Abstract
Keywords
Topical bioadhesive gel Aceclofenac Three-level factorial design Design HPMC
Introduction
Osteoarthritis (OA) is the most prevalent disease after the age of 65 in about 60% of men and 70% of women (1). OA is primarily a non-inflammatory degenerative joint disease characterized by progressive loss of articular cartilage, subchondrial bone sclerosis, osteophyte formation, changes in the synovial membrane, and an increased volume of synovial fluid with reduced viscosity and hence, changed lubrication properties. Current treatment options for OA are limited. They include intra-articular (IA) injection of glucocorticoids and hyaluronic acid (HA) preparations or symptomatic treatment with simple non-steroidal anti-inflammatory drugs (NSAIDs) (2). Due to the localized nature of the disease, the IA injection of glucocorticoids and HA seems to be an attractive approach for OA, but they provide only short-term pain relief and/or often do not provide adequate pain relief and have no patient compliance (2). On the other hand, Rheumatoid arthritis (RA) is a common chronic systemic inflammatory disease that is primarily characterized by inflammation of the synovium with destruction of cartilage and bone as the disease progresses. More severe disease may be associated with vasculitis, pericarditis, pleural effusion, pulmonary interstitial fibrosis, peripheral neuropathies, subcutaneous and pulmonary nodules and scleritis (3, 4). Since there is no cure, symptomatic treatment is the only choice to reduce the pain and inflammation. Formulations of NSAIDs are the first choice remedies which would fulfill the need of providing significantly long remission of pain with improved patient compliance.
Aceclofenac is a potent non-steroidal anti-inflammatory drug that prevents prostaglandin synthesis by inhibiting enzyme cyclooxygenase. Aceclofenac is widely used for the treatment of rheumatoid arthritis, osteoarthritis and ankylosing spondylitis (5-7). However, the oral administration of aceclofenac has often resulted in side effects, including gastrointestinal ulcer and anemia due to gastrointestinal bleeding (6). It requires frequent oral dosing because of its short half-life (3-4 h). Topical administration of aceclofenac would be a possible alternative offering distinct advantages such as elimination of the absorption variable rate, first pass intestinal and hepatic metabolism inherent with oral dosing and delivering the drug directly to the inflamed site and thereby, producing high local concentrations and avoiding the side effects (7).
The skin is the largest organ of the body, accounting for more than 10% of body mass. It has important protective and homeostatic roles and is generally regarded as a critical protective barrier to the external environment (9). A drug molecule will encounter several diffusional resistances in the course of skin permeation. The stratum corneum (SC), the outermost layer of the epidermis, is the rate limiting membrane to percutaneous delivery (10). To be therapeutically beneficial, the barrier properties of skin must be modified in such a way that the drug can be administered at a sufficiently high rate to achieve a therapeutically effective level in the proper site. There are several approaches which can be utilized to alter the barrier properties of the skin and so the percutaneous permeation rate of drugs (11). One of the useful approaches is the co-administration of skin permeation enhancers. Nowadays, topical drug delivery is a well-accepted way of delivering drugs locally and currently topical gels are used to treat the pain and inflammation in rheumatoid arthritis, osteoarthritis and ankylosing spondylitis and also in various skin diseases.
The topical administration of bioadhesive gels has distinct advantages over the other delivery system (such as good accessibility) and can be applied for localized purposes. Because of its excellent accessibility, the self-administration of a drug is possible and can be stopped at any time (12). Some studies have shown that after the topical application, significant levels of anti-inflammatory drugs were found in deep tissues such as facia, muscle, and synovium, which is a desirable feature for the relief of local symptoms (13). Rabinowitz et al. (1982) found that in articular tissues and synovial fluids, topical application of salicylates results in higher drug concentrations than that of oral aspirin in dogs (14). Topical bioadhesive gels of various drugs have been developed such as flurbiprofen, benzocaine, tetracaine, ketamine, piroxicam, tretinoin and griseofulvin (13, 15-19). Literature survey indicates that topical bioadhesive thermo sensitive gel for aceclofenac has not been investigated yet.
The optimum rheological properties are required for the gel to ease the application on the skin. The high viscosity of the gel makes it difficult to apply on the skin. However, low viscosity leads to rapid loss of gel from the application site. Therefore, ideal gel should be initially in fluid state for the ease of application and after being applied on the body at 37°C, it turns into the viscous gel state and shows the bioadhesiveness (20). It has been known that poloxamer exhibits the phenomenon of reverse thermal gelation, remaining as a clear solution from 20% to 30% (w/w) at low temperature and it forms a gel on warming at room temperature by undergoing sol-gel transition (21, 22). Therefore, it was proposed to develop a topical bioadhesive gel system of aceclofenac with Poloxamer 407 (PL407) and hydroxypropyl methylcellulose (HPMC) as polymers. Poloxamer 407, a non-toxic copolymer with average molecular weight of 11500, contains 70% hydrophilic ethylene oxide units and 30% hydrophobic propylene oxide units. However, in the development of formulation containing a drug that was poorly water-soluble and solubilized in the aqueous medium by poloxamers, the bioadhesive polymers such as carbopol, polycarbophil and sodium alginate could not be used, since the drug was precipitated in the preparation (23). HPMC was used to control drug release from several pharmaceutical systems due to its non-toxic nature and swelling properties.
