Abstract
Keywords
Epigallocatechin-3-gallate hyperalgesia oxidative stress Diabetic rat
Introduction
Diabetes mellitus is one of the serious problems worldwide and the number of diabetic people is estimated to be increased markedly by the year 2030 (1). Uncontrolled chronic hyperglycemia in diabetes leads to severe complications including neuropathy, retinopathy, and autonomic dysfunctions. Diabetic neuropathy as observed in some deranged conditions of nociception (i.e. hyperalgesia) is the most common complication with an incidence of more than 50% (2). Diabetes-induced deficits in motor and sensory nerve conduction velocities and other manifestations of peripheral diabetic neuropathy have been well correlated with chronic hyperglycemia. Hyperglycemia could also lead to an increased oxidative stress (enhanced free radical formation and/or a defect in antioxidant defenses), advanced glycation end product formation, nerve hypoxia/ischemia, and impaired nerve growth factor support (3- 5). Several studies suggest that an oxidative stress may be one of the major pathways in the development of diabetic neuropathy and the antioxidant therapy can prevent or reverse the hyperglycemia-induced nerve dysfunctions (6, 7). Recent interests are focusing on the use of non-vitamin antioxidants such as flavonoids in reducing the devastating complications of diabetes in experimental animals and patients (8). Plant-based pharmaceuticals including flavonoids have been employed in the management of various mankind diseases (8). They are as an essential part of human diet and are present in plant extracts that have been used for centuries in oriental medicine. Antioxidant properties, reactive oxygen species (ROS) scavenging, and cell function modulation of flavonoids could account for the large part of their pharmacological activity (8-10). Since diabetes mellitus is considered as a free radical mediated disease, there has been renewed interest in the use of flavonoids in diabetes research. Green tea polyphenols are natural plant flavonoids that comprise many types of catechins. Among these, epigallocatechin-3-gallate (EGCG), comprising about one-third of green tea dry mass (11), is a polyphenolic bioflavonoid derived from a variety of plants, especially green tea that is primarily responsible for its beneficial effects. It has been suggested that EGCG could attenuate lipid peroxidation (12), being capable of reducing the risk of type 1 diabetes (13), and exert the hypoglycemic and hypolipidemic effects (14). EGCG has also been proved to exert europrotective/neurorescue activities against oxidative damage and neurodegeneration (15-17). An increasing body of evidence has demonstrated that EGCG possesses neuroprotective effects against a variety of toxic insults and inflammatory neuronal injuries (18-21). Previous studies also showed that green tea extract has neuroprotective effect on the ischemia/reperfusion-induced brain injury through the inhibition of inflammatory stress-induced neuronal cell death (22, 23). Therefore, we designed this study to investigate, for the first time, the effect of chronic EGCG treatment, a free radical scavenger on hyperalgesia in streptozotocin-diabetic (STZ-diabetic) neuropathic rat, using standard formalin and hot tail immersion tests and also to evaluate the role of oxidative stress.
Experimental
Animals
Male albino Wistar rats (Pasteur’s institute, Tehran, Iran) weighing 225-285 g (10-12 weeks old) were housed in an air-conditioned colony room on a light/dark cycle (21-23°C and a humidity of 30-40%) and supplied with standard pelleted diet and tap water ad libitum. Procedures involving animals and their care were conducted in conformity with the NIH guidelines for the care and use of laboratory animals.
Experimental procedure
The rats (n = 56) were randomly allocated and similarly grouped into seven groups: normal saline-treated control, EGCG-treated control at a dose of 40 mg/Kg, saline-treated diabetic, EGCG-treated diabetic groups at doses of 20 and 40 mg/Kg and sodium salicylate (SS)-treated control and diabetic. The rats were rendered diabetic by a single intraperitoneal injection of 60 mg Kg-1 streptozotocin (STZ) (Pharmacia and Upjohn, USA) freshly dissolved in cold normal saline. Control animals received an injection of an equivalent volume of normal saline. One week after the STZ injection, overnight fasting blood samples were collected and serum glucose concentrations were measured using glucose oxidation method (Zistshimi, Tehran). Only those animals with a fasting serum glucose level higher than 250 mg dL-1 were selected as diabetic for the following experiments. The day on which hyperglycemia had been confirmed was designated as day 0. Diabetes was symptomatically verified by the presence of hyperglycemia, polyphagia, polydipsia, polyuria and weight loss in the following weeks. EGCG (Sigma, USA) was administered p.o. at doses of 20 and 40 mg/Kg/day one week after STZ injection for a period of 7 weeks. Dose of EGCG was chosen according to our pilot study and previous studies (24, 25). EGCG was dissolved in normal saline and freshly administered. SS (200 mg/Kg, IP) was administered 1 h before conducting the formalin test as a positive control. Serum glucose level and body weight were monitored at the start and regularly every week until the end of the study. Changes in food consumption and water intake were regularly observed (quantitatively was not measured) during the experimental period. Pain-related behaviors including formalin, hot tail immersion and paw pressure tests were performed at the end of the study as described below.
