Abstract
Keywords
Apoptosis Hippocampus Memory Omega-3 fatty acids Scopolamine
Introduction
Male Wistar rats weighing 240-300 g were housed at controlled temperature (22°C ± 2°C) on a 12 h alternating light-dark cycle with free access to water and a standard commercial diet with fixed value of carbohydrate, protein, fat, fiber and vitamins plus minerals (660, 230, 40, 60 and 10 mg/Kg, respectively; Nuvital Nutrients, Curitiba, Parana, Brazil). All experimental procedures were conducted in accordance with the NIH Guide for the Care and Use of Laboratory Animals.
Treatments and surgical procedure
The drugs used in the present study were scopolamine (Tocris Cookson Ltd., UK), a standardized omega-3 fatty acids formulation, contained 120 mg of DHA and 180 mg of EPA per g (Zahravi Pharmaceutical Company, Tehran, Iran) and for surgical procedures, ketamine and xylazine were used (Alfasan Chemical Co, Woerden, and Holland). Omega-3 fatty acids [60 mg/Kg (DHA: 24 mg/Kg + EPA: 36 mg/Kg)] was administered through gavage in a constant volume of 1 mL for 2 or 8 weeks. Scopolamine (2 µg/rat as 1 µg in each side) was injected bilaterally into the dorsal hippocampal CA1 region in a volume of 1 μL/rat.
Animals were anesthetized with intra-peritoneal injection of ketamine hydrochloride 10% (50 mg/Kg) plus xylazine 2% (4 mg/Kg) and placed in a stereotaxic apparatus. A midline incision was made and the skin and underlying periosteum retracted to expose the rat skull. Bilateral stainless-steel, 22-gauge guide cannulas were placed streotactically 1 mm above the intended site of injection according to the atlas of Paxinos and Watson 2007. Stereotaxic coordinates for the CA1 region of dorsal hippocampus were incisor bar (-3.3 mm), -3 to -3.5 mm (depending on the body weight) posterior to bregma, ±1.8 to 2 mm lateral to the sagittal suture and -2.8 to -3 mm ventral to the dorsal surface of the skull. Cannulas were secured to anchor jewelers’ screws with acrylic dental cement. After completing the surgery, two stainless steel stylets (27-gauge insect pins) were inserted into the guide cannulas to keep them free from debris. After a brief anesthetic clearing period, all animals were allowed to recover from surgery for one week.
The animals were gently restrained by hand; the stylets were removed from the guide cannula and replaced by 27-gauge injection needles (1 mm below the tip of the guide cannula). The injection’s solutions were administered manually in a total volume of 1 µL/rat (0.5 µL in each side) and then animals were immediately placed in their home cages.
Passive avoidance test
The step-through inhibitory avoidance apparatus box (Borj Sanat Co, Tehran, Iran) is comprised of two equal compartments, one light (white opaque resin, 20 cm × 20 cm × 30 cm) and one dark (black opaque resin, 20 cm × 20 cm × 30 cm) separated by a guillotine door (7 × 9 cm). Stainless steel grids (2.5 mm in diameter and at 1 cm intervals) were installed on the floor of the dark compartment to produce foot shock. Intermittent electric shocks (50 Hz, 3 s, and 1 mA intensity) were conveyed to the grid floor of the dark compartment by an insulated stimulator.
Training was based on our previous studies (14). Following 30 min of habituation, each animal was gently placed in the light compartment of the apparatus. Five sec later, the guillotine door was opened allowing the animal to enter the dark compartment. The time latency of animal crossing from the light to the dark compartment was recorded and rats with an elapse of more than 100 sec were excluded from the experiments. Once entering the dark compartment, the door was closed and an electrical foot shock (50 Hz, 1 mA and 3 s) was delivered through the stainless steel rods. The training was terminated after the rat remained in the light compartment for a 120 sec period. The number of trials (entries into the dark chamber) was recorded. All the animals were trained with a maximum of 3 trials.