As a result, the aim of the present study was to formulate and evaluate the topical bioadhesive thermo-sensitive gel of aceclofenac using the combination of Poloxamer 407 and HPMC. In the development of pharmaceutical dosage form with appropriate characteristics, an important issue is to design an optimized pharmaceutical formulation in a short time of period with minimum trials. To that end, response surface methodology (RSM) is currently gaining attention to identify and quantify the effect of different formulation variables on the important characteristics. In this study, a 3-level factorial design was employed to determine the effect of independent variables such as the amount of PL-407 and HPMC on the formulation characteristics such as bioadhesion, rheological properties and in-vitro release studies.
Experimental
Material
Aceclofenac and HPMC K100 M were generously gifted by Lupin Labs (Pune, India).
Poloxamer 407 (Lutrol F 127) was obtained from BASF (BASF, India). All other chemicals used were of analytical grade.
Experimental design
A 3-level factorial design was used to study the effect of two formulation variables on characteristics of topical gel such as bioadhesiveness, viscosity and in-vitro release studies. The amount of Poloxamer 407 and HPMC were the two numeric factors (independent variables). The studied responses (dependent variables) were bioadhesion, consistency index (rheological property) and percentage of drug release in 8 h. Dependent and independent variables along with their levels were listed in Table 1. Experimental design for preparation of different batches of bioadhesive topical gel was summarized in Table 2.
Factors (independent variables), factor levels and responses (dependent variables) used in 3-level factorial experimental design
Factors | Type of factor | Factor level used | Response | ||
---|---|---|---|---|---|
X1- Amount of Poloxamer 407 in gel (%w/w) | Numeric | 20 | 25 | 30 | Y1- Bioadhesive character (in gf) |
X2- Amount of HPMC in gel (%w/w) | Numeric | 2 | 3 | 4 | Y2- Consistency index (in dyne/cm sq) |
Y3- CPR in 2 h (%) | |||||
Y4- CPR in 8 h (%) |
Experimental design for preparation of different batches
Std | Run | Factors | |
---|---|---|---|
X1- %w/w Poloxamer 407 in gel | X2- %w/w HPMC in gel | ||
4 | 1 | 20 | 3 |
5 | 2 | 25 | 3 |
10 | 3 | 25 | 3 |
9 | 4 | 30 | 4 |
3 | 5 | 30 | 2 |
6 | 6 | 30 | 3 |
12 | 7 | 25 | 3 |
13 | 8 | 25 | 3 |
8 | 9 | 25 | 4 |
11 | 10 | 25 | 3 |
7 | 11 | 20 | 4 |
1 | 12 | 20 | 2 |
2 | 13 | 25 | 2 |
Preparation of HPMC-PL407 gel containing aceclofenac
PL407 possesses reverse thermal gelling property and therefore, the gel containing PL407 was prepared by cold method (24, 25). Weighed PL407 was added slowly in cold distilled water (approximately 5°C controlled by using ice bath) with continuous stirring and HPMC was dissolved in normal distilled water and stirred, simultaneously. Then, the HPMC solution was added to PL407 solution with stirring. Accurately weighed aceclofenac was dissolved in 30 mL of ethanol and the ethanolic solution of drug was added slowly in the previously prepared polymer gel with continuous stirring at 400-600 rpm. The volume was made up to 100 g with distilled water. The prepared gel was kept for 24 h at refrigerator temperature for complete polymer desolvation.
Evaluation of topical bioadhesive gel
Drug content uniformity of aceclofenac bioadhesive gels
All prepared gels were analyzed for the desired range of aceclofenac content and the samples which come within the range of 100 ± 10 were taken for in-vitro release studies. A hundred mg of gel was taken in 10 mL of water and mixed for 15 min (25). After filtration, 0.5 mL of solution was diluted to 5 mL by means of water and the absorbance of the solution was measured at 275 nm spectrophotometrically (Hitachi UV-Spectrophotometer). The experiments were done in triplicate.
Bioadhesive testing by modified arm balance method
The bioadhesive testing has been performed using modified two arm balance methods as described in our previous work. Briefly, the weighed gel (0.5 g) was placed on the intestine glued to the upper side of the lower plate. Then, the upper plate was placed over the lower plate and 50 g preload force (or contact pressure) was applied for 5 min (preload time). After the removal of preload force, the water kept in a bottle at some height was siphoned in the container at the rate of 10 mL per min till the plates were detached from each other. The rate of water dropping was controlled with an on/off switch identical to the infusion bottle. The weight of water required for detachment of glass plates was considered as the bioadhesion force of the applied gel (26).
Rheological studies
The samples were placed in beaker and were allowed to equilibrate for 30 min before measuring the viscosity. Viscosity determinations of the prepared formulations were carried out by Brookfield Rheometer fitted with spindle 61 at angular velocity 5, 10 and 20 rpm at room temperature. The average of the readings was used to calculate the viscosity (27). The consistency index and flow index were calculated from the Power law equation (28).
τ = Krn
Where: “τ” is shear stress; “r” is shear rate; “K” is consistency index; “n” is flow index.
