dT (13-19) primer (Invitrogen cat No. 18418-012) were mixed and snap chilled. Four μL of 5× reaction buffer, 20 U of RNase inhibitor, 2 μL of 10 mM dNTP and 200 U of Revert Aid M-MuLV Reverse Transcriptase were added to this mixture. The final reaction volume was kept 20 μL. The reaction mixture was incubated at 42°C for 1 h. The reaction was then stopped through heating the mixture at 70ºC for 10 min. The cDNA was divided into aliquots of suitable size and stored at -20°C (40).
Real-time PCR
Brilliant SYBR Green QPCR Core Reagent Kit (Stratagene cat No. 600546) was used for this purpose. One μL of cDNA was added to 11 μL of nuclease free water, 2.5 μL of 10×core PCR buffer, 1.25 μL of 50 mM MgCl2 solution, 1 μL of 20 mM dNTP mix, 1 μL (12.5 pM/μL) of forward primer, 1 μL (12.5 nM/μl) of reverse primer, 4 μL of 50% glycerol solution, 0.75 μL of 100% DMSO, 1.25 μL of 1:3000 diluted SYBR Green I dye and 0.25 μL (1.25 U) of SureStart Taq DNA polymerase. Real-time PCR was carried out in M×3000p (Stratagene). The thermal profile used for this was as follows: 95ºC for 10 min then 40 cycles of 95ºC for 30 sec, 64ºC for 30 sec and 72°C for 30 sec with fluorescence recording at the end of each cycle, followed by denaturation of products from 55ºC to 95ºC with fluorescence recording throughout the step. Fold changes in target transcript levels were determined using reported method (40), considering Etarget = Eref = 2. The GAPDH gene was used as a reference.
Statistical analysis
The results were expressed as mean ± SD and the statistical evaluation of the data was done using the prism software (version 5.00) and Student’s t-test. P-values less than 0.05 were considered as significant.
Results and Discussion
Liver mitochondrial enzyme estimation
Table 2 shows the increased lipid peroxidation level in liver (98.38 ± 1.14 MMDA/g Hb) in SRBC-treated group as compared to the vehicle-treated rat (83.85 ± 2.12 MMDA/g Hb). Furthermore, cyclophosphamide-treated rats showed slight increase (90.42 ± 2.13 MMDA/g Hb) in the lipid peroxidation level in the liver and after the treatment of Tc ethanolic extract, the elevated lipid peroxidation was decreased to 62.52 ± 1.13 ± 1.16 MMDA/g Hb.
Effect of Tc on rat’s liver mitochondrial enzymes
Treatment group | Liver Mitochondrial Enzymes | |||
---|---|---|---|---|
LPO (MMDA/g Hb) | GSH (μMol/g Hb) | SOD (units/mg protein) | Catalase (units/mg protein) | |
Vehicle | 83.85 ± 2.12 | 4.22 ± 0.21 | 22.22 ± 0.10 | 271.31 ± 3.2 |
SRBC sensitized | 98.38 ± 1.14 | 2.13 ± 0.18 | 19.48 ± 0.16 | 252.22 ± 2.5 |
Cyclophosphamide | 90.42 ± 2.13 | 2.31 ± 0.38 | 20.38 ± 0.18 | 256.29 ± 3.8 |
Tc | 62.52 ± 1.13* | 4.82 ± 0.31* | 25.32 ± 0.14* | 278.41 ± 9.2* |
The levels of reduced glutathione, superoxide dismutase and catalase were decreased through the SRBC sensitization and were increased via the treatment with Tc ethanolic extract. The levels of reduced glutathione, superoxide dismutase and catalase, treated by SRBC sensitization and Tc ethanolic extract, were 2.13 ± 0.18 and 4.82 ± 0.31 μMol/g Hb, 19.48 ± 0.16 and 25.32 ± 0.21 units/mg protein and 252.22 ± 2.5 and 278.41 ± 9.2 units/mg protein respectively. Cyclophosphamide showed immunosupression through increasing the lipid peroxidation level and decreasing the level of reduced glutathione, superoxide dismutase and catalase as compared with the control group.
The oxidation of membrane’s lipid molecules damages the development of several physiological and pathological disorders. The inhibition of lipid peroxidation by any means is the best way to avoid these disorders in the body (41). Present study showed the effect of Tc ethanolic extract on lipid peroxidation which was inhibitorier as compared with SRBC and cyclophosphamide-treated groups.