Twenty-four h after the training, a retrieval test was carried out to determine the long-term memory formation. Each animal was placed in the light compartment for 20 sec, then the door was opened and the step-through latency for entering the dark compartment was measured. The cut-off time of 300 sec was applied for those animals which still remained in the light compartment. During these sessions, no electric shock was applied.
Locomotor activity was measured with an activity meter (Borj Sanat Co, Tehran, Iran). On the test day and immediately after the memory retrieval measurement, the animals were placed in the floor of a clear Perspex container (30×25×20 cm high). Horizontal movements were recorded for 5 min by sensors installed beneath the container floor. The number of sensor activations was used as the indicator of animals’ locomotor activity.
Forty-eight rats were randomly divided into 4 groups of 12. Before any pre-training injections, behavioral test and other evaluations, omega-3 fatty acids (60 mg/Kg) were administered through gavage in 2 groups of rats for 14 or 56 days (Table 1). Control groups received diluted solution (saline) without DHA or EPA for 14 or 56 days (Table 1). Scopolamine (2 µg/rat) was injected bilaterally into dorsal hippocampus (CA1 region) in a volume of 1 μL/rat in all groups. Table 1 shows the mentioned designations in detail.
Avoidance response with pre-training administration of scopolamine started immediately after the termination of omega-3 or saline gavage.
Experimental groups and treatments
Groups | N | Weeks of treatment | Gavage | Pre-training injection | Training | 24 h | Behavioral Test | Tissue sample |
---|---|---|---|---|---|---|---|---|
Saline (2) + Scopolamine | 12 | 2 | Saline | Scopolamine | * | - | * | * |
Saline (8) + Scopolamine | 12 | 8 | Saline | Scopolamine | * | - | * | * |
ω3 (2) + Scopolamine | 12 | 2 | ω3 | Scopolamine | * | - | * | * |
ω3 (8) + Scopolamine | 12 | 8 | ω3 | Scopolamine | * | - | * | * |
Biochemical analysis
Rats were killed following the passive avoidance test. The brain was rapidly removed and stored at -80°C. Both hippocampuses were carefully separated in physiologic serum. One hippocampus of each rat was homogenized in homogenization buffer (12.5 mM sodium phosphate buffer with pH of 7.0, 400 mM NaCl), followed by centrifugation at 1000 × g for 10 min at 4°C. The resulted supernatant underwent biochemical analysis.
Malondialdehyde (MDA) was measured as an index of lipid peroxidation level in hippocampal tissue. To measure MDA, the method of thiobarbituric acid was used (15), in which MDA reacts with thiobarbituric acid (TBA) to produce a red color with the highest absorbance at 532 nm [Northwest Life Science Specialties LLC (NWLSSTM), Malondialdehyde Assay Kit, sensitivity = 0.08 µmol].
Catalase (CAT) and Superoxide Dismutase (SOD) activities were determined using the commercial kits [Catalase Activity Assay Kit (abcam: ab83464) and Superoxide Dismutase Activity Colorimetric Assay Kit (abcam: ab65354)]. CAT activity was measured through a spectrophotometric assay of hydrogen peroxide based on the formation of its yellow stable complex. Catalase first reacted with H2O2 to produce water and oxygen and the unconverted H2O2 reacted with OxiRed probe to produce a product measured by colorimetric method at 570 nm. SOD activity was determined by using xanthine oxidase method based on O2•− generation. The rate of the reduction with a superoxide anion is linearly related to the xanthine oxidase (XO) activity and is inhibited with SOD. The inhibition activity of SOD was determined by colorimetric method. The SOD and CAT activities were expressed as units per mg tissue protein (U/mg protein).
