Abstract
Keywords
Hippocampus Bicuculline Muscimol State-dependent Memory Mice
Introduction
In the step down inhibitory avoidance task, animals learn to avoid stepping down from a platform on a grid floor where they once received a footshock. It has been reported that this task relies heavily on the dorsal hippocampus (1, 2). A number of studies have indicated that several neurotransmitter systems are involved in hippocampl-dependent learning and memory (1, 3).
Gama-aminobutyric acid (GABA) is the main central inhibitory neurotransmitter which acts through the interaction with GABAA, GABAB and GABAC receptors (4-6). The GABAA and GABAC receptors are ionotropic which directly associated with a Cl- channel (7), and mediate rapid synaptic inhibition (8, 9). The GABAB receptors are coupled to the K+ channels via G proteins and mediate the late component of inhibitory postsynaptic potentials (6, 10, 11). GABA plays a controlling role on the balance of excitability and inhibitory states in the cortex and hippocampus (12). It has been reported that GABAA receptors are responsible for mediating a wide range of activities, including anticonvulsant (13), sedation and hypnosis (14). It is also well known that the GABAA receptors affect learning and memory processes (15, 16). GABAA receptor agonists impair memory while their antagonists facilitate retrieval in different tasks (3, 15, 17-19).
Lithium has been used as an important mood stabilizing drug in the treatment of manic-depressive illness and can potentiate the effects of antidepressant drugs (20-22). In addition to its established role as a mood stabilizer, a neuroprotective (23, 24) and an antiapoptotic effect (25, 26) for lithium have been reported. Furthermore, clinical observations have suggested that lithium may exert adverse effects on memory. It has been reported that the drug treatment inhibited learning, memory, and speed of information processing in bipolar disorder patients and to some extent in control subjects (27-30). We have already reported a state-dependent learning induced by lithium (31-33). We have also reported a possible involvement of nitric oxide (31), μ-opioid receptors (34) and dopaminergic system (32) in lithium-induced state-dependent learning. Considering role of the dorsal hippocampus in mediating inhibitory avoidance memory (1), and involvement of a GABA signaling in the effect of lithium (35), this study was conducted to understand the involvement of GABAA receptors of the dorsal hippocampus in state-dependent learning induced by lithium.
Experimental
Materials
Drugs
The drugs used in the present study were lithium chloride (Merck, Germany), muscimol (Tocris, UK), bicuculline (Sigma, St. Louis, CA, USA). All drugs were dissolved in sterile 0.9% saline, just before the experiment, except for bicuculline that was dissolved in one drop of glacial acetic acid and made up to a volume of 2 mL with sterile 0.9% saline and then diluted to the required volume. Muscimol and bicuculline were injected into the CA1 regions of the dorsal hippocampi (intra-CA1) and lithium was injected intraperitoneally (IP). Control animals received either saline and/or vehicle.
Methods
Subjects
Male albino NMRI mice (Pasteur Institute; Tehran, Iran) weighing 20-30 g were used. The animals were housed 10 per a Plexiglas cage and maintained in a room with controlled light/dark cycle (12/12 h with light beginning at 7:00 a.m.) and temperature (22 ± 2 °C), with free access to food and water. They were allowed to adapt to the laboratory conditions for at least one week before surgery. The experiments were carried out during the light phase of the photoperiod. Ten animals were used in each group of experiments and each animal was used once only. In this research, 25 groups were examined. The research adhered to the Principles of Laboratory Animal Care (NIH publication #85−23, revised in 1985)
Surgery and cannulae implantation
Mice were anesthetized with intraperitoneal injection of ketamine/xylazine mixture (100 and 10 mg/kg, respectively), and placed in a stereotaxic frame (Stoelting Co. USA). The skin was incised and the skull was cleaned. Two 23-gauge guide cannulae were placed (bilaterally) 1 mm above the intended site of injection according to the atlas of Paxinos and Franklin (2001). Stereotaxic coordinates for the CA1 regions of the dorsal hippocampus were AP: -2 mm from bregma, L: ±1.6 from the midline, and V: -1.5 mm from the skull surface. The guide cannulae were anchored to the skull by a jeweler’s screw and dental cement, and then two stainless steel stylets (30-gauge) were inserted into the guide cannulae to keep them patent prior to injections. All animals were allowed one week to recover from the surgery.