Formalin test
The previously described method was applied (26). Briefly, each animal was acclimatized to the observation box before any testing began. Then, it was given a subcutaneous injection of 50 μL of 2.5% formalin into the plantar surface of one hind paw. It was then immediately placed in a Plexiglas box. Observations were continued for the next 60 min. A nociceptive score was determined for 5-min blocks by measuring the amount of time spent in each of the four behavioral categories: 0, the position and posture of the injected hind paw is indistinguishable from the contralateral paw; 1, the injected paw has little or no weight placed on it; 2, the injected paw is elevated and is not in contact with any surface; 3, the injected paw is licked, bitten, or shaken. Then, a weighted nociceptive score, ranging from 0 to 3 was calculated by multiplying the time spent in each category by the category weight, summing these products and dividing by the total time for each 5 min block of time. The first 10 min post-injection was considered as the early (first) phase and the time interval 15-60 as the late (sec) phase.
Hot tail immersion test
Diabetic thermal hyperalgesia was assessed using tail immersion test (27). After the adaptation, rat tail was immersed in warm water (49°C) and the tail flick response latency (withdrawal response of tail) was observed as the end-point response. Each experiment was repeated 4 times for each animal with an interval of 2 min and its average was reported. Meanwhile, a cut-off time of 30 sec was also considered.
Paw pressure test
To test the effect of EGCG on mechanical hyperalgesia, the vocalization thresholds of paw pressure in diabetic rats were measured as described by Randall and Selitto (28) using an analgesia-meter. Increasing pressure (32 g sec-1) was applied through a plastic tip onto the dorsal surface between the 3rd and 4th metatarsus of the left hind paw until the rat squeaked. Vocalization thresholds were expressed in grams and the cutoff was 500 g. Threshold measurements were repeated twice and the average was taken.
Assay of serum MDA and nitrite concentration and erythrocyte SOD activity
The MDA concentration (thiobarbituric acid reactive substances, TBARS) in the serum was measured as dsecribed before (29). Briefly, 1.0 mL of 20% trichloroacetic acid and 1.0 mL of 1% TBARS reagent were added to 100 μL of serum, then mixed and incubated at boiling water for 80 min. After cooling on ice, samples were centrifuged at 1000×g for 20 min and the absorbance of the supernatant was read at 532 nm. TBARS results were expressed as MDA equivalents using tetraethoxypropane as standard.
Serum nitrite content was assayed by the Griess method (30). Because NO is a compound with a short half-life and is rapidly converted to the stable end products nitrate (NO3) and nitrite (NO2), the principle of the assay is the conversion of nitrate into nitrite by cadmium and followed by color development with Griess reagent (sulfanilamide and n-naphthyl ethylenediamine) in acidic medium. The total nitrite was measured by Griess reaction. The absorbance was determined at 540 nm.
For SOD activity, after centrifuging blood samples (3000 rpm, 4°C, 10 min), RBC portion was seperated and made into 5% homogenate using a homogenizer in cold saline solution. Then, after recentrfuging, the supernatant was used for assay. In this respect, a competitive inhibition assay was performed using xanthine/xanthine oxidase reaction-generated superoxide radicals to reduce nitro blue tetrazolium (NBT) quantitatively to blue formazan. Conversion of superoxide radicals to hydrogen peroxide by superoxide dismutase inhibited dye formation and served as a measure of superoxide dismutase activity. Briefly, 0.5 mL of supernatant was incubated with xanthine (50 μmol/L) and xanthine oxidase (2.5 μmol/L) in 50 mmol/L of potassium phosphate buffer (pH = 7.8, 37ºC) for 40 min and NBT was added. Blue formazan was then monitored spectrophotometrically at 550 nm. The amount of protein that inhibited NBT reduction to 50% maximum was defined as 1 nitrite unit (NU) of SOD activity (29).
Chemicals
EGCG was purchased from Sigma Chemical (St Louis, MO, USA) and dissolved in normal saline and freshly administered. Sodium salicylate was obtained from Darupakhsh (Tehran, Iran) and streptozotocin from Pharmacia and Upjohn (New Jersey, USA). All other chemicals were purchased from Merck (Darmstadt, Germany) and Temad (Tehran, Iran).
Statistical analysis
All results are expressed as mean ± SEM. Distribution of our data follows a normal pattern. Significance of difference between two groups was evaluated using unpaired and paired Student’s t-test. For multiple comparisons, one-way analysis of variance (ANOVA) was used. When ANOVA showed significant difference, Tukey’s post-hoc test was applied. Statistical significance was regarded as p < 0.05.
Results
General considerations
One week after the STZ injection, four rats (one rat from saline-treated diabetic and three rats from EGCG-treated diabetic groups) were excluded from the study due to their serum glucose level lower than 250 mg/dL and/or being dead.
