Abstract
Keywords
Introduction
Antimicrobial properties of silver nanoparticles have been broadly investigated by several researches throughout the last decade. The main difference among such studies is due to the various synthesis methods applied, yielding different shapes, sizes, optical properties and bactericidal effects (1-5, 11, 12). However, they all agree upon the fact that nanosilver is such a precious target at delivering alternative methods of disinfection as the issue of antibiotic resistance has been raised (4-6, 2, 10).
Escherichia coli (6, 8, 10), Staphylococcus aureus (3, 10), methicillin-resistant Staphylococcus aureus (11), Staphylococcus epidermis (4) and Bacillus subtilis (4) are among the targeted microorganisms used in the studies.
It has been concluded that nanomaterials could prove to be simple, cost effective and suitable for formulation of new type of bacterial materials (6, 7, 11). Therefore, it would be beneficial to set some specific standards through which bactericidal efficacy of silver nanoparticles made by various synthesis methods could be assessed.
Concerning the ever-growing application of nanosilver in various fields (13-14), we managed to access CEN TC 216 standards relating to the evaluation of chemical disinfectants and antiseptics used in food, industrial, domestic and institutional areas.
Experimental
Materials
Preparation of silver nanoparticles
The whole procedure of nanosilver synthesis included the two steps of seeding and growing. Seeding was done by electrochemical reduction of silver salt, followed by growing them in a different medium containing sodium citrate as a reducing agent and hydroxypropyl methylcellulose (HPMC) as a reducing and capping agent at 80°C (1, 2).
Deconex 53 instrument
Deconex 53 (Borer chemie) is an aldehyde-free, non-fixing disinfectant for manual disinfection and cleaning of surgical instruments, care utensils and dental instruments.
Typically, deconex53 instrument is being used for decontamination or pre-treatment of surgical and ward instruments. The product can also be used for final disinfection of non-critical medical devices. Active Ingredients of deconex 53 include Didecyl dimethyl ammonium chloride and N-(3-aminopropyl)-N-dodecylpropane-1, 3-diamine .
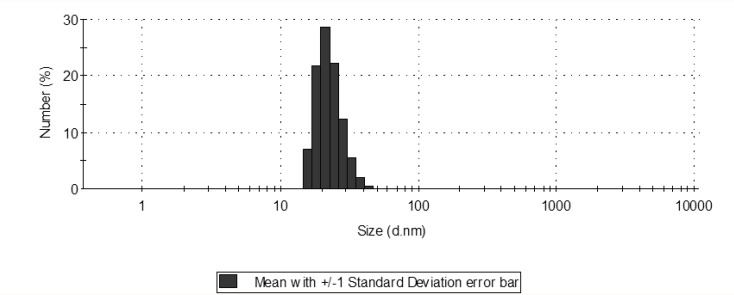
Methods
Characterization of silver nanoparticles
The synthesized nanoparticles were characterized by Ultraviolet-visible (UV-visible) spectroscopy (Shimadzu) and further examined by particle-size analyzer [Zetasizer (Nano-zs) by Malvern] to find out its size distribution.
Bacterial strains and preparation
Bacterial strains included Pseudomonas aeruginosa (ATCC 15442), Staphylococcus aureus (ATCC 6538) and Escherichia coli (ATCC 10536). The 24 h cultured colonies were used to yield 107 CFU/mL of each bacterial strain in normal saline. Plate counts of 10-4, 10-5 and 10-6 dilutions were done to confirm the initial bacterial load.
Bacterial susceptibility
The antibacterial potency of the synthesized silver nanoparticles was analyzed according to the CEN TC 216. Available standards were: (a) EN 1040:1997 (phase 1) which is a quantitative suspension test for the evaluation of basic bactericidal activity of chemical disinfectants and antiseptics; (b) EN 1276: 1997 (phase 2 step 1) which evaluates the bactericidal activity of chemical disinfectants and antiseptics used in food, industrial, domestic and institutional areas; (c) WI 216028: CEN Enquiry 1999 (phase 2 step 2) which evaluates bactericidal activity on surfaces of chemical disinfectants and antiseptics used in food, industrial, domestic and institutional areas (18).
Four dilutions of silver nanoparticles and Deconex 53 were made (geometric range two) through distilled water for phase 1 and hard water for phase 2. Standards indicate that dilutions should be prepared in such a way that include at least one in the active and one in the inactive range (two in the active range for phase 1). Silver nanoparticles were exposed to bacterial inoculums for 5, 15, 30 and 60 min at 20°C in phase 1 and 5 min for phase 2. In phase 2, the influence of interfering substances including bovine serum albumin (0.3% for 1st step and 3% for the 2nd step), skimmed milk (Himedia) and yeast extract (Fluka) in the efficacy of the disinfectants, was assessed (dirty condition). Survivor count was done by inoculation in the tumor-specific antigen (TSA) culture and incubation at 37°C for 48 h.
Results and Discussion
UV-visible spectroscopy revealed surface plasmon absorption with two peaks at 322 and 450 nm. According to the Mie theory (19), only a single Surface plasmon resonance (SPR) band is expected in the absorption spectra of spherical nanoparticles, whereas, anisotropic particles could give rise to two or more SPR bands depending on the shape of the particles. The number of SPR peaks increases as the symmetry of the nanoparticle decreases.
Size distribution showed a range of 15.69 to 50.75 with a mean of 22.8 nm. Of course, smaller nanoparticles show more antibacterial properties due to the appropriate penetration through microorganisms’ membrane (2).
Phase 1
Figures 2 and 3 display the antibacterial potency of four dilutions of synthesized nanosilver against Staphylococcus aureus and Pseudomonas aeruginosa in phase 1. The first three dilutions showed a significant bactericidal effect for both of the tested strains (6 to 7 log-reduction of the initial inoculums), while the fourth dilution only reduced the initial bacterial load by 3 to 4 log-reduction. According to the EN 1040 (phase 1) standard, 5-log reduction in viability within 60 min or less with both microorganisms, is essential. So this formulation could successfully pass this phase.
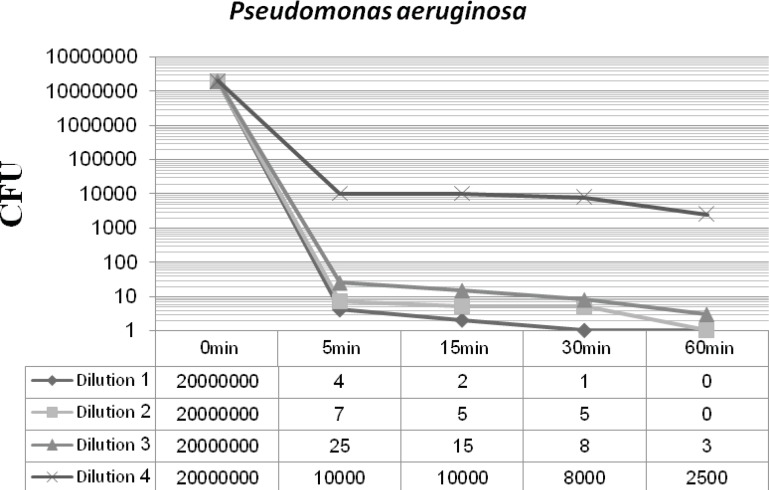