Glutathione is the major endogenous antioxidant produced by the cells, participating directly in the neutralization of free radicals and reactive oxygen compounds, as well as maintaining the exogenous antioxidants such as vitamins C and E in their reduced (active) forms. Through the direct conjugation, it detoxifies many xenobiotics (foreign compounds) and carcinogens, both organic and inorganic. It is essential for the immune system to exert its full potential viz. modulating the antigen presentation to lymphocytes, and thereby, influencing the cytokine production; enhancing the proliferation of lymphocytes thereby increasing magnitude of response; enhancing the killing activity of cytotoxic T-cells and natural killer cells and regulating apoptosis thereby maintaining the control of immune response. Glutathione plays a fundamental role in numerous metabolic and biochemical reactions such as DNA synthesis and repair, protein synthesis, prostaglandin synthesis, amino acid transport and enzyme activation. Thus, every system in the body can be affected through the state of glutathione system, especially the immune system, the nervous system, the gastrointestinal system and the lungs (42-45).
Superoxide dismutase induces the activation of endogenous system of the antioxidant defenses which fights against free radicals (46). It is known that superoxide dismutase plays an important role in the detoxification of superoxide anion and H2O2, and thereby promotes the cell against the free radical-induced damage (47).
Catalase is an enzymatic antioxidant and helps in neutralizing the toxic effect of H2O2. Hydrogen peroxide is not reactive enough to cause a chain of lipid peroxodation reactions, but its combination with superoxide radical produces hydroxyl radicals that are highly reactive and thus initiates the lipid peroxidation reaction (48). This lipid peroxidation damages the cell membrane resulting into the development of several physiological and pathological disorders. Catalase prevents this through the conversion of hydrogen peroxide to water and non-reactive oxygen species and thus, prevents from the generation of hydroxyl radical and protect the cell from the oxidative damage (49, 50). Our studies showed distinct increase in the level of glutathione, superoxide dismutase and catalase in case of Tc-treated groups as compared to SRBC and cyclophosphamide-treated groups. Therefore, Tc has antioxidant activity as well as immunomodulator property through protecting the cells from the oxidative damage.
Separation of pineal proteins through SDS-PAGE
A thick band at 248 kDa appeared in the group treated with Tc ethanolic extract indicating higher secretion of melatonin (Mol. Wt. 248 kDa) (lane 2). In SRBC-sensitized group, the expression of proteins (lane 4) was less than that of control group (lane 3). The immunosuppressant cyclophosphamide showed the least expression of proteins as compared to other groups (lane 5). The increased expression of melatonin in lane 2 confirms the immunomodulator activity of Tc (Figure 1).
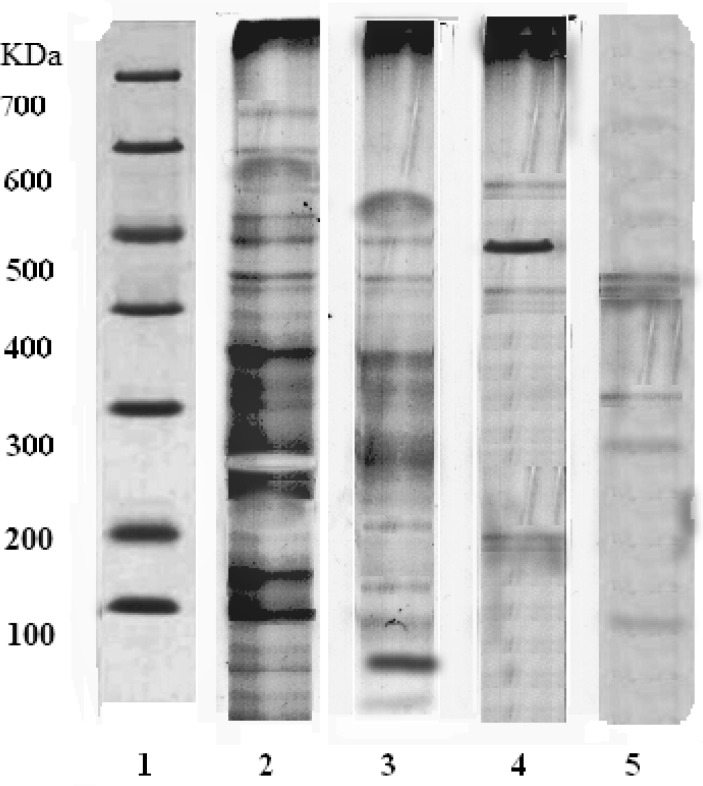
Melatonin plays an important role as immunomodulatory (51-53) properties, as well as many other physiological functions (54). Melatonin was reported to be an integral part of the immune system, through exerting direct and/or indirect stimulatory effect on both cellular and humoral immunity (51). The present study showed that Tc treatment shows the thick band of protein expression indicating the increased production of the melatonin as compared to SRBC sensitized, vehicle and cyclophosphamide-treated groups. Hence, Tc exhibits immunostimulant action through enhancing melatonin secretion in pineal gland.