The weight of the vehicle-treated diabetic rats at the 8th week was found to be significantly decreased as compared to control rats (p < 0.01) and EGCG treatment at a dose of 40 mg/Kg caused a less significant decrease in the weight of the diabetic rats as compared with untreated diabetic rats (p < 0.05; Figure 1). In addition, diabetic rats had also an elevated serum glucose level over those of control rats (p < 0.0001) and treatment of diabetic rats with EGCG at both doses of 20 and 40 mg/Kg for 7 weeks caused a significant decrease in the serum glucose (p < 0.01) relative to saline-treated diabetics. Meanwhile, EGCG treatment of control rats did not produce any significant change regarding serum glucose level (Figure 2).
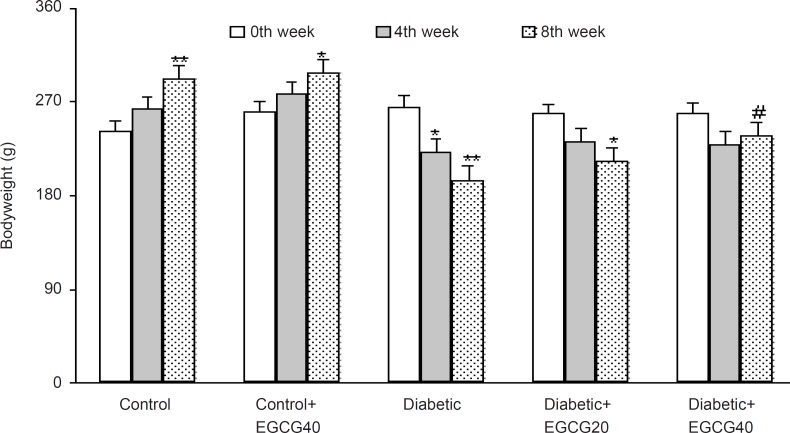
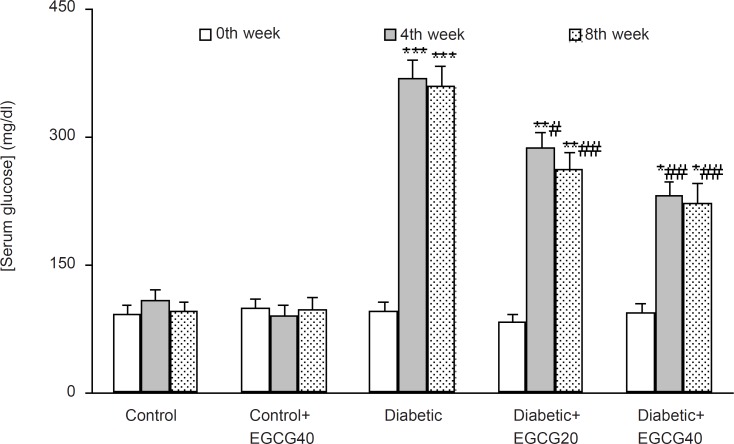
Formalin test
Hind limb formalin injection produced a marked biphasic response in the rats of all groups. Hyperalgesia was significantly (p < 0.05) greater in untreated diabetics than in control rats in both phases of the test. Pretreatment of control and diabetic rats with sodium salicylate (200 mg/Kg, IP) 1 h before the test caused a significant reduction (p < 0.01) in nociceptive score only in the second phase of the formalin test. In addition, the treatment of diabetic rats with EGCG (40 mg/Kg) caused lower nociceptive scores in both phases of the formalin test as compared to vehicle-treated diabetic rats (p < 0.05). No such response was observed for EGCG (40 mg/Kg)-treated control and EGCG (20 mg/Kg)-treated diabetic rats (Figure 3).
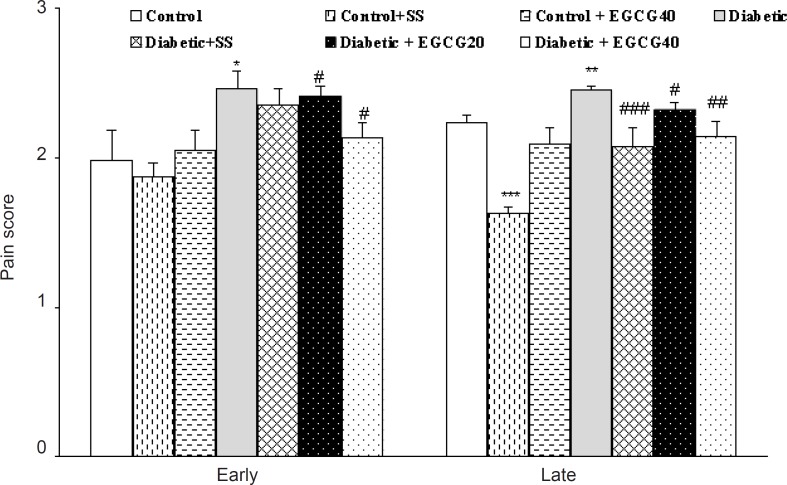
Thermal and mechanical hyperalgesia
A significant decrease in tail flick latency was observed after two months of diabetes in hot tail immersion test (p < 0.01). This deficit in tail flick response latency was significantly (p < 0.05) reversed on treatment with EGCG (40 mg/Kg). To examine the effect of EGCG on mechanical hyperalgesia, diabetic rats were subjected to the Randall-Selitto test. The vocalization threshold in diabetic rats was lower than that in age- matched normal rats (129.1 ± 20.33 g vs. 214 ± 16.2 g, respectively; p < 0.005). EGCG (40 mg/Kg) increased the vocalization threshold in diabetic rats (129.1 ± 20.33 g vs. 188.6 ± 19.3; p < 0.05) (Figures 4).