Intra-hippocampal microinjections
For drug infusions, the animals were gently restrained by a hand; the stylets were removed from the guide cannulae and a 30-gauge injection needle was inserted (1 mm beyond the tip of the guide cannula). The injection solutions were administered in a total volume of 1 μL/mouse (0.5 μL/each side) over a 60 sec period followed by an additional 60 sec to facilitate the diffusion of the drugs from the tip of the guide cannula.
Apparatus
The inhibitory avoidance apparatus consisted of a wooden box (30×30×40 cm height). The floor of which was consisted of stainless steel rods (29 parallel rods, 0.3 cm in diameter, set 1 cm apart). A wooden platform (4×4×4 cm) was set in the center of the grid floor. Intermittent electric shocks (1 Hz, 0.5 sec, 40 V DC) were delivered to the grid floor by an isolated stimulator (Grass S44, USA).
Inhibitory avoidance task
A single-trial step-down inhibitory avoidance task was used. Each mouse was gently placed on the wooden platform. When the mouse stepped down from the platform and placed all its four paws on the grid floor, the electric shock was delivered continuously for 15 sec. This training procedure was carried out between 9:00 and 13:00 h. Each mouse was placed on the platform again at 24 h after training and the step-down latency was recorded with a stopwatch as inhibitory avoidance memory. An upper cut-off time of 300 sec was set. The retention test was also carried out between 9:00 and 13:00 h.
Drug treatment
Ten animals were used in each experimental group. In the experiments where animals received two injections, the control groups also received two saline or vehicle injections. The drug doses for lithium and GABAA agents were selected according to pilot experiments and our previous studies (32-37).
Experiment 1
In this experiment effects of post-training administration of lithium on inhibitory avoidance memory, and then effects of pre-test administration of lithium on memory impairment induced by lithium (10 mg/kg) given after training were examined. Four groups of Animals were used in this experiment. One group of animals received post-training and pre-test saline administration. The other groups received immediate post-training lithium (10 mg/kg), and on the test day they received saline or different doses of lithium (5 or 10 mg/kg, IP) 45 min prior to the test.
Experiment 2
This experiment examined effect of intra-CA1 administration of GABAA receptor antagonist, bicuculline, on memory retrieval by the lower dose of lithium. Nine groups of animals were used in this experiment. One group received injections of saline (10 mL/kg) both post-training and pre-test. The other eight groups of animals received lithium (10 mg/kg) after training and divided in two sets with four groups. On the test day, the animals of the first set received saline (10 mL/kg) 45 min before testing plus intra-CA1 injections of vehicle or bicuculline (0.062, 0.125 or 0.25 μg/mouse) five min before testing. The animals of the second set received LiCl (5 mg/kg) 45 min before testing plus intra-CA1 injections of vehicle or bicuculline (0.062, 0.125 or 0.25 μg/mouse) five min prior to the test.
Experiment 3
This experiment examined effect of intra-CA1 administration of GABAA receptor agonist, muscimol, on memory retrieval by the lithium. Twelve groups of animals were used in this experiment. One group received injections of saline (10 mL/kg) both post-training and pre-test. Three groups of the animals received post-training saline, and on the test day they received muscimol (0.01, 0.03 or 0.06 μg/mouse, intra-CA1) five min prior to testing. The other eight groups of animals were received post-training lithium (10 mg/kg) and divided in two sets with four groups. On the test day, the animals of the first set received saline (10 mL/kg) 45 min before testing plus intra-CA1 injections of saline or muscimol (0.01, 0.03 or 0.06 μg/mouse) five min prior to testing. The animals of the second set received LiCl (10 mg/kg) 45 min before testing plus intra-CA1 injections of saline or muscimol (0.01, 0.03 or 0.06 μg/mouse) five min before to the test.