Lymphocyte proliferation assay
The administration of Tc-ethanolic extract increased the spleen lymphocytes proliferation (1.428 ± 0.361) as compared to the vehicle-treated group (0.787 ± 0.256) and cyclophosphamide-treated rats (0.832 ± 0.471) (Table 3). Phytohaemagglutinin used as a standard shows proliferation of lymphocytes in all groups.
Proliferation of rat spleen lymphocytes in response to antigen prepared from Tc ethanolic extract using MTT dye reduction test
Treatment | Stimulation index | Phytohaemagglutinin-stimulated |
---|---|---|
Vehicle | 0.787 ± 0.256 | 1.222 ± 0.841 |
Cyclophosphamide | 0.832 ± 0.471 | 0.936 ± 0.412 |
Tc | 1.428 ± 0.361* | 1.386 ± 0.361* |
The earlier works have revealed that drugs having immunomodulatory activity show enhanced proliferation of splenocytes (55). In present study, Tc was found to significantly enhance the proliferation of splenocytes as compared with control and cyclophosphamide treatment. The proliferation of lymphocytes indicated the increase in the number of B and T cells, which release cytokines and growth factors that regulate other immune cells and secretion of antibodies in the blood (56).
Comparison of IL-2, IL-10 and TNF-α gene expression in rat spleen mRNA
Measuring and assessing cytokine profile provides a useful method for the accurate study of cytokine such as IL-2, IL-10 and TNF-α and immunomodulatory effect was investigated through RT-PCR. Tc treatment showed more fold changes in IL-2, IL-10 and TNF-α mRNA (17.63, 42.52 and 5.39 respectively) levels as compared to the vehicle, cyclophosphamide and SRBC sensitized groups as shown in Table 4. Increased expression of fold change in different mRNA levels using Tc confirms the immunostimulant action.
Effect of different treatments on IL-2, IL-10 and TNF-α gene expression
Treatment | Fold change in IL-2, IL-10 and TNF- α mRNA level | ||
---|---|---|---|
IL-2 | IL-10 | TNF-α | |
Vehicle | 1.00 | 1.00 | 1.00 |
Cyclophosphamide | 6.41 | 8.63 | -1.78 |
Tc | 17.63 | 42.52 | 5.39 |
SRBC sensitized | -2.87 | 22.63 | 1.18 |
Cytokines are released by living cells of the host in a highly regulated fashion to regulate the cell functions via specific receptors (57) which participate in the control of all immunologically relevant events, whether they concern the activation, differentiation, maturation, proliferation, apoptosis, or acquisition of effectors’ functions. Cytokines influence the quantitative, as well as the qualitative outcome of all the immune responses (58). In our study, after the administration of ethanolic extract of Tc in male Wister rats, significant immunomodulatory activities were shown through fold changes in IL-2, IL-10 and TNF-α mRNA levels. IL-2 shows immunomodulatory activities by T and B lymphocytes proliferation, natural killer cell activation (59, 60) and also increasing in the immunoglobulin G production whereas IL-10 promotes elevation of Th2 cells (58, 60) and TNF-α modulate the cytokine gene expression (59). Hence, the immunomodulatory activity of Tc may be due to the above mechanism(s).
Conclusion
From the above results, it can be concluded that Tc stem (mango plant climber) has potent immunomodulatory activity and the effect may be due to the specific chemical constituents or the synergistic effect of chemical compounds of the drug.
Acknowledgements
References
-
1.
Dahanukar SA, Kulkarni RA, Rege NN. Pharmacology of medicinal plants and natural products. Indian J. Pharmacol. 2000;32:S81-S118.
-
2.
Liu Y, Jiao F, Qiu Y, Li W, Lao F, Zhou GQ, Sun BY, Xing GM, Dong JQ, Zhao YL, Chai ZF, Chen CY. The effect of Gd@C82(OH)22 nanoparticles on the release of Th1/Th2 cytokines and induction of TNF-α mediated cellular immunity. Biomaterials. 2009;30:3934-3945. [PubMed ID: 19403166].