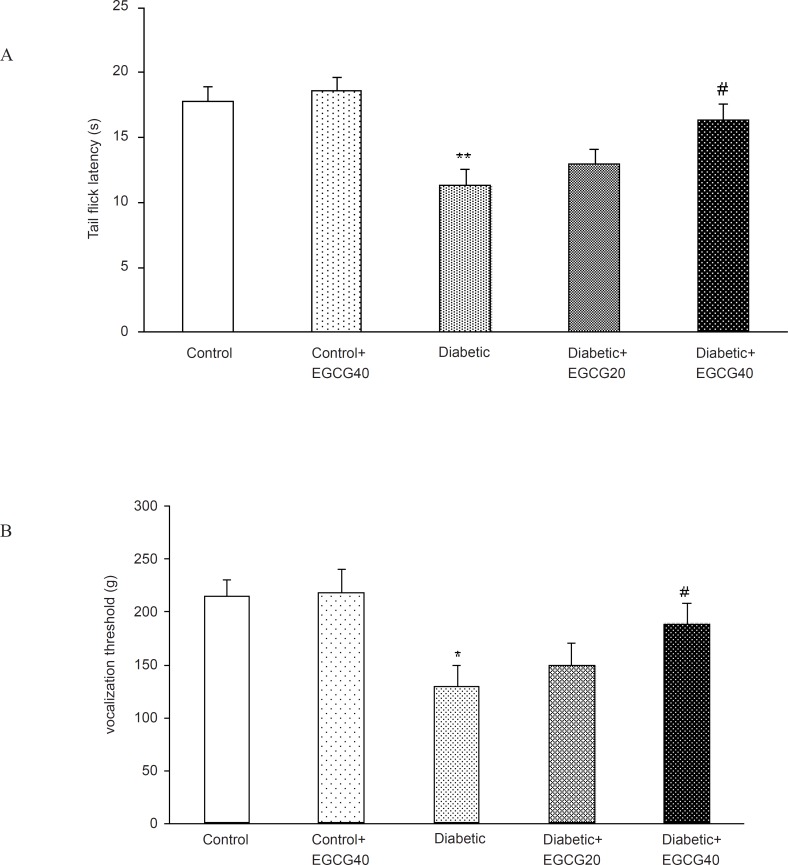
Markers of oxidative stress
Regarding oxidative stress markers, STZ-induced diabetes resulted in an elevation of serum MDA (p < 0.001), nitrite (p < 0.01) content and decreased erythrocyte SOD activity (p = 0.03). Treatment of diabetic group with EGCG (40 mg/ Kg) for 7 weeks significantly lowered MDA (p = 0.02), nitrite (p < 0.05) content and significantly attenuated the reduced activity of SOD (p < 0.05). Meanwhile, there was no significant change in EGCG-treated control group relative to control animals with respect to these parameters (Figure 5-7).
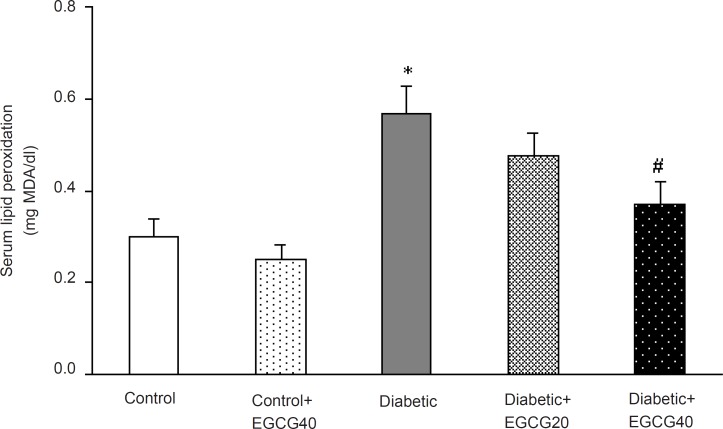
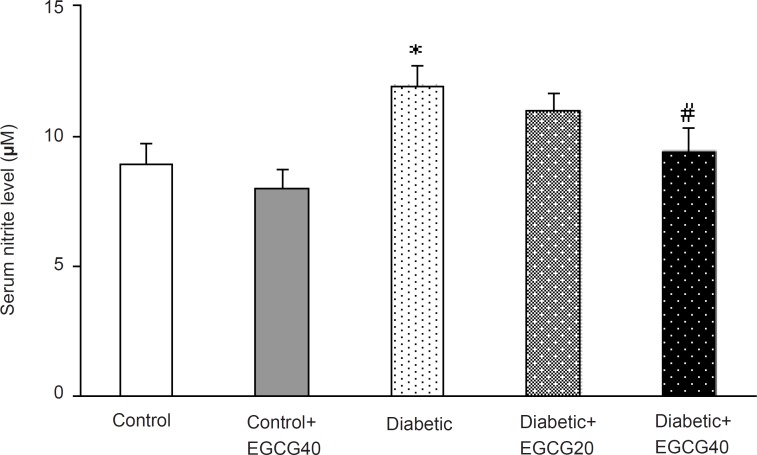
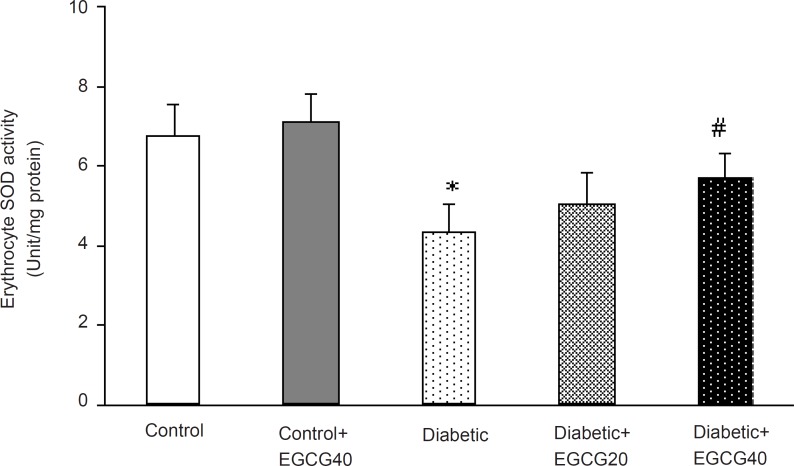
Discussion
The main findings of this study were three-fold. First, long-term diabetes was accompanied with disturbances in pain-related behaviors, as was evident by a reduction in tail flick latency and vocalization threshold and an intensified nociceptive response in both phases of the formalin test. It is a well-established fact that diabetic rats display exaggerated hyperalgesic behavior in response to noxious stimuli (31) and for this reason STZ-diabetic rats have been increasingly used as a model of painful diabetic neuropathy to assess the efficacy of potential analgesic agents (32). Although the evaluation of mechanisms causing these symptoms is complicated because of the overlap between the systemic effects of hyperglycemia and its toxic effects within the peripheral nervous system, direct functional toxicity of hyperglycemia in the peripheral nervous system (33), an increased activity of primary afferent fibers leading to an increased excitatory tone within the spinal cord, increased release of glutamate and activation of the NMDA receptor, reduced activity of both opioidergic and GABAergic inhibitory systems (34), decreased activity of nNOS-cGMP system in neurons of dorsal root ganglion (35), altered