Data analysis
Because of the individual variations, the data were analyzed by one-way analysis of variance (ANOVA) for non-parametrical data (Kruskal-Wallis) followed by a two-tailed Mann-Whitney’s U-test. Then, Holmes Sequential Bonferroni correction was done for paired comparisons. The step-down latencies (as an index of inhibitory avoidance memory) for each experimental group were expressed as the median ± quartiles. In all statistical evaluations, p < 0.05 was used as the criterion for statistical significance.
Results
State-dependent learning induced by lithium
As shown in Figure 1, post-training administration of lithium (10 mg/kg) decreased the step-down latency on the test day compared with saline-treated animals, and the decrease in the step-down latency due to post-training lithium was fully or partly reversed by pre-test administration of the drug [Kruskal–Wallis non-parametric ANOVA, H(3) = 17.97, p < 0.001]; suggesting state-dependent learning induced by lithium (Figure 1)
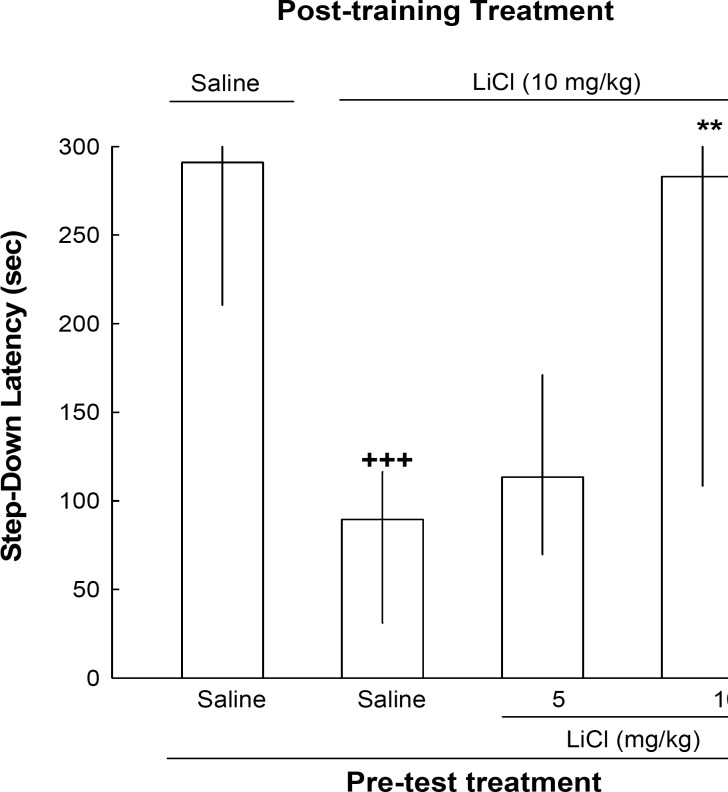
Effect of pre-test intra-CA1 injections of bicuculline on reversal of state-dependent learning by a lower dose of lithium
The result of experiment 2 showed that pre-test administration of a lower dose of lithium (5 mg/kg) in combination with different doses of intra-CA1 administration of bicuculline (0.062, 0.125 and 0.25 μg/mouse) increased the step-down latency compared to the control group [Kruskal–Wallis non-parametric ANOVA, H(8) = 29.92, p < 0.001]. Post-hoc analysis revealed that bicuculline at dose of 0.25 μg/mouse increased the effect of pre-test lithium (5 mg/kg) on reversal of step-down latency (Figure 2).