-
3.
Sharma P, Charaka Samhita. Chikitsasthana. India: Varanasi Chaukhamba Orientalia; 1983. 2 p.
-
4.
Lily G, Padwad Y, Singh R, Karan D, Chanda S, Chopra MK, Bhatnagar P, Kashyap R, Sawhney RC. Antiinflammatory activity of seabuckthorn (Hippophae rhamnoides) leaves. Int. J. Immunopharmacol. 2005;5:1675-1684.
-
5.
Sainis KB, Sumariwalla PF, Goel A, Chintalwar GJ, Sipa- himalani AT. Banerji A Immunomodulatory properties of stem extracts of Tinospora cordifolia: cell targets and active principles. In: Upadhyay SN, editor. Immunomodulation. New Delhi: Narosa Publishing House; 1997. 95 p.
-
6.
Shand FL, Howard JG. Induction in-vitro of reversible immunosuppression and inhibition of B cell receptor regeneration by defined metabolites of cyclophosphamide. Eur. J. Immunol. 1979;9:17-21. [PubMed ID: 374091].
-
7.
Hardman JG, Limbird LE, Molinoff PB, Ruddon RW, Gilman AG. Alkylating agents. In: New York Academy of Sciences, editor. Goodman and Gilman’s the Pharmacological Basis of Therapeutics. New York: McGraw-Hill; 1996. p. 1216-1218.
-
8.
Wagner H. Immunomodulatory agents. Proc. Alfred Benzon Symp. 1983;20:559.
-
9.
Chattopadhyay RR, Bhattacharyya SK. Terminalia chebula: an update pharmacognosy reviews. Phcog. Rev.: Plant Review. 2007;1:151-157.
-
10.
Prakash SS, Niranjan Patra CH, Santanu CH, Hemant Kumar P, Jagannath Patro V, Vimala Devi V. Studies on flowability, compressibility and in-vitro release of Terminalia chebula fruit powder tablets. Iranian J. Pharm. Res. 2011;10:393-401.
-
11.
Deodhar SD, Sethi R, Srimal RC. Preliminary study on antirhematic activity of curcumin (diferuloyl methane). Ind. J. Med. Res. 1980;71:632-634.
-
12.
Dev S. Ethanotherapeutics and modern drugs development: The potential of Ayurveda. Curr. Sci. 1997;73:909-928.
-
13.
Dahanukar SA, Thatte UM, Rege NN. Immunostimulants in Ayurveda medicine. In: Wagner H, editor. Immunomodulatory Agents from Plants. Basel: Berkhauser Verlag; 1999. p. 289-323.
-
14.
Thatte UM, Rao SG, Dhanukar SA. Tinospora cordifolia induces colony stimulating activity in serum. J. Postgrad. Med. 1994;40:202-203. [PubMed ID: 9136239].
-
15.
Sainis KB, Ramakrishnan R, Sumariwalla PF, Goel A, Sipahimalani AT, Banerji A. Further studies on immunomodulation by natural products from Tinospora cordifolia. In: Upadhyay SN, editor. Immunopharmacology: Strategies for Immunotherapy. India: Narosa Publishing House; 1999. p. 96-104.
-
16.
Aher Vaibhav D, Wahi Arunkumar. Pharmacological study of Tinospora cordifolia as an immunomodulator. Int. J. Curr. Pharma. Rev. 2010;2:52-54.
-
17.
Nayampalli SS, Ainapure SS, Samant BD, Kudtarkar RG, Desai NK, Gupta KC. A comparative study of diuretic effects of Tinospora cordifolia and hydrochloro-thiazide in rats and a preliminary phase I study in human volunteers. J. Postgrad. Med. 1988;34:233. [PubMed ID: 3254990].
-
18.
Raghunathan K, Mittra R, editors. Pharmacognosy of Indigenous Drugs. New Delhi: Central Council for Research in Ayurveda adn Siddha; 1982. p. 484-510.
-
19.
Zhao TF, Wang X, Rimando AM, Che C. Folkloric medicinal plants: Tinospora sagittata var. cravaniana and Mahonia bealei. Planta Med. 1991;57:505. [PubMed ID: 1798808].
-
20.
Ikram M, Khattak SG, Gilani SN. Antipyretic studies on some indigenous Pakistani medicinal plants: II. J. Ethnopharmacol. 1987;19:185-192. [PubMed ID: 3497307].