In-vitro dissolution study of Aceclofenac gels
Accurately weighed gel (equivalent to 15 mg of the drug) was taken in the center of a hollow cylindrical dialysis membrane. This membrane was then folded and hermetically sealed from both the ends. This “dialysis bag” was then hanged with the help of a wire in a 500 mL beaker (13, 29, 30). Two hundred mL of phosphate buffer (PB) with pH of 6.8 was added to dissolution medium. The entire system was kept at 37°C with continuous magnetic stirring at 200 ± 5 rev/min. Sampling of 1 mL was done at predetermined time-intervals of 5, 10, 15, 30, 60, 120, 180, 240, 300, 360 and 420 min. The media volume was maintained by adding equal volumes of fresh media. The concentration of aceclofenac was spectrophotometrically measured at 275 nm. The interference studies were done in order to ascertain that the polymers do not interfere with the analysis of the drug. UV scans of 0.1% drug, 0.1% polymers and combination of polymers and drug (0.1% each) revealed that the drug exhibited λmax at 275 nm in PB with pH of 6.8 and polymer showed no absorbance at the same wavelength.
The saturation-solubility (Cs) of Aceclofenac in PB with pH of 6.8 is 7.53 ± 0.01 mg/mL at 37°C. Sink conditions were maintained for release studies (C < Cs × 0.1). Therefore, final concentration of Aceclofenac after the complete release in PB with pH of 6.8 was maintained less than 75 μg/mL in compliance with the sink condition.
Statistical analysis of the data
Various RSM computations for the current study were performed employing 30 days Trial Version of Design-Expert software (Version 7.1.2, Stat-Ease Inc., Minneapolis, MN). Polynomial models including interaction and quadratic terms were generated for all the response variables using multiple linear regression analysis. Equations were derived and coefficients of interactions were calculated to determine the effect of each variable on the formulation characteristics. Statistical validity of the model was established on the basis of Analysis of variance (ANOVA) and the 3D response graphs were constructed using Design-Expert software.
Results and Discussion
Experiments of 3-level factorial design
Response data for all the 13 experimental runs of 3-level factorial design, performed in accordance with Table 2, are presented in Table 3.
Results for bioadhesion, consistency index and in-vitro drug release studies of prepared topical bioadhesive gel with 3-level factorial experimental design
Run | Response | |||
---|---|---|---|---|
Y1= bioadhesion (gf) | Y2= Consistency Index | Y3 = CPR at 2 | Y4= CPR at 8 | |
1 | 53 | 8881 | 27.57 | 53.8 |
2 | 60 | 10127 | 22.81 | 50.86 |
3 | 61 | 10154 | 22.81 | 50.86 |
4 | 92 | 20653 | 16.66 | 38.17 |
5 | 37 | 5139 | 21.82 | 53.56 |
6 | 70 | 13001 | 17.74 | 44.21 |
7 | 61 | 10092 | 22.97 | 51.14 |
8 | 59 | 10198 | 22.45 | 50.56 |
9 | 85 | 20356 | 19.82 | 44.56 |
10 | 62 | 10154 | 22.34 | 50.38 |
11 | 68 | 16368 | 24.19 | 47.95 |
12 | 15 | 3556 | 39.67 | 66.98 |
13 | 20 | 4563 | 29.78 | 60.53 |
Mathematical modeling
Mathematical relationship was generated between the factors (independent variables) and responses (dependent variables) using the statistical package Design-Expert. First step in mathematical modeling was fitting the experimental data to appropriate model. A suitable model was selected by software on the basis of different parameter obtained from regression analysis such as p-value, adjusted R2, predicted R2 and Predicted Residual Sum of Square (PRESS) value (Tables 4 and 5). Table 3 lists the values of various response parameters of the prepared batches. ANOVA was applied for estimating the significance of model, at 5% significance level. If more than one model was significant (p < 0.05) for the response, the adjusted R2 and PRESS value of the model were compared to select the best mathematical model for that response. Focus on maximizing the value of adjusted R2 and predicted R2. Low PRESS value indicated adequate fitting of model (31). General quadratic equation for two independent variables is as follow:
Y = β0 + β1X1 + β2X2 + β3X1 X2 + β4X12 + β5X22
Where: β0 is the intercept representing the arithmetic averages of all the quantitative outcomes of 13 runs. β1 to β5 are all coefficients calculated from the observed experimental values of Y. X1 and X2 are the coded levels of factors. The terms X1X2 and Xi2 (i є {1, 2}) represent the interaction and quadratic terms, respectively. Coefficients with one factor represent the effect of that particular factor while the coefficients with more than one factor and those with second order terms represent the interaction between those factors and the quadratic nature of the phenomena, respectively. Synergistic effect and antagonistic effect of factor were indicated by positive sign and negative sign in front of that factor term, respectively.
Fit summary of model for the measured responses Y1 (bioadhesion in gf), Y2 (Consistency index in dyne/cm2), Y3 (Cumulative percentage release at 2 h) and Y4 (Cumulative percentage release at 8 h).