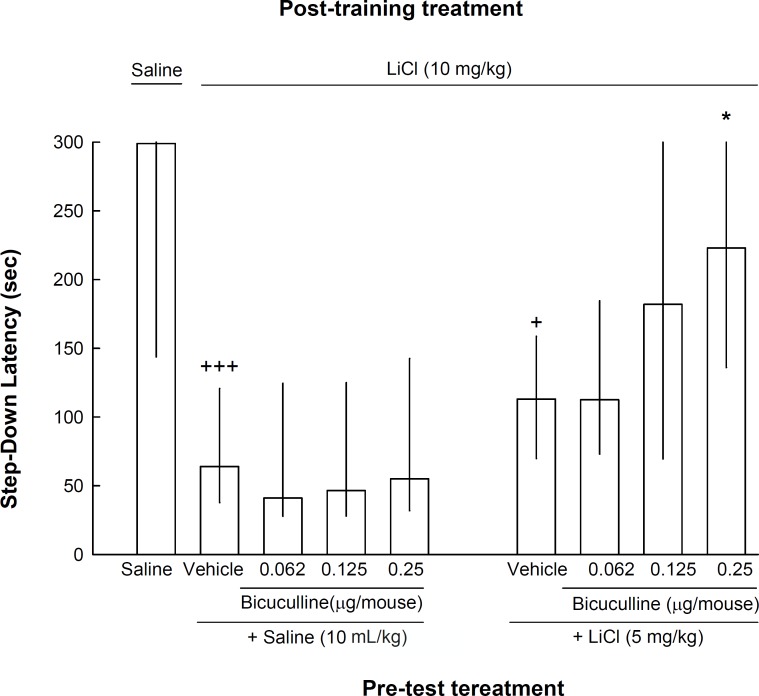
Effect of muscimol on state-dependent learning induced by lithium
The result of experiment 3 revealed that pre-test administration of muscimol had no significant effect on step-down latency of post-training saline treated animals. On the other hand, although muscimol (0.06 μg/mouse) reversed the decrease of step-down latency induced by post-training lithium (10 mg/kg), but it disrupted reversal effect of pre-test lithium (10 mg/kg) on state-dependent learning [Kruskal–Wallis non-parametric ANOVA, H(11) = 41.47, p < 0.001] (Figure 3).
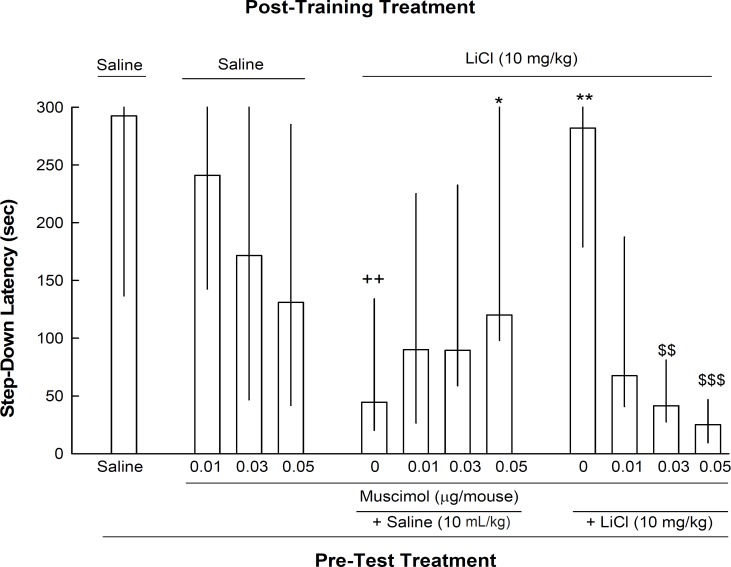
Discussion
Consistent with our previous studies (31, 34), the present data showed that post-training administration of lithium decreased step-down latency of inhibitory avoidance task in mice, which was fully or partly reversed by pre-test administration of the drug. This effect of lithium on the step-down latency seems to be due to state-dependent learning (31-34). In state-dependent learning when pre- or post-training administration of a drug decreases memory for a task, administration of the drug prior to testing reinstates the memory for the task (38-40). State-dependent learning has been reported for many drugs and hormones (41-44). With the idea of state-dependent learning, in animals which received post-training lithium and pre-test saline in the present study, the decrease of the step-down latency may be due to this fact that the animals did not receive lithium before the test. However, the exact mechanism of state-dependent learning induced by drugs, including lithium, will require more investigations.