-
21.
Nayampalli SS, Desai NK, Ainapure SS. Anti-allergic properties of Tinospora cardifolia in animal models. Indian J. Pharm. 1986;18:250-252.
-
22.
Rai M, Gupta SS. The deposition of the secondary salts over the five pellets in rats was inhibited by the aqueous extract of T. cordifolia. J. Res. Ind. Med. 1966;10:113-116.
-
23.
Asthana JG, Jain S, Mishra A, Vijaykanth MS. Evaluation of antileprotic herbal drug combinations and their combination with Dapsone. Indian Drugs. 2001;38:82-86.
-
24.
Thawani VR, Kalikar MV, Varadpande VK, Sontakkee SD, Singh RP, Khiyani RK. Immunomodulatory effect on T. cordifolia in human Immunodeficiency virus positive patients. Indian J. Pharmacol. 2008;40:107-110. [PubMed ID: 20040936].
-
25.
Sheela D, Ramasundaram S, Jaya PN. Immunomodulatory activity of triphala on neutrophil functions. Biol. Pharm. Bull. 2005;28:1398-1403. [PubMed ID: 16079482].
-
26.
Furthvan R, Bergvanden BM. Clinical Immunology. London: Gower Medical Publishing; 1991. 67 p.
-
27.
Sorberg M. Bendisen G. Human leukocyte migration as a parameter of hypersensitivity. Acta Med. Scand. 1967;18:247-252.
-
28.
Benencia F, Courreges M, Cecialia C, Felix C. In-vivo and in-vito immunomodulatory activities of Trichilia glabra aqueous leaf extracts. J. Ethnopharmacol. 2000;69:199-205. [PubMed ID: 10722201].
-
29.
Sredni B, Albeck M, Kazimirsky G, Shalet F. Immunomodulator AS101 administered orally as a chemoprotective and radioprotective agent. Int. J. Immunopharmacol. 1992;14:613-619. [PubMed ID: 1521929].
-
30.
Patel S, Basnji D, Otilia JF, Patel MM, Shah KK. Scrutinizing the role of aqueous extract of Trapa bispinosa as an immunomodulator in experimental animals. Int. J. Res. Pharm. Sci. 2010;1:13-19.
-
31.
Jonshon D, Lardy H. Isolation of liver, kidney mitochondria. Methods in Enzymology. 1947;10:94-96.
-
32.
Hogeboom GH. Fractionation of cell components of animal tissues. In: Colowick SP, Kaplan NO, editors. Methods in Enzymology. New York: Academic Press; 1955. p. 16-19.
-
33.
Ohkawa H, Ohishi N, Yagi K. Assay for lipid peroxidation in animal tissue by Thiobarbituric acid reaction. Anal. Biochem. 1979;95:351-358. [PubMed ID: 36810].
-
34.
Ellman GL. Tissue sulphydryl group elucidation of the biologically active components of Terminalia chebula Retzius. Arch. Biochem. Biophys. 1959;82:70-77. [PubMed ID: 13650640].
-
35.
Aebi H. Catalase in-vitro. Methods Enzymol. 1984;105:121-126. [PubMed ID: 6727660].
-
36.
Marklund S, Marklund C. Involvement of the superoxide anion radical in the autooxidation of pyrogallol and a convenient assay for superoxide dismutase. Eur. J. Biochem. 1984;7:469-474.
-
37.
Sambrokk J, Russell D. Molecular Cloning. A Laboratory Manual (3rd ed). 2000;3:40-49.
-
38.
Mosmann TR, Coffman RL. Th1 and Th2 cells. Different pattern of lymphokines secretion lead to different functional properties. Ann. Rev. Immunol. 1989;7:145-173.
-
39.
Singh GK, Kumar P, Chauhan RS, Singhal LK. Cow urine upregulates lymphoblastogenesis in chicks. ISAH. Warsaw, Poland. 2005;2:90-92.
-
40.
Pfaffi MW. A new mathematical model for relative quantification in Real-Time RT-PCR. Nucleic Acids Res. 2001;29:2003-2007. [PubMed ID: 11353068].
-
41.
Janero DR. Malonyldialdehyde and thiobarbituric acid reactivity as diagnostic indices of lipids peroxidation and peroxidation tissue injury. Free Radic. Biol. Med. 1990;9:515-540. [PubMed ID: 2079232].
-
42.