sensitivity of the dopaminergic receptors and altered responsiveness of the dopaminergic system, possibly through the enhancement and/or deactivation of the endogenous Met-enkephalinergic system (36), and alterations in L-type Ca2+ channels (37) could be involved in the modulation of nociception in diabetic rats.
Second, it was demonstrated that oral administration of EGCG at a dose of 40 mg/Kg for a period of 7 weeks could attenuate the reduction in tail flick latency and vocalization threshold and produce a significant analgesic effect in both phases of the formalin test in diabetic rats. On the other hand, sodium salicylate significantly reduced the nociceptive score only in the second phase of the formalin test in control and diabetic rats. It has been known that central acting drugs like narcotics inhibit both phases of the formalin test equally (38), while peripheral acting drugs like aspirin only inhibit the late phase (39). Therefore, the effect of EGCG in this study could be mediated possibly through a central and/or a peripheral mechanism. One of the possible mechanisms that could partially explain the beneficial analgesic property of EGCG may be attributed to its hypoglycemic effect, as observed in this study. In this respect, hypoglycemic effect of EGCG at a dose of 25 mg/Kg for two months has been reported before (14). EGCG has been suggested to inhibit the hepatic gluconeogenesis through a ROS-dependent pathway. EGCG also mimics the cellular effects of insulin such as reducing gene expression of rate-limiting gluconeogenic enzymes (40, 41). Furthermore, EGCG like insulin may increase tyrosine phosphorylation of the insulin receptor and insulin receptor substrate-1 and it reduces phosphoenolpyruvate carboxykinase gene expression in a phosphoinositide 3-kinase-dependent manner. EGCG also mimics insulin by increasing phosphoinositide 3-kinase, mitogen-activated protein kinase, and p70 (s6k) activity (42).