It has been reported that lithium which is used in treatment of bipolar disorders may increase GABA signaling (35). On the other hand, several lines of experimental data indicate that GABAA receptors are involved in learning and memory processes (3, 12, 45, 46). Therefore, in the present research we examined effects of intra-CA1 injections of the GABAA receptor agents on state-dependent learning induced by lithium. At first, we injected different doses of lithium with the GABAA receptor antagonist and agonist (bicuculline and muscimol, respectively) and we observed that bicuculline and muscimol showed different effects by themselves and in combination with lithium.
The results of the present study showed that intra-CA1 injections of the GABAA receptor antagonist, bicuculline before testing partly increased the effect of lower dose of lithium on reinstatement of the step-down latency. A positive effect for bicuculline on memory has been also reported in other studies. It has been reported that post-training administration of bicuculline increased memory retention in rat (46). Bicuculline, when administered into the amygdala after training, blocked benzodiazepine-induced amnesia (47). However, lower doses of bicuculline induced amnesia at both low and high footshock intensities (45).
The present results also revealed that in the post-training saline treated animals, intra-CA1 injections of the GABAA receptor agonist, muscimol before testing had no significant effect on the step-down latency. In the post-training lithium treated animals, pre-test intra-CA1 injection of muscimol reversed the decrease of step-down latency due to post-training lithium. However, pre-test intra-CA1 injection of the same dose of muscimol disrupted state-dependent learning induced by lithium.
It has been also shown that post-training intrahippocampal injection of muscimol decreased memory retention of inhibitory avoidance in rats dose-dependently (46). The impairment and enhancement of retention of an inhibitory avoidance task by muscimol and bicuculline, respectively, was blocked in animals with amygdala and dorsal hippocampus lesions (48). It has been reported that amygdaloid GABA neurotransmission or mRNA transcription controls footshock-associated fear using a fear passive avoidance task (49). Unilateral microinjection of midazolam into the basolateral amygdala (via a GABAergic mechanism) reduced avoidance responses (50). The above cited data may further support that the GABAA receptors in the hippocampus are important for inhibitory avoidance memory. It has been reported that immediate post-training systemic injections of bicuculline improved and muscimol impaired retention of inhibitory avoidance task in CD1 mice, which did not reversed by pre-test administration of the same drugs (51). According to the present results and the above cited data it seems that the effects of post-training administration of bicuculline and muscimol on retention are not state dependence.
Taken together our results about effects of bicuculline and muscimol on inhibitory avoidance memory are consistent with other reports that bicuculline showed a positive effect but muscimol induced a negative effect. Since pre-test administration of bicuculline into the CA1 region of the hippocampus could increase the effect of pre-test lithium on reversal of memory, one may propose that sate-dependent learning induced by lithium to be dependent at least partly on a GABAergic mechanism in the hippocampus. In addition, state-dependent learning induced by lithium disrupted due to pre-test injections of muscimol which may further support the involvement of GABAA receptors of the hippocampus in effects of lithium on inhibitory avoidance memory.
References
-
1.
Izquierdo I, Bevilaqua LR, Rossato JI, Bonini JS, Medina JH, Cammarota M. Different molecular cascades in different sites of the brain control memory consolidation. Trends Neurosci. 2006;29:496-505. [PubMed ID: 16872686].
-
2.
Izquierdo I, Medina JH. Memory formation: the sequence of biochemical events in the hippocampus and its connection to activity in other brain structures. Neurobiol. Learn. Mem. 1997;68:285-316. [PubMed ID: 9398590].
-
3.