Western blotting for apoptotic or anti-apoptotic protein expression
The hippocampus from other hemisphere was placed in the solubilization buffer [sodium dodecyl sulfate (2.5%), glycerol (10%), Tris-HCl (62.5 mmol/L, pH = 6.8), and 2-mercaptoethanol (5%)] and boiled for 10 min. The whole extracted tissue was stored at -70°C and the protein concentration was measured using a bicinchoninic acid protein assay (absorbance = 560 nm, Pierce). The equal amount of protein per sample (50 μg) was applied on a 12% sodium dodecyl sulfate polyacrylamide gels with 4.5% stacking gel. After having a rinse in deionized water, the membrane was incubated at 4°C in 0.1 mol/L sodium phosphate buffer (pH = 7.4) and then incubated for 2 h with a 1:3500 dilution of rabbit polyclonal anti-rat Bcl-2 and Bax antibodies [Abcam: Bcl2 antibody and Bax antibody]. Membranes were incubated with HRP-conjugated secondary antibody [Abcam: Rabbit IgG secondary antibody - H and L - Pre-Adsorbed] after three washes. The bands were finally visualized with the ECL chemiluminescence system (Amersham) and the film was developed and used for the measurement of optical density.
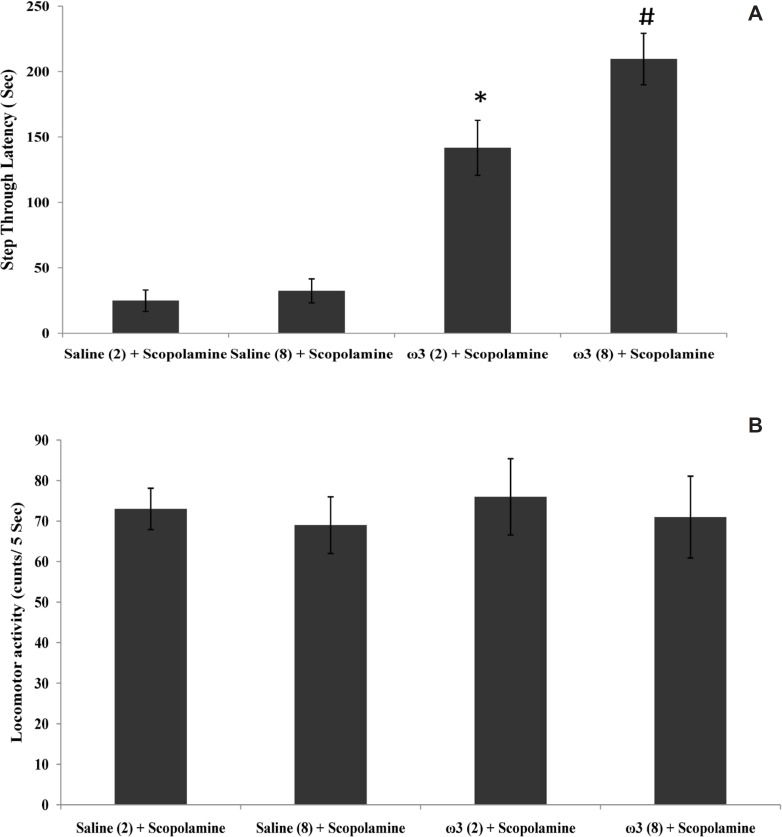
Data analysis
The results were statistically evaluated by Independent-samples t-test. All data were expressed as mean ± Standard Error of Mean (SEM). In all comparisons, statistical significance level was determind as p < 0.05.