Third, increased free radical mediated-toxicity has also been well documented in clinical diabetes (43) and STZ-diabetic rats (44). Furthermore, oxidative stress is one of the main causes of development of peripheral nerve damage in diabetic neuropathy (45). The elevated level of toxic oxidants in diabetic state may be due to processes such as glucose oxidation and lipid peroxidation. STZ-diabetes is characterized by several derangements in endogenous antioxidant enzymes (43) and the induction of antioxidant enzymes is a critical approach for protecting cells against a variety of endogenous and exogenous toxic compounds such as ROS (44). Numerous studies have shown that green tea flavonoids, have antioxidant and iron chelating activities and thus, can prevent and/or reduce the deleterious effects of oxygen-derived free radicals associated with various chronic diseases (46, 47). Of these, EGCG is the most effective antioxidant (48, 49). Although the mechanisms of EGCG’s antioxidant activity remain unclear, pharmacological studies have identified several antioxidant properties such as: (1) blockade of nNOS and iNOS induction (49-51) and (2) scavenging of free radicals or attenuation of lipid peroxidation (48, 49, 53, 54). Thus, EGCG is expected to be neuroprotective. In our study, chronic EGCG treatment at a dose of 40 mg/Kg for 7 weeks partially reduced lipid peroxidation and nitrite content and improved SOD activity in diabetic rats. Therefore, part of beneficial effect of EGCG in this research could be attributed to the attenuation of oxidative stress in diabetic rats.
To conclude, chronic administration of EGCG could attenuate the hyperalgesic state of diabetic rats and this may be of potential benefit in painful diabetic conditions.
Acknowledgements
References
-
1.
Wild S, Roglic G, Green A, Sicree R, King H. Global prevalence of diabetes: estimates for the year 2000 and projections for 2030. Diabetes Care. 2004;27:1047-1053. [PubMed ID: 15111519].
-
2.
Sima AA, Sugimoto K. Experimental diabetic neuropathy: an update. Diabetologia. 1999;42:773-788. [PubMed ID: 10440118].
-
3.
Van Dam PS. Oxidative stress and diabetic neuropathy: pathophysiological mechanisms and treatment perspectives. Diabetes Metab. Res. Rev. 2002;18:176-184. [PubMed ID: 12112935].
-
4.
Obrosova IG. Update on the pathogenesis of diabetic neuropathy. Curr. Diab. Rep. 2003;3:439-445. [PubMed ID: 14611738].
-
5.
Vincent AM, Russell JW, Low P, Feldman EL. Oxidative stress in the pathogenesis of diabetic neuropathy. Endocr. Rev. 2004;25:612-628. [PubMed ID: 15294884].
-
6.
Nickander KK, McPhee BR, Low PA, Tritschler H. Alpha-lipoic acid: antioxidant potency against lipid peroxidation of neural tissues in-vitro and implications for diabetic neuropathy. Free Radic. Biol. Med. 1996;21:631-639. [PubMed ID: 8891666].
-
7.
Cameron NE, Cotter MA. Effects of antioxidants on nerve and vascular dysfunction in experimental diabetes. Diabetes Res. Clin. Pract. 1999;45:137-146. [PubMed ID: 10588366].
-
8.
Laight DW, Carrier MJ, Anggard EE. Antioxidants, diabetes and endothelial dysfunction. Cardiovasc. Res. 2000;47:457-464. [PubMed ID: 10963719].
-
9.
Machha A, Mustafa MR. Chronic treatment with flavonoids prevents endothelial dysfunction in spontaneously hypertensive rat aorta. J. Cardiovasc. Pharmacol. 2005;46:36-40. [PubMed ID: 15965352].
-
10.
Chu JX, Wang AL, Han SHY. The Effects of total flavonoids from buckwheat flowers and leaves on renal damage and PTP1B expression in type 2 diabetic rats. Iranian J. Pharm. Res. 2011;10:511-517.
-
11.
Yang CS, Chen L, Lee MJ, Balentine D, Kuo MC, Schantz SP. Blood and urine levels of tea catechins after ingestion of different amounts of green tea by human volunteers. Cancer Epidemiol. Biomarkers Prev. 1998;7:351-4. [PubMed ID: 9568793].
-
12.
Guo Q, Zhao B, Li M, Shen S, Xin W. Studies on protective mechanisms of four components of green tea polyphenols against lipid peroxidation in synaptosomes. Biochim. Biophys. Acta. 1996;1304:210-22. [PubMed ID: 8982267].
-
13.
Fu Z, Zhen W, Yuskavage J, Liu D. Epigallocatechin gallate delays the onset of type 1 diabetes in spontaneous non-obese diabetic mice. Br. J. Nutr. 2011;105:1218-25. [PubMed ID: 21144096].
-
14.
Roghani M, Baluchnejadmojarad T. Hypoglycemic and hypolipidemic effect and antioxidant activity of chronic epigallocatechin-gallate in streptozotocin-diabetic rats. Pathophysiol. 2010;17:55-9.
-
15.
Kang KS, Wen Y, Yamabe N, Fukui M, Bishop SC, Zhu BT. Dual beneficial effects of (-)-epigallocatechin-3-gallate on levodopa methylation and hippocampal neurodegeneration: in-vitro and in-vivo studies. PLoSOne. 2010;5:e11951.
-
16.
Mandel SA, Amit T, Weinreb O, Reznichenko L, Youdim MB. Simultaneous manipulation of multiple brain targets by green tea catechins: a potential neuroprotective strategy for Alzheimer and Parkinson diseases. CNS Neurosci. Ther. 2008;14:352-65. [PubMed ID: 19040558].
-
17.