Etemadi L, Sahraei H, Ghoshooni H, Oryan S, Eidi M, Eidi A. The effect of GABAB receptor activation within the ventral tegmental area on morphine-induced incentive sensitization in female rats. Iranian J. Pharm. Res. 2004;Suppl. 1:1-32.
-
4.
Hill DR, Bowery NG. 3H-baclofen and 3H-GABA bind to bicuculline-insensitive GABA B sites in rat brain. Nature. 1981;290:149-52. [PubMed ID: 6259535].
-
5.
Lobo IA, Harris RA. GABA(A) receptors and alcohol. Pharmacol. Biochem. Behav. 2008;90:90-4. [PubMed ID: 18423561].
-
6.
Bormann J. Electrophysiology of GABAA and GABAB receptor subtypes. Trends Neurosci. 1988;11:112-6. [PubMed ID: 2465608].
-
7.
Bormann J. The ‘ABC’ of GABA receptors. Trends Pharmacol. Sci. 2000;21:16-9. [PubMed ID: 10637650].
-
8.
McDonald BJ, Moss SJ. Differential phosphorylation of intracellular domains of gamma-aminobutyric acid type A receptor subunits by calcium/calmodulin type 2-dependent protein kinase and cGMP-dependent protein kinase. J. Biol. Chem. 1994;269:18111-7. [PubMed ID: 8027073].
-
9.
Benke D, Honer M, Michel C, Bettler B, Mohler H. gamma-aminobutyric acid type B receptor splice variant proteins GBR1a and GBR1b are both associated with GBR2 in situ and display differential regional and subcellular distribution. J. Biol. Chem. 1999;274:27323-30. [PubMed ID: 10480953].
-
10.
Nicoll RA, Malenka RC, Kauer JA. Functional comparison of neurotransmitter receptor subtypes in mammalian central nervous system. Physiol. Rev. 1990;70:513-65. [PubMed ID: 1690904].
-
11.
Thompson SM. Modulation of inhibitory synaptic transmission in the hippocampus. Prog. Neurobiol. 1994;42:575-609. [PubMed ID: 7938542].
-
12.
Paulsen O, Moser EI. A model of hippocampal memory encoding and retrieval: GABAergic control of synaptic plasticity. Trends Neurosci. 1998;21:273-8. [PubMed ID: 9683315].
-
13.
Sperk G, Furtinger S, Schwarzer C, Pirker S. GABA and its receptors in epilepsy. Adv. Exp. Med. Biol. 2004;548:92-103. [PubMed ID: 15250588].
-
14.
Lu J, Greco MA. Sleep circuitry and the hypnotic mechanism of GABAA drugs. J. Clin. Sleep Med. 2006;2:S19-26. [PubMed ID: 17557503].
-
15.
Castellano C, McGaugh JL. Retention enhancement with post-training picrotoxin: lack of state dependency. Behav. Neural. Biol. 1989;51:165-70. [PubMed ID: 2539081].
-
16.
Ganjkhani M, Hatam M, Oliveira CVR, Ciriello J. GABAergic receptors in rostral ventrolateral medulla mediates the cardiovascular responses to activation of bed nucleus of the stria terminalis in the female rat. Iranian J. Pharm. Res. 2004;Suppl. 1:77.
-
17.
Brioni JD, McGaugh JL. Post-training administration of GABAergic antagonists enhances retention of aversively motivated tasks. Psychopharmacology (Berl). 1988;96:505-10. [PubMed ID: 3149774].
-
18.
Castellano C, Brioni JD, Nagahara AH, McGaugh JL. Post-training systemic and intra-amygdala administration of the GABA-B agonist baclofen impairs retention. Behav. Neural. Biol. 1989;52:170-9. [PubMed ID: 2552976].
-
19.
Chapouthier G. From the search for a molecular code of memory to the role of neurotransmitters: a historical perspective. Neural Plast. 2004;11:151-8. [PubMed ID: 15656266].
-
20.