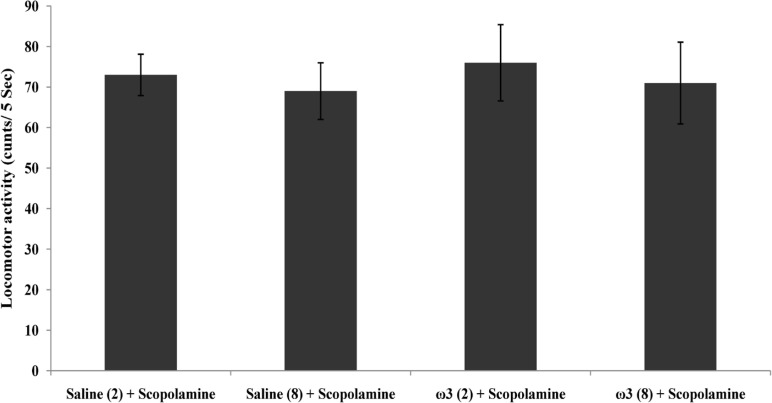
Results and Discussion
The effects of short-term or long-term pre-exposure to omega-3 fatty acids on scopolamine-induced amnesia
As shown in Figure 1A, the amnesia induced by pre-training scopolamine administration was significantly decreased in rats which had previously received 60 mg/Kg of omega-3 once daily for 2 weeks or 8 weeks (p < 0.01 and p < 0.001, respectively vs. scopolamine-treated control groups). Locomotor activity (p = 0.94) in this experiment was not significantly different in rats which received omega-3 fatty acids and saline-treated groups for 2 or 8 weeks (Figure 1B).
MDA contents and CAT and SOD activity
Tissue level of MDA in group of rats which were treated for 2 weeks with 60 mg/Kg omega-3 fatty acids remained unchanged compared to the control group (p > 0.05), however, long-term pretreatment with omega-3 fatty acids at dose of 60 mg/Kg for 8 weeks decreased the tissue MDA contents after the scopolamine injection (p < 0.01 vs. saline (8 weeks) + scopolamine group; Figure 2).
SOD activity in hippocampal tissue does not increased through short-term pretreatment with omega-3 fatty acids (60 mg/Kg), while after 8 weeks of treatment, SOD activity was decreased significantly compared with the control group (p < 0.05; Figure 3).
CAT activity remained unchanged by short-term pretreatment of omega-3 fatty acids (60 mg/Kg) plus scopolamine (p > 0.05; Figure 4). In long-term omega-3 fatty acids-treated group (60 mg/Kg) CAT activity was significantly increased (p < 0.01 vs. saline (8 weeks) + scopolamine; Figure 4).
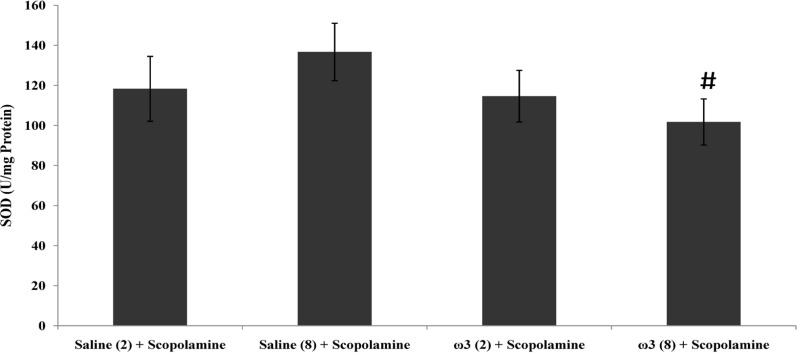
Bax and Bcl-2 expression
Beta-actin used as a reference protein to evaluate the expression of Bcl-2 or Bax compared to it and presented the value as the ratio of these apoptotic proteins to this protein. The expression of pro-apoptotic protein Bax (21KD) was decreased by the short-term treatment of omega-3 fatty acids (60 mg/Kg), however, it was not statistically significant (p = 0.078; Table 2). Long-term pretreatment with omega-3 fatty acids (60 mg/Kg), significantly decreased the ratio of Bax to beta-actin protein (p < 0.05). The expression of Bcl-2 was not changed significantly using the short or long-term treatment of mega-3 fatty acids at the dose of 60 mg/Kg at present study (Table 2).