Kinscheref R. Effect of glutathione depletion and oral n-acetyl-cysteine treatment on CD4+ and CD8+ cells. FASEB J. 1994;8:448-451. [PubMed ID: 7909525].
-
43.
Wu D. In-vitro glutathione supplementation enhances interleukin-2 production and mitigenic response of peripheral blood mononuclear cells from young and old subjects. J. Nutr. 1994;124:655-663. [PubMed ID: 8169657].
-
44.
Fidelus M. Glutathoine and lymphocyte activation: a function of aging and auto immune disease. Immunol. 1987;61:503-508.
-
45.
Buhl R. Systemic glutathione deficiency in symptom free HIV-1 seropositive individuals. Lancet. 1989;334:1294-1298. [PubMed ID: 2574255].
-
46.
Bourg M, Joanny F. Superoxide dismutase, a powerful antioxidant, is now available orally. Phytotherapie. 2005;3:1-4.
-
47.
Goyal BR, Mahajan S. Mali R. Ramesh GK , Anita MA. Beneficial effect of Achyranthes apsers Linn. in toluene di-isocynate induced occupational asthama in rats. Global J. Pharmacol. 2007;1:6-12.
-
48.
Lubac B, Hayr M, DenkW, Bauer G. Brain lipid peroxidation and hydroxyl radical attack following the intravenous infusion of hydrogen peroxide in an infant. Free Radic. Biol. Med. 1996;21:219-223. [PubMed ID: 8818637].
-
49.
Chelikani P, Fita I, Loewen P. Diversity of structures and properties among catalases. Cell Mol. Life Sci. 2004;61:192-208. [PubMed ID: 14745498].
-
50.
Zamocky M, Koller F. Understanding the structure and function of catalases: clues from molecular evolution and in-vitro mutagenesis. Prog. Biophys. Mol. Biol. 1999;72:19-66. [PubMed ID: 10446501].
-
51.
Guerrero JM, Reiter RJ. Melatonin-immune system relationships. Curr. Top. Med. Chem. 2002;2:167-179. [PubMed ID: 11899099].
-
52.
Carrillo-Vico A, Garcia-Maurino S, Calvo JR, Guerrero JM. Melatonin counteracts the inhibitory effect of PGE2 on IL-2 production in human lymphocytes viaits mt1 membrane receptor. FASEB J. 2003;17:755-757. [PubMed ID: 12594180].
-
53.
Carrillo-Vico A, Lardone PJ, Fernandez-Santos JM, Martin-Lacave I, Calvo JR, Karasek M, Guerrero JM. Human lymphocyte-synthesized melatonin is involved in the regulation of the interleukin-2/interleukin-2 receptor system. J. Clin. Endocrinol. Metab. 2005;90:992-1000. [PubMed ID: 15562014].
-
54.
Sallinen P, Saarela S, Ilves M, Vakkuri O, Leppaluoto J. The expression of MT1 and MT2 melatonin receptor mRNA in several rat tissues. Life Sci. 2005;76:1123-1134. [PubMed ID: 15620576].
-
55.
Kijjoa A, Amat N, Upur H, Ablimit A, Matsidik A. Immunomodulatory effect of Abnormal Savda Munsiq, a traditional Uighur medicine, on the combined stress mice. J. Ethonopharmacol. 2009;122:42-47.
-
56.
Berrington JE, Barge D, Fenton AC, Can AJ, Spickett GP. Lymphocyte subsets in term and significantly preterm UK infants in the first year of life analysed by single platform flow cytometry. Clin. Exp. Immunol. 2005;140:289-292. [PubMed ID: 15807853].
-
57.
Kroemer G, Alboran M, Gonzalo JA, Martinez C. Immunoregulation by cytokines. Crit. Rev. Immunol. 1993;19:163-191. [PubMed ID: 8352909].
-
58.
Kresina F. Thomas Immune Modulating Agents. New York: Marcel Dekker; 1998. p. 1-19.
-
59.
Kroemer GJ, Anderu-Sanchez L, Gonzalo JA, Gutierrez-Ramoz JC, Martinez AC. Interleukin-2 autotolerance and autoimmunity. Adv. Immunol. 1991;150:147-235. [PubMed ID: 1950796].
-
60.
Plaut M, Pierce JH, Watson CJ, Hanley-Hyde Nordan RP, Paul WE. Mast cell lines produce lymphokines in response to cross-linkage of FcRI or to calcium ionophores. Nature. 1989;339:64.