Nierenberg AA, Price LH, Charney DS, Heninger GR. After lithium augmentation: a retrospective follow-up of patients with antidepressant-refractory depression. J. Affect. Disord. 1990;18:167-75. [PubMed ID: 2139061].
-
21.
Prien RF, Kupfer DJ, Mansky PA, Small JG, Tuason VB, Voss CB, Johnson WE. Drug therapy in the prevention of recurrences in unipolar and bipolar affective disorders. Report of the NIMH Collaborative Study Group comparing lithium carbonate, imipramine, and a lithium carbonate-imipramine combination. Arch. Gen. Psychiatry. 1984;41:1096-104. [PubMed ID: 6437366].
-
22.
Schou M. Lithium in psychiatric therapy and prophylaxis. J. Psychiatr. Res. 1968;6:67-95. [PubMed ID: 4876759].
-
23.
Chen G, Zeng WZ, Yuan PX, Huang LD, Jiang YM, Zhao ZH, Manji HK. The mood-stabilizing agents lithium and valproate robustly increase the levels of the neuroprotective protein bcl-2 in the CNS. J. Neurochem. 1999;72:879-82. [PubMed ID: 9930766].
-
24.
Chuang DM. Neuroprotective and neurotrophic actions of the mood stabilizer lithium: can it be used to treat neurodegenerative diseases? Crit. Rev. Neurobiol. 2004;16:83-90.
-
25.
Chuang DM. The antiapoptotic actions of mood stabilizers: molecular mechanisms and therapeutic potentials. Ann. N. Y. Acad. Sci. 2005;1053:195-204. [PubMed ID: 16179524].
-
26.
Nonaka S, Katsube N, Chuang DM. Lithium protects rat cerebellar granule cells against apoptosis induced by anticonvulsants, phenytoin and carbamazepine. J. Pharmacol. Exp. Ther. 1998;286:539-47. [PubMed ID: 9655900].
-
27.
Honig A, Arts BM, Ponds RW, Riedel WJ. Lithium induced cognitive side-effects in bipolar disorder: a qualitative analysis and implications for daily practice. Int. Clin. Psychopharmacol. 1999;14:167-71. [PubMed ID: 10435769].
-
28.
Kocsis JH, Shaw ED, Stokes PE, Wilner P, Elliot AS, Sikes C, Myers B, Manevitz A, Parides M. Neuropsychologic effects of lithium discontinuation. J. Clin. Psychopharmacol. 1993;13:268-75. [PubMed ID: 8376614].
-
29.
Pachet AK, Wisniewski AM. The effects of lithium on cognition: an updated review. Psychopharmacology (Berl). 2003;170:225-34. [PubMed ID: 14504681].
-
30.
Stip E, Dufresne J, Lussier I, Yatham L. A double-blind, placebo-controlled study of the effects of lithium on cognition in healthy subjects: mild and selective effects on learning. J. Affect. Disord. 2000;60:147-57. [PubMed ID: 11074103].
-
31.
Zarrindast MR, Mardaneh-Shendy M, Ahmadi S. Nitric oxide modulates state-dependency induced by lithium in an inhibitory avoidance task in mice. Behav. Pharmacol. 2007;18:289-95. [PubMed ID: 17551321].
-
32.
Zarrindast MR, Misaghi S, Ahmadi S. The dopaminergic system plays a role in the effect of lithium on inhibitory avoidance memory in mice. Eur. J. Pharmacol. 2008;590:198-203. [PubMed ID: 18606403].
-
33.
Zarrindast MR, Parsaei L, Ahmadi S. Repeated administration of histamine improves memory retrieval of inhibitory avoidance by lithium in mice. Pharmacology. 2008;81:187-94. [PubMed ID: 18043010].
-
34.
Zarrindast MR, Lahmi A, Ahmadi S. Possible involvement of μ-opioid receptors in effect of lithium on inhibitory avoidance response in mice. J. Psychopharmacol. 2008;22:865-71. [PubMed ID: 18208927].
-
35.