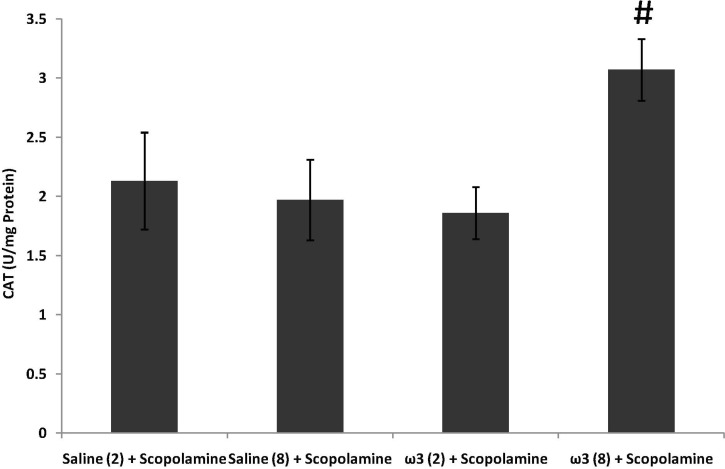
Expression of Bax or Bcl-2 in hippocampus of different groups
N | Weeks of treatment | Bax / β-actin ratio | Bcl-2 / β-actin ratio | |
---|---|---|---|---|
Saline (2) + Scopolamine | 12 | 2 | 90 ± 11 | 47 ± 7 |
Saline (8) + Scopolamine | 12 | 8 | 86 ± 14 | 51 ± 8 |
ω3 (2) + Scopolamine | 12 | 2 | 79 ± 15 | 42 ± 8 |
ω3 (8) + Scopolamine | 12 | 8 | 61 ± 7 # | 69 ± 9 |
Dietary omega-3 fatty acids can be hopeful to improve learning and memory (4-6). To our knowledge, for the first time, the present study suggested the beneficial effects of long-term exposure to omega-3 fatty acids on the scopolamine-induced amnesia and its impact on the other molecular events including oxidative stress and apoptosis while the effect of short-term treatment was significant just on the behavioral test.
Reduced latency time for entering the dark part was concomitant with the increased tissue MDA contents and increased SOD activity in hippocampal tissue of rats which were treated with saline and scopolamine. The similar results had been concluded in a very recent study (16) which conducted on the mice brain and confirmed that scopolamine (2 mg/Kg, IP) impairs learning in passive avoidance and the Morris water maze tests, along with the increased MDA contents (lipid peroxidation) and SOD activity but decreased CAT activity. Obvious evidences exist between the oxidative damage of hippocampus and learning impairments (17, 18) by affected gene expression (19). Decreased membrane fluidity is the main consequence of exposure to the reactive oxygen species that impairs cell signaling. In addition, the previous research demonstrated that the overexpression of SOD can impair the LTP formation in CA1 neuronal synapses and consequently affect the memory’s function which is reversible through the treatment of hippocampal slices with catalase (20) or SOD inhibitor (21). The higher levels of CAT activity in the group treated with omega-3 acids for long time was concomitant with the improved memory that is in accordance with the protective effects of CAT on defected memory function.
The previous studies have shown that the overexpression of Bcl-2 not only protects the neural cells against oxidative stress, but also enhances the survival of newborn neurons in primary hippocampal cultures and in adult rat brain (22). The impact of omega-3 fatty acids on the expression of Bcl-2 and Bax proteins has been evaluated in brain (23, 24) which confirms the decreasing of Bax to Bcl-2 ratio in hippocampal tissue after the long-term of pretreatment with omega-3 fatty acids in present study. DHA enhances the expression of anti-apoptotic members of the Bcl-2 gene family and downregulates proapoptotic proteins Bax and Bik (2). In present study, significant changes at molecular level were obtained by long-term treatment with omega-3 fatty acids that emphasized the optimal effect of these agents by their long-term application. It seems that at initial phases, omega-3 fatty acids act by using current cellular resources, while after a long time, it can induce the structural changes which help the synaptic strengthening and learning or memory performance.