Source | Y1 | Y2 | Y3 | Y4 | ||||
---|---|---|---|---|---|---|---|---|
f-value | p-value | f-value | p-value | f-value | p-value | f-value | p-value | |
Linear vs Mean | 105.39 | < 0.0001 | 144.02 | < 0.0001 | 34.06 | < 0.0001 | 148.46 | < 0.0001 |
2 FI vs Linear | 0.034 | 0.8584 | 1.64 | 0.2323 | 9.00 | 0.0127 | 1.76 | 0.2178 |
Quadratic vs 2 FI | 12.71 | 0.0047 | 6.07 | 0.0295 | 23.09 | 0.0008 | 18.21 | 0.0017 |
Model summary statistics of responses to select suitable model to fit data
Source | Linear | 2 FI | Quadratic | |||||||
---|---|---|---|---|---|---|---|---|---|---|
Adj. R2 | Pred. R2 | PRESS | Adj. R2 | Pred. R2 | PRESS | Adj. R2 | Pred. R2 | PRESS | ||
Response | Y1 | 0.9456 | 0.9104 | 529.8 | 0.9398 | 0.8264 | 1027.58 | 0.9833 | 0.9085 | 541.08 |
Y2 | 0.9597 | 0.9382 | 2.183 E + 007 | 0.9622 | 0.9346 | 2.309 E + 007 | 0.9822 | 0.9165 | 2.948 E + 007 | |
Y3 | 0.8464 | 0.6812 | 132.59 | 0.9147 | 0.6934 | 127.53 | 0.9856 | 0.9239 | 31.67 | |
Y4 | 0.9609 | 0.9338 | 41.21 | 0.9637 | 0.9290 | 44.22 | 0.9925 | 0.9630 | 23.04 |
Drug content uniformity study of topical bioadhesive gels of Aceclofenac
All prepared gels were analyzed for aceclofenac content and the content of drug in each prepared TBG ranged from 96% to 104%. The drug content of the prepared TBG was within desired range of 90% to 110% (21).
Effect of formulation variables on bioadhesion
From the p-values presented in Table 4, linear model and quadratic model was found to be significant for bioadhesion. Quadratic model was selected on the basis of maximum value of adj. R2 and low PRESS value indicating adequate fitting of model (Table 5). Quadratic model was significant with model f-value of 142.32 (p-value < 0.0001). The quadratic equation generated by software is as follows:
Y1 = 60.66 + 10.50X1 + 28.83X2 + 0.50X1X2 + 0.71X12 - 8.29X22
Equation reveals that both factors (X1 and X2) affect bioadhesion characteristics of gel significantly. Equations also indicated that the effect of the change in HPMC concentration seems to be more pronounced in comparison with that of the change in PL-407 concentration since the coefficient of factor X2 has a larger value than that of factor X1. The combined effect of factors X1 and X2 can further be elucidated with the help of response surface plots (Figure 1A ), which demonstrated that Y1 varies in a linear fashion with the amount of both the polymers. However, the steeper ascent in the response surface with HPMC (X2) – instead of Poloxamer (X1) – is clearly discernible from response surface plots, indicating that the effect of HPMC is comparatively more pronounced than that of Poloxamer. From this discussion, one can conclude that the bioadhesion may be changed by appropriate selection of the levels of X1 and X2. Figure 1B shows a linear relationship between the observed response values and the predicted values indicating the correctness of the model.
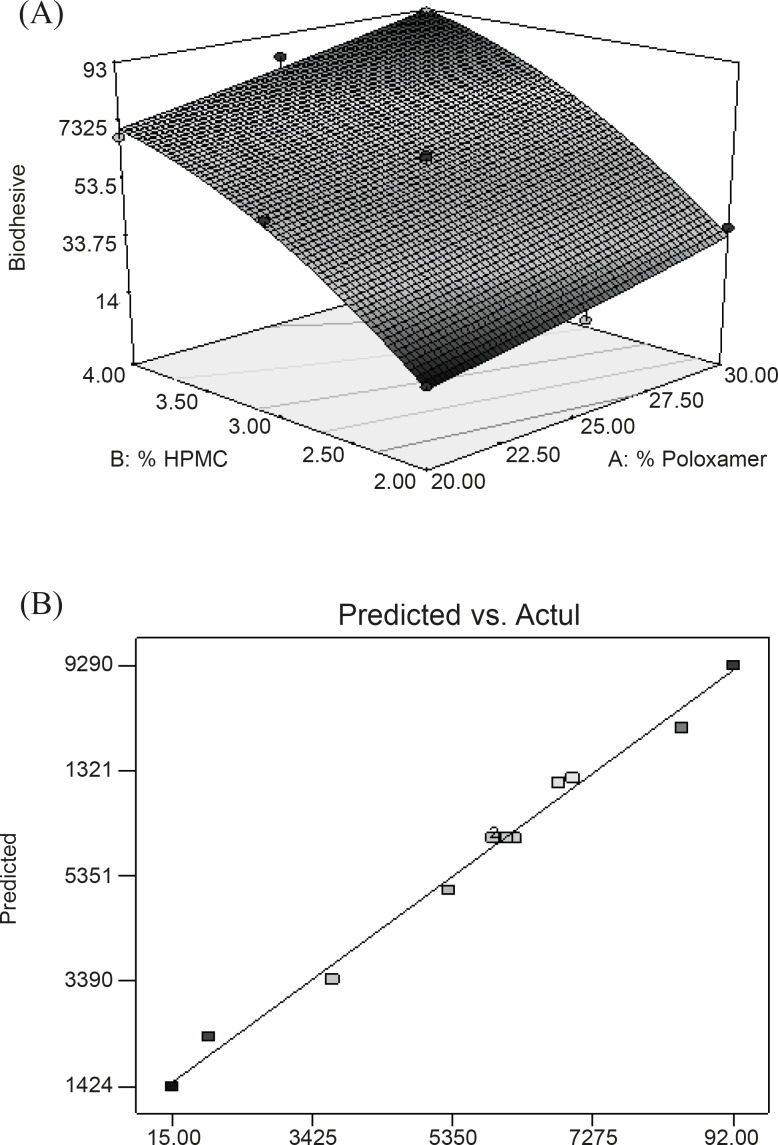
Effect of formulation variables on rheological properties (consistency index)
It is essential for any formulation to study its rheological behavior to be used for topical drug delivery applications. It is important for its efficacy in delivering molecules onto or across the skin. Rheological studies of all the gels were done to study the effect of polymer proportion on the viscosity. Consistency index and flow index were calculated for all the batches. Consistency index (CI) was a measure of consistency and equivalent to apparent viscosity at a shear rate of 1 sec-1. The flow index (FI) was a measure of the deviation of a system from Newtonian behavior (n = 1). Value of n < 1 indicates pseudoplastic flow or shear thinning system whereas n > 1 