Manji HK, Drevets WC, Charney DS. The cellular neurobiology of depression. Nat. Med. 2001;7:541-7. [PubMed ID: 11329053].
-
36.
Zarrindast MR, Ahmadi S, Haeri-Rohani A, Rezayof A, Jafari MR, Jafari-Sabet M. GABA(A) receptors in the basolateral amygdala are involved in mediating morphine reward. Brain Res. 2004;1006:49-58. [PubMed ID: 15047023].
-
37.
Rezayof A, Razavi S, Haeri-Rohani A, Rassouli Y, Zarrindast MR. GABA(A) receptors of hippocampal CA1 regions are involved in the acquisition and expression of morphine-induced place preference. Eur. Neuropsychopharmacol. 2007;17:24-31. [PubMed ID: 16624534].
-
38.
Overton DA. Historical context of state dependent learning and discriminative drug effects. Behav. Pharmacol. 1991;2:253-64. [PubMed ID: 11224069].
-
39.
Shulz DE, Sosnik R, Ego V, Haidarliu S, Ahissar E. A neuronal analogue of state-dependent learning. Nature. 2000;403:549-53. [PubMed ID: 10676963].
-
40.
Overton DA. Historical context of state dependent learning and discriminative drug effects. Behav. Pharmacol. 1991;2:253-64. [PubMed ID: 11224069].
-
41.
Bruins Slot LA, Colpaert FC. Opiate states of memory: receptor mechanisms. J. Neurosci. 1999;19:10520-9. [PubMed ID: 10575048].
-
42.
Bruins Slot LA, Colpaert FC. Recall rendered dependent on an opiate state. Behav. Neurosci. 1999;113:337-44. [PubMed ID: 10357458].
-
43.
Colpaert FC. Amnesic trace locked into the benzodiazepine state of memory. Psychopharmacology (Berl). 1990;102:28-36. [PubMed ID: 1975447].
-
44.
Izquierdo I, Dias RD. Endogenous state-dependency:memory regulation by post-training and pre-testing administration of ACTH, beta -endorphin, adrenaline and tyramine. Braz. J. Med. Biol. Res. 1983;16:55-64. [PubMed ID: 6315115].
-
45.
Cruz-Morales SE, Quirarte GL, Diaz del Guante MA, Prado-Alcala RA. Effects of GABA antagonists on inhibitory avoidance. Life Sci. 1993;53:1325-30. [PubMed ID: 8412493].
-
46.
Zarrindast MR, Bakhsha A, Rostami P, Shafaghi B. Effects of intrahippocampal injection of GABAergic drugs on memory retention of passive avoidance learning in rats. J. Psychopharmacol. 2002;16:313-9. [PubMed ID: 12503830].
-
47.
Dickinson-Anson H, McGaugh JL. Bicuculline administered into the amygdala after training blocks benzodiazepine-induced amnesia. Brain Res. 1997;752:197-202. [PubMed ID: 9106457].
-
48.
Ammassari-Teule M, Pavone F, Castellano C, McGaugh JL. Amygdala and dorsal hippocampus lesions block the effects of GABAergic drugs on memory storage. Brain Res. 1991;551:104-9. [PubMed ID: 1913142].
-
49.
Van Nobelen M, Kokkinidis L. Amygdaloid GABA, not glutamate neurotransmission or mRNA transcription controls footshock-associated fear arousal in the acoustic startle paradigm. Neuroscience. 2006;137:707-16. [PubMed ID: 16289581].
-
50.
Harris JA, Westbrook RF. Effects of benzodiazepine microinjection into the amygdala or periaqueductal gray on the expression of conditioned fear and hypoalgesia in rats. Behav. Neurosci. 1995;109:295-304. [PubMed ID: 7619319].
-
51.
Castellano C, McGaugh JL. Effects of post-training bicuculline and muscimol on retention: lack of state dependency. Behav. Neural. Biol. 1990;54:156-64. [PubMed ID: 2173543].