In conclusion, we can attribute the protective effects of short-term omega-3 fatty acids treatment on learning and memory to functional factors while after a long time period, the protein translation and the inhibition of neuronal apoptosis and oxidative damage are involved in the anti-amnesic effects of omega-3 fatty acids.
Acknowledgements
References
-
1.
Yuen AW, Sander JW, Fluegel D, Patsalos PN, Bell GS, Johnson T, Koepp MJ. Omega-3 fatty acid supplementation in patients with chronic epilepsy: a randomized trial. Epilepsy Behav. 2005;7:253-258. [PubMed ID: 16006194].
-
2.
Lukiw WJ, Cui JG, Marcheselli VL, Bodker M, Botkjaer A, Gotlinger K, Serhan CN, Bazan NG. A role for docosahexaenoic acid-derived neuroprotectin D1 in neural cell survival and Alzheimer disease. J. Clin. Invest. 2005;115:2774-2783. [PubMed ID: 16151530].
-
3.
Chung WL, Chen JJ, Su HM. Fish oil supplementation of control and (n-3) fatty acid-deficient male rats enhances reference and working memory performance and increases brain regional docosahexaenoic acid levels. J. Nutr. 2008;138:1165-1171. [PubMed ID: 18492851].
-
4.
Fujita S, Ikegaya Y, Nishikawa M, Nishiyama N, Matsuki N. Docosahexaenoic acid improves long-term potentiation attenuated by phospholipase A(2) inhibitor in rat hippocampal slices. Br. J. Pharmacol. 2001;132:1417-1422. [PubMed ID: 11264234].
-
5.
Kawashima A, Harada T, Kami H, Yano T, Imada K, Mizuguchi K. Effects of eicosapentaenoic acid on synaptic plasticity, fatty acid profile and phosphoinositide 3-kinase signaling in rat hippocampus and differentiated PC12 cells. J. Nutr. Biochem. 2010;21:268-277. [PubMed ID: 19369057].
-
6.
Cao D, Xue R, Xu J, Liu Z. Effects of docosahexaenoic acid on the survival and neurite outgrowth of rat cortical neurons in primary cultures. J. Nutr. Biochem. 2005;169:538-546. [PubMed ID: 16115542].
-
7.
Akbar M, Calderon F, Wen Z, Kim HY. Docosahexaenoic acid: a positive modulator of signaling in neuronal survival. Proc. Natl. Acad. Sci. USA. 2005;102:10858-10863. [PubMed ID: 16040805].
-
8.
Moreira JD, Knorr L, Thomazi AP, Simao F, Battu C, Oses JP, Gottfried C, Wofchuk S, Salbego C, Souza DO, Perry ML, Vinade L. Dietary omega-3 fatty acids attenuate cellular damage after a hippocampal ischemic insult in adult rats. J. Nutr. Biochem. 2010;21:351-6. [PubMed ID: 19410444].
-
9.
Plamondon H, Roberge MC. Dietary PUFA supplements reduce memory deficits but not CA1 ischemic injury in rats. Physiol. Behav. 2008;95:492-500. [PubMed ID: 18700150].
-
10.
Stewart ZA, Pietenpol JA. p53 Signaling and cell cycle check-points. Chem. Res. Toxicol. 2001;14:243-263. [PubMed ID: 11258974].
-
11.
Lawrence MS, Ho DY, Sun GH, Steinberg GK, Sapolsky RM. Overexpression of Bcl-2 with herpes simplex virus vectors protect CNS neurons against neurological insults in-vitro and in-vivo. J. Neurosci. 1996;16:486-496. [PubMed ID: 8551333].
-
12.
Sinha RA, Khare P, Rai A, Maurya SK, Pathak A, Mohan V, Nagar GK, Mudiam MK, Godbole MM, Bandyopadhyay S. Anti-apoptotic role of omega-3-fatty acids in developing brain: perinatal hypothyroid rat cerebellum as apoptotic model. Int. J. Dev. Neurosci. 2009;27:377-83. [PubMed ID: 19460632].