indicates dilatant flow or shear thickening system (28). All the gels showed a flow index of less than 1 (data not shown), indicating pseudoplastic flow behavior. Thus, gel becomes thin when applied on the skin and provide better spreadability. Values of consistency index (Y2) were summarized in Table 3. Mathematical modeling was applied to result obtained for the consistency index to evaluate the effect of independent variable (HPMC and Poloxamer content in gel) and interaction of two independent variables on rheological properties of gel. From the p-values presented in Table 4, linear contribution and quadratic contribution were found to be significant as p-value is less than 0.05 for both sources. To further fit the data to suitable model, the software was used to analyze the value of adjusted R2, predicted R2 and Predicted Residual Sum of Square (PRESS). Low PRESS value indicates adequate fitting of quadratic model (31). PRESS value for Y2 response (consistency index) showed no significant difference between the linear model and quadratic model (Table 5). The value of Adj. R2 of quadratic model was more than that of linear model (Table 5). Moreover, quadratic model was selected by software because of the highest order of polynomial model. Quadratic model was significant with model f-value of 133.53 (p-value < 0.0001). The quadratic equation generated by software is as follows:
Y2 = 10396.93 + 1664.6X1 + 7353.17X2 + 675.50X1X2 - 85.76X12 + 1432.74X22
In this case, X1, X2 and X22 are significant model terms. The equation represents the quantitative effect of factors (X1 and X2) upon the consistency index (Y2 response). Increased concentration of polymers resulted in a stronger gel structure as reflected by equation generated for quadratic model. Equation also reveals that HPMC has more pronounced effect than PL-407 on the consistency index of gel. Surface (Figure 2A) showed a steeper ascent in the response surface with HPMC (X2) than with Poloxamer (X1). It was in accord with equation generated by software showing pronounced effect of HPMC on consistency index. Model term X22 was also found to be significant (Table 6). This can be explained on the fact that HPMC swells in water and forms three disordered dimensional-physical networks. Tightly oriented gel structures are formed within poloxamer micelle pathways by HPMC-molecule entanglement and extensive hydrogen binding (32). Figure 2B represented the observed response value compared with that of predicted values indicating the correctness of model.
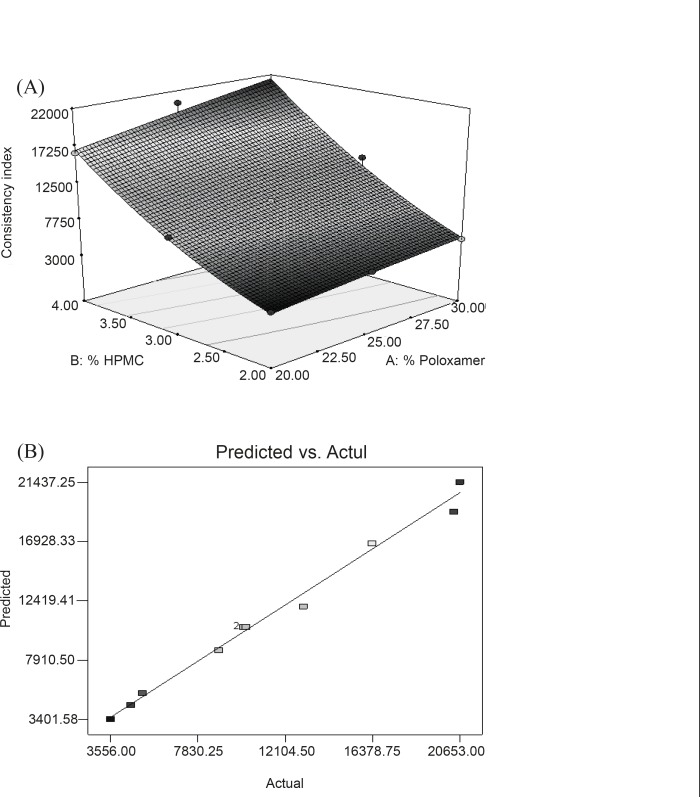
Analysis of variance (ANOVA) table for measured responses
Model/Model term | Y1 | Y2 | Y3 | Y4 | ||||
---|---|---|---|---|---|---|---|---|
f-value | p-value | f-value | p-value | f-value | p-value | f-value | p-value | |
Model | 142.32 | < 0.0001 | 133.53 | < 0.0001 | 164.78 | < 0.0001 | 317.22 | < 0.0001 |
X1 | 80.33 | < 0.0001 | 31.79 | 0.0008 | 412.77 | < 0.0001 | 458.46 | < 0.0001 |
X2 | 605.74 | < 0.0001 | 620.23 | < 0.0001 | 311.76 | < 0.0001 | 1082.71 | < 0.0001 |
X1X2 | 0.12 | 0.7377 | 3.49 | 0.1040 | 53.19 | 0.0002 | 8.47 | 0.0226 |
X12 | 0.17 | 0.6945 | 0.039 | 0.8494 | 0.75 | 0.4159 | 12.55 | 0.0094 |
X22 | 23.07 | 0.0020 | 10.84 | 0.0133 | 34.85 | 0.0006 | 34.43 | 0.0006 |
Effect of formulation variables on cumulative percentage release in 2 h
For this response, all the models (linear, 2FI and quadratic) are found to be significant with p-value < 0.05 (Table 4). Among all the models, lowest PRESS value was found for quadratic model. Therefore, quadratic model was selected to fit the data of this response (Table 5). Quadratic model was significant with model f-value of 164.78 (p-value < 0.0001).