Weinreb O, Amit T, Mandel S, Youdim MB. Neuroprotective molecular mechanisms of (-)-epigallocatechin-3-gallate: a reflective outcome of its antioxidant, iron chelating and neuritogenic properties. Genes Nutr. 2009;4:283-296. [PubMed ID: 19756809].
-
18.
Mandel S, Einreb O, Amit T, Youdim MB. Cell signaling pathways in the neuroprotective actions of the green tea polyphenol (−)-epigallocatechin-3-gallate: implications for neurodegenerative diseases. J. Neurochem. 2004;88:1555-15569. [PubMed ID: 15009657].
-
19.
Cho HS, Kim S, Lee SY, Park JA, Kim SJ, Chun HS. Protective effect of the green tea component, L-theanine on environmental toxins-induced neuronal cell death. Neurotoxicol. 2008;29:656-662.
-
20.
Zhang B, Rusciano D, Osborne NN. Orally administered epigallocatechin gallate attenuates retinal neuronal death in-vivo and light-induced apoptosis in-vitro. Brain Res. 2008;1198:141-152. [PubMed ID: 18255049].
-
21.
Antonio AM, Druse MJ. Antioxidants prevent ethanol-associated apoptosis in fetal rhombencephalic neurons. Brain Res. 2008;1204:16-23. [PubMed ID: 18329634].
-
22.
Hong JT, Ryu SR, Kim HJ, Lee JK, Lee SH, Kim DB, Yun YP, Ryu JH, Lee BM, Kim PY. Neuroprotective effect of green tea extract in experimental ischemiareperfusion brain injury. Brain Res. Bull. 2000;53:743-749. [PubMed ID: 11179838].
-
23.
Hong JT, Ryu SR, Kim HJ, Lee JK, Lee SH, Yun YP, Lee BM, Kim PY. Protective effect of green tea extract on ischemia/reperfusion-induced brain injury in Mongolian gerbils. Brain Res. 2001;88:11-18. [PubMed ID: 11146047].
-
24.
Kumar P, Kumar A. Effect of lycopene and epigallocatechin-3-gallate against 3-nitropropionic acid induced cognitive dysfunction and glutathione depletion in rat: a novel nitric oxide mechanism. Food Chem. Toxicol. 2009;47:2522-30. [PubMed ID: 19616597].
-
25.
Rezai-Zadeh K, Shytle D, Sun N, Mori T, Hou H, Jeanniton D. Green tea epigallocatechin-3-gallate (EGCG) modulates amyloid precursor protein cleavage and reduces cerebral amyloidosis in Alzheimer transgenic mice. J. Neurosci. 2005;25:8807-14. [PubMed ID: 16177050].
-
26.
Dubuisson D, Dennis SG. The formalin test: a quantitative study of the analgesic effects of morphine, meperidine and brain stem stimulation in rats and cats. Pain. 1977;4:161-174. [PubMed ID: 564014].
-
27.
Courteix C, Eschalier A, Lavarenne J. Streptozotocin-induced diabetic rats: behavioral evidence for a model of chronic pain. Pain. 1993;53:81-88. [PubMed ID: 8316394].
-
28.
Randall LO, Selitto JJ. A method for measurement of analgesic activity on inflamed tissue. Arch. Int. Pharmacodyn. Ther. 1957;111:409-19. [PubMed ID: 13471093].
-
29.
Baluchnejadmojarad T, Roghani M, Khastehkhodaie Z. Chronic treatment of silymarin improves hyperalgesia and motor nerve conduction velocity in diabetic neuropathic rat. Phytother Res. 2010;24:1120-1125. [PubMed ID: 19960427].
-
30.
Roghani M, Baluchnejadmojarad T. Chronic epigallocatechin-gallate improves aortic reactivity of diabetic rats: underlying mechanisms. Vascul. Pharmacol. 2009;51:84-89. [PubMed ID: 19393342].
-
31.
Freshwater JD, Svensson CI, Malmberg AB, Calcutt NA. Elevated spinal cyclooxygenase and prostaglandin release during hyperalgesia in diabetic rats. Diabetes. 2002;51:2249-2255. [PubMed ID: 12086957].
-
32.
Fox A, Eastwood C, Gentry C, Manning D, Urban L. Critical evaluation of the streptozotocin model of painful diabetic neuropathy in the rat. Pain. 1999;81:307-316. [PubMed ID: 10431718].
-
33.
Dobretsov M, Hastings SL, Stimers JR, Zhang JM. Mechanical hyperalgesia in rats with chronic perfusion of lumbar dorsal root ganglion with hyperglycemic solution. J. Neurosci. Methods. 2001;110:9-15. [PubMed ID: 11564519].
-
34.
Malcangio M, Tomlinson DR. A pharmacologic analysis of mechanical hyperalgesia in streptozotocin-diabetic rats. Pain. 1998;76:151-157. [PubMed ID: 9696468].
-
35.
Sasaki T, Yasuda H, Maeda K, Kikkawa R. Hyperalgesia and decreased neuronal nitric oxide synthase in diabetic rats. Neuroreport. 1998;9:243-247. [PubMed ID: 9507963].
-
36.