-
13.
Fernandes JS, Mori MA, Ekuni R, Oliveira RM, Milani H. Long-term treatment with fish oil prevents memory impairments but not hippocampal damage in rats subjected to transient, global cerebral ischemia. Nutr. Res. 2008;28:798-808. [PubMed ID: 19083490].
-
14.
Zarrindast MR, Nouri M, Ahmadi S. Cannabinoid CB1 receptors of the dorsal hippocampus are important for induction of conditioned place preference (CPP) but do not change morphine CPP. Brain. Res. 2007;1163:130-7. [PubMed ID: 17631872].
-
15.
Uchiyama M, Mihara M. Determination of malonaldehyde precursor in tissues by thiobarbituric acid. Anal. Biochem. 1978;86:271-278. [PubMed ID: 655387].
-
16.
Lee MR, Yun BS, Park SY, Ly SY, Kim SN, Han BH, Sung CK. Anti-amnesic effect of Chong-Myung-Tang on scopolamine-induced memory impairments in mice. J. Ethnopharmacol. 2010;132:70-4. [PubMed ID: 20673844].
-
17.
Lien TV, Bich HT, Phuong LT, Han TH. The effect of HHKV and TCTN1 extracts on malonyl dialdehyde (MDA) level of the brain. Iranian J. Pharm. Res. 2004;3:75-75.
-
18.
Fukui K, Onodera K, Shinkai T, Suzuki S, Urano S. Impairment of learning and memory in rats caused by oxidative stress and aging, and changes in antioxidative defense systems. Ann. NY. Acad. Sci. 2001;928:168-175. [PubMed ID: 11795507].
-
19.
Nicolle MM, Gonzalez J, Sugaya K, Baskerville KA, Bryan D, Lund K, Gallagher M, McKinney M. Signatures of hippocampal oxidative stress in aged spatial learning-impaired rodents. Neuroscience. 2001;107:415-431. [PubMed ID: 11718997].
-
20.
Gahtan E, Auerbach JM, Groner Y, Segal M. Reversible impairment of long-term potentiation in transgenic Cu/Zn-SOD mice. Eur. J. Neurosci. 1998;10:538-544. [PubMed ID: 9749716].
-
21.
Thiels E, Urban NN, Gonzalez-Burgos GR, Kanterewicz BI, Barrionuevo G, Chu CT, Oury TD, Klann E. Impairment of long-term potentiation and associative memory in mice that overexpress extracellular superoxide dismutase. J. Neurosci. 2000;20:7631-7639. [PubMed ID: 11027223].
-
22.
Sasaki T, Kitagawa K, Yagita Y, Sugiura S, Omura-Matsuoka E, Tanaka S, Matsushita K, Okano H, Tsujimoto Y, Hori M. Bcl2 enhances survival of newborn neurons in the normal and ischemic hippocampus. J. Neurosci. Res. 2006;84:1187-96. [PubMed ID: 16941650].
-
23.
Pan HC, Kao TK, Ou YC, Yang DY, Yen YJ, Wang CC, Chuang YH, Liao SL, Raung SL, Wu CW, Chiang AN, Chen CJ. Protective effect of docosahexaenoic acid against brain injury in ischemic rats. J. Nutr. Biochem. 2009;20:715-25. [PubMed ID: 18805685].
-
24.
Sinha RA, Khare P, Rai A, Maurya SK, Pathak A, Mohan V, Nagar GK, Mudiam MK, Godbole MM, Bandyopadhyay S. Anti-apoptotic role of omega-3-fatty acids in developing brain: perinatal hypothyroid rat cerebellum as apoptotic model. Int. J. Dev. Neurosci. 2009;27:377-83. [PubMed ID: 19460632].