The quadratic equation generated by software is as follows:
Y3 = 22.56 - 5.87X1 - 5.10X2 + 2.58X1X2 + 0.37 X12 + 2.51X22
In this case, X1, X2, X1X2 and X22 were found to be significant model terms (Table 6). Equation reveals that both factors have antagonistic effect on the drug release. Figure 3A displayed a non-linear relationship for CPR in 2 h at high levels of the polymers. This can be attributed to the occurrence of potential interaction between the two polymers at the corresponding factor levels, construing that each polymer tends to modify the effect of the other one toward the drug release. Model term X1X2 was found to be significant which indicating the potential effect of this interaction on the drug release in 2 h. Figure 3A also displayed the non-linearity of the response at high concentration of HPMC supported by the model term X22 which is found to be significant for the response. This could be due to the rigid gel structure formation due to the interaction between HPMC and PL-407 as already explained in consistency index. Figure 3B represented the observed response values compared with that of predicted values indicating the correctness of the model.
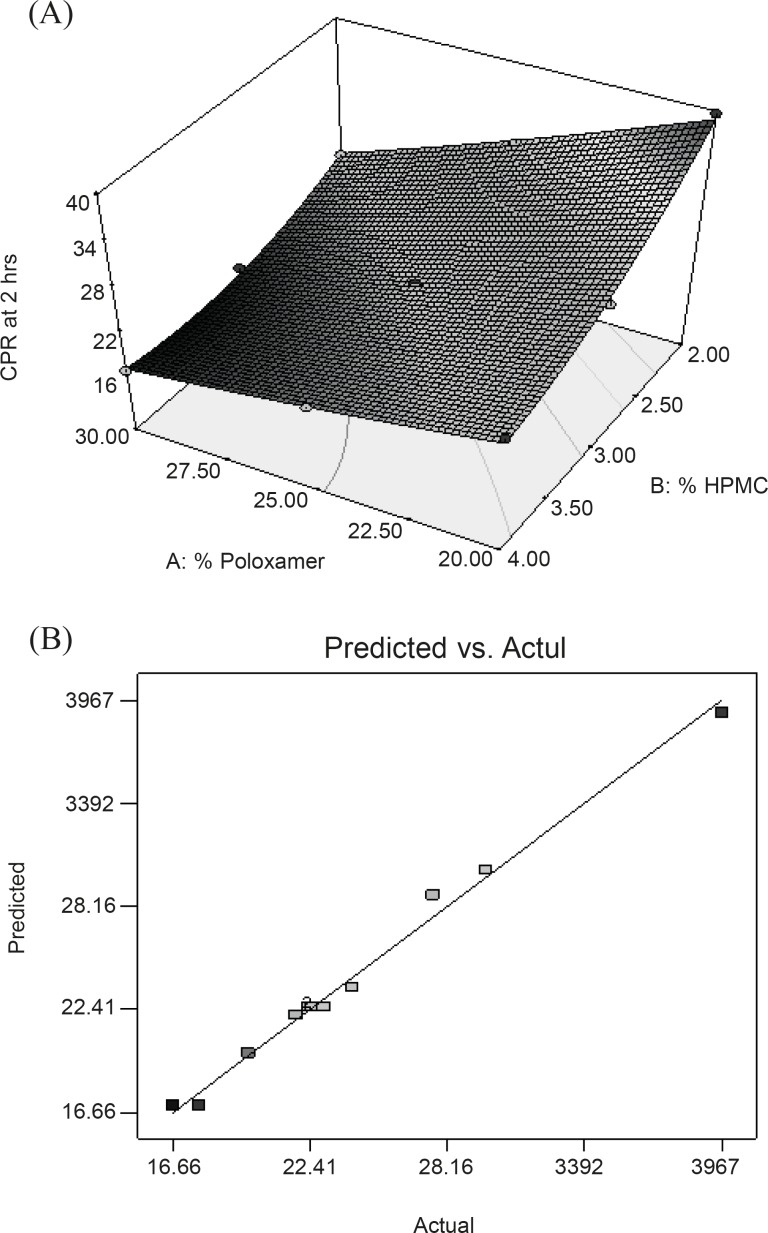
Effect of formulation variables on cumulative percentage release in 8 h
From the p-values presented in Table 4, linear contribution and quadratic contribution were found to be significant since p-value is less than 0.05 for both sources. In this case, A, B, AB, A2 and B2 are significant model terms. PRESS value for quadratic model (23.04) was found lower than that of linear model (41.21) as indicating in Table 5. Therefore, quadratic model was selected to fit the data of this response. Quadratic model was significant with model f-value of 317.22 (p-value < 0.0001). The quadratic equation generated by software is as follows:
Y4 = 50.64 - 5.47X1 - 8.40X2 + 0.91X1X2 - 1.33X12 + 2.21X22
In this case, all the model terms (X1, X2, X1X2, X12 and X22) were found to be significant (Table 6). The equation reveals that both factors have antagonistic effect on the drug release. CPR of the drug in 8 h with highest polymer content (30% Poloxamer and 4% HPMC) was found to be lowest. However, in this equation, it was clearly indicating that the retarding effect of HPMC was more prominent than PL-407. Coefficient of interactions shown in above equation was also significant which confirms the formation of rigid gel structure of PL-407 with HPMC. At high concentration of HPMC and PL-407, a very thick gel (highest consistency index value) was formed which provide a very slow release of drug. Figure 4A represented the response surface indicating the more pronounced effect of HPMC than PL-407 on Y4. Figure 4B represented the observed response value compared with that of predicted values indicating the correctness of model.