Rutledge LP, Ngong JM, Kuperberg JM, Samaan SS, Soliman KF, Kolta MG. Dopaminergic system modulation of nociceptive response in long-term diabetic rats. Pharmacol. Biochem. Behav. 2002;74:1-9. [PubMed ID: 12376147].
-
37.
Gullapalli S, Gurumoorthy K, Kaul CL, Ramarao P. Role of L-type Ca2+ channels in attenuated morphine antinociception in streptozotocin-diabetic rats. Eur. J. Pharmacol. 2002;435:187-194. [PubMed ID: 11821025].
-
38.
Shibata M, Ohkubo T, Takahashi H, Inoki R. Modified formalin test: characteristic biphasic pain response. Pain. 1989;38:347-352. [PubMed ID: 2478947].
-
39.
Rosland JH, Tjolsen A, Maehle B, Hole K. The formalin test in mice-effect of formalin concentration. Pain. 1990;42:235-242. [PubMed ID: 2247320].
-
40.
Waltner-Law ME, Wang XL, Law BK, Hall RK, Nawano M, Granner DK. Epigallocatechin gallate, a constituent of green tea, represses hepatic glucose production, J. Biol. Chem. 2002;277:34933-34940.
-
41.
Koyama Y, Abe K, Sano Y, Ishizaki Y, Njelekela M, Shoji Y, Hara Y, Isemura M. Effects of green tea on gene expression of hepatic gluconeogenic enzymes in-vivo. Planta Med. 2004;70:1100-1102. [PubMed ID: 15549673].
-
42.
Collins QF, Liu HY, Pi J, Liu Z, Quon MJ, Cao W. Epigallocatechin-3-gallate (EGCG), a green tea polyphenol, suppresses hepatic gluconeogenesis through 5-AMP-activated protein kinase. J. Biol. Chem. 2007;282:30143-30149. [PubMed ID: 17724029].
-
43.
Toleikis PM, Godin DV. Alteration of antioxidant status in diabetic rats by chronic exposure to psychological stressors. Pharmacol. Biochem. Behav. 1995;52:355-366. [PubMed ID: 8577802].
-
44.
Sen CK. Oxygen toxicity and antioxidants: state of the art. Indian J. Physiol. Pharmacol. 1995;39:177-196. [PubMed ID: 8550109].
-
45.
Coppey LJ, Gellett JS, Davidson EP, Dunlap JA, Yorek MA. Effect of treating streptozotocin-induced diabetic rats with sorbinil, myo-inositol or aminoguanidine on endoneurial blood flow, motor nerve conduction velocity and vascular function of epineurial arterioles of the sciatic nerve. Int. J. Exp. Diabetes Res. 2002;3:21-36. [PubMed ID: 11900277].
-
46.
Higdon JV, Frei B. Tea catechins and polyphenols: health effects, metabolism, and antioxidant functions. Crit. Rev. Food Sci. Nutr. 2003;43:89-143. [PubMed ID: 12587987].
-
47.
Hasegawa M. Biochemistry and molecular biology of tauopathies. Neuropathology. 2006;26:484-490. [PubMed ID: 17080729].
-
48.
Kondo K, Kurihara M, Miyata N, Suzuki T, Toyoda M. Scavenging mechanisms of (-)-epigallocatechin gallate and (-)-epicatechin gallate on peroxyl radicals and formation of superoxide during the inhibitory action. Free Radic. Biol. Med. 1999;27:855-863. [PubMed ID: 10515590].
-
49.
Guo Q, Zhao B, Li M, Shen S, Xin W. Studies on protective mechanisms of four components of green tea polyphenols against lipid peroxidation in synaptosomes. Biochim. Biophys. Acta. 1996;1304:210-222. [PubMed ID: 8982267].
-
50.
Van Acker SA, Tromp MN, Haenen GR, van dV, Bast A. Flavonoids as scavengers of nitric oxide radical. Biochem. Biophys. Res. Commun. 1995;214:755-759. [PubMed ID: 7575540].
-
51.
Lin YL, Lin JK. (-)-Epigallocatechin-3-gallate blocks the induction of nitric oxide synthase by down-regulating lipopolysaccharide-induced activity of transcription factor nuclear factor-kappaB. Mol. Pharmacol. 1997;52:465-472. [PubMed ID: 9281609].
-
52.
Chan MM, Fong D, Ho CT, Huang HI. Inhibition of inducible nitric oxide synthase gene expression and enzyme activity by epigallocatechin gallate, a natural product from green tea. Biochem. Pharmacol. 1997;54:1281-1286. [PubMed ID: 9393670].
-
53.
Patil L, Balaraman R. Effect of Green Tea Extract on Doxorubicin Induced Cardiovascular Abnormalities: Antioxidant Action. Iranian J. Pharm. Res. 2011;10:89-96.
-
54.
Sano M, Takahashi Y, Yoshino K, Shimoi K, Nakamura Y, Tomita I. Effect of tea (Camellia sinensis L.) on lipid peroxidation in rat liver and kidney: a comparison of green and black tea feeding. Biol. Pharm. Bull. 1995;18:1006-1008. [PubMed ID: 7581239].