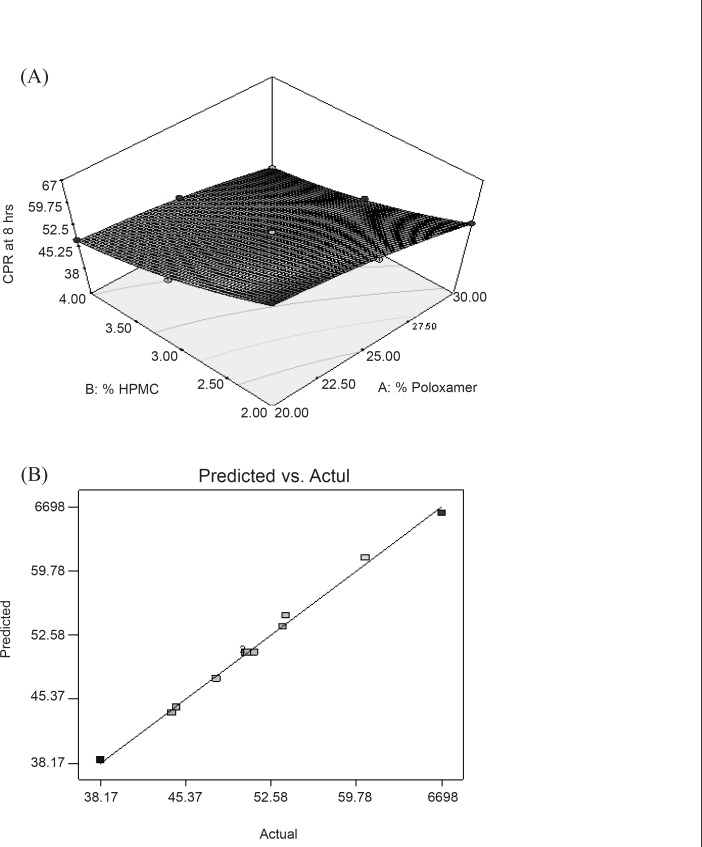
Conclusion
The present study concludes that topical bioadhesive gel of aceclofenac can be formulated by using combination of Poloxamer 407 and HPMC employing the response surface approach. The effect of formulation variables on the product characteristics can be easily predicted and precisely interpreted by using a 3-level factorial experimental design and generated quadratic mathematical equations. On the basis of product characteristics such as bioadhesive strength, consistency index and in-vitro release, it can be concluded that the best batch of topical bioadhesive gel of Aceclofenac would be with 20% PL-407 and 3% HPMC.
Acknowledgements
References
-
1.
Sarzi-Puttini P, Cimmino MA, Scarpa R, Caporali R, Parazzini F, Zaninelli A, Atzeni F, Canesi B. Osteoarthritis: an overview of the disease and its treatment strategies. Semin. Arthritis Rheum. 2005;35:1-10. [PubMed ID: 16084227].
-
2.
Gerwin N, Hops C, Lucke A. Intra-articular drug delivery in osteoarthritis Adv. Drug. Deliv. Rev. 2006;58:226-242.
-
3.
McKay N, Kelly CA. Extra-articular features of rheumatoid arthritis. Medicine. 2006;34:383-386.
-
4.
Zarrin Ghalam Moghaddam J, Naghade M. Comparison of the effect of Hirudo medicinals and Pyroxicam capsule on reduction of inflamation and pain in the primary stages of rheumatoid arthritis of knee. Iranian J. Pharm. Res. 2004;3:Suppel. 43-43.
-
5.
Yamazaki R, Kawai S, Matsuzaki T, Kaneda N, Hashimoto S, Yokokura T, Okamoto R, Koshino T, Mizushima Y. Aceclofenac blocks prostaglandin E2 production following its intra-cellular conversion into cyclooxygenase inhibitors. Eur. J. Pharmacol. 1997;329:181-187. [PubMed ID: 9226412].
-
6.
Lee J, Lee Y, Kim J, Yoon M, Choi YW. Formulation of microemulsion systems for transdermal delivery of aceclofenac. Arch. Pharm. Res. 2005;28:1097-1102. [PubMed ID: 16212244].
-
7.
Shaikh IM, Jadhav KR, Gide PS, Kadam VJ, Pisal SS. Topical delivery of Aceclofenac from lecithin organogels: preformulation study. Current Drug Delivery. 2006;3:417-427. [PubMed ID: 17076644].
-
8.
Shin SC, Cho CW, Choi HK. Structure and function of skin in dermatological and transdermal formulation. Drug Dev. Ind. Pharm. 1999;25:273-278. [PubMed ID: 10071819].
-
9.
Walters KA, Roberts MS. Structure and Function of Skin in Dermatological and Transdermal Formulation. NY: Marcel Dekker Inc; 2002. 119 p.
-
10.
Elias PM. Lipids and the epidermal permeability barrier. Arch. Dermatol. Res. 1981;270:95-117. [PubMed ID: 6167209].
-
11.
Naik A, Kalia YN, Guy RH. Transdermal drug delivery: overcoming the skin’s barrier function. Pharm. Sci. Technol. Today. 2000;3:318. [PubMed ID: 10996573].
-
12.
Cho CW, Choi JS, Shin SC. Development of the ambroxol gels for enhanced transdermal delivery. Drug Dev. Ind. Pharm. 2008;34:330-335. [PubMed ID: 18363149].
-
13.
Gendy AME, Jun HW, Kassem AA. In-vitro release studies of flurbiprofen from different topical formulations. Drug Dev. Ind. Pharm. 2002;28:823-831. [PubMed ID: 12236068].
-
14.
Rabinowitz JL, Feldman ES, Weinberger A, Schumacher RH. Comparative tissue absorption of oral 14C-Aspirin and topical Triethanolamine 14-Salicylate in human and canine knee joints. J. Clin. Pharmacol. 1982;22:42-48. [PubMed ID: 6977559].
-
15.
Shin SC, Cho CW, Yang KH. Development of lidocaine gels for enhanced local anesthetic action. Int. J. Pharm. 2004;287:73. [PubMed ID: 15541914].
-
16.
Shin SC, Lee JW, Yang KH, Lee CH. Preparation and evaluation of bioadhesive benzocaine gels for enhanced local anesthetic effects. Int. J. Pharm. 2003;260:77-81. [PubMed ID: 12818812].
-
17.
Csoka I, Csanyi E, Zapantis G, Nagy E, Feher KA, Horvath G, Blazs´o G, Eros I. In-vitro and in-vivo percutaneous absorption of topical dosage forms: case studies. Int. J. Pharm. 2005;291:1-19. [PubMed ID: 15707725].
-
18.
Shin SC, Kim HJ, Oh IJ, Cho CW, Ho YK. Development of tretinoin gels for enhanced transdermal delivery. Eur. J. Pharm. Biopharm. 2005;60:67-71. [PubMed ID: 15848058].
-
19.
HR Moghimi HR, Noorani N, Zarghi A. Stereoselective permeation of tretinoin and isotretinoin through enhancer-treated rat skin. II. effects of lipophilic penetration enhnacers. Iranian J. Pharm. Res. 2004;3:17-22.
-
20.
Shin SC, Cho CW. Enhanced transdermal delivery of pranoprofen from the bioadhesive gels. Arch. Pharm. Res. 2006;29:928-933. [PubMed ID: 17121190].
-
21.
Yong CS, Choi JS, Quan QZ, Rhee JD, Kim CK, Lim SJ, Kim KM, Choi HG. Effect of sodium chloride on the gelation temperature, gel strength and bioadhesive force of poloxamer gels containing diclofenac sodium. Int. J. Pharm. 2001;226:195-205. [PubMed ID: 11532582].
-
22.
Shin SC, Kim JY, Oh IJ. Mucoadhesive and physicochemical characterization of carbopol-poloxamer gels containing triamcinolone acetonide. Drug Dev. Ind. Pharm. 2000;26:307-312. [PubMed ID: 10738647].
-
23.
Yun MO, Choi HG, Jung JH, Kim CK. Development of a thermo-reversible insulin liquid suppository with bioavailability enhancement. Int. J. Pharm. 1999;189:137-145. [PubMed ID: 10536242].
-
24.
Schomlka IR. Artificial skin. I. Preparation and properties of pluronic F-127 gels of treatment of burns. J. Biomed. Mater. Res. 1972;6:571-582. [PubMed ID: 4642986].
-
25.
Varshosaz J, Tavakoli N, Saidan S. Development and characterization of a periodontal bioadhesive gel of metronidazole. Drug Delivery. 2002;9:127-133. [PubMed ID: 12055041].
-
26.
Singh S, Jain S, Muthu MS, Tiwari S, Tilak R. Preparation and evaluation of buccal bioadhesive films containing clotrimazole. AAPS PharmSciTech. 2008;9:660-7. [PubMed ID: 18500560].
-
27.
Rebelo ML, Pina ME. Release kinetics of tretinoin from dermatological formulations. Drug Dev. Ind. Pharm. 1997;23:727-730.
-
28.
Joshi M, Patravale V. Formulation and evaluation of nanostructured lipid carrier (NLC)- based gel of valdecoxib. Drug Dev. Ind. Pharm. 2006;32:911-918. [PubMed ID: 16954103].
-
29.
Bilensoy E, Rouf MA, Vural I, Sen M, Hincal AA. Mucoadhesive, thermosensitive, prolonged release vaginal gel for Clotrimazole: β-cyclodextrin complex. AAPS PharmSciTech. 2006;7:125.
-
30.
Verger ML, Fluckiger L, Kim Y. Preparation and characterization of nanoparticles containing an antihypertensive agent. Eur. J. Pharm. Biopharm. 1998;46:137-143. [PubMed ID: 9795032].
-
31.
Huang YB, Tsai YH, Yang WC, Chang JS, Wu PC. Optimization of sustained-release propranolol dosage form using factorial design and response surface methodology. Biol. Pharm. Bull. 2004;27:1626-1629. [PubMed ID: 15467208].
-
32.
Miller SC, Drabik BR. Rheological properties of poloxamer vehicles. Int. J. Pharm. 1984;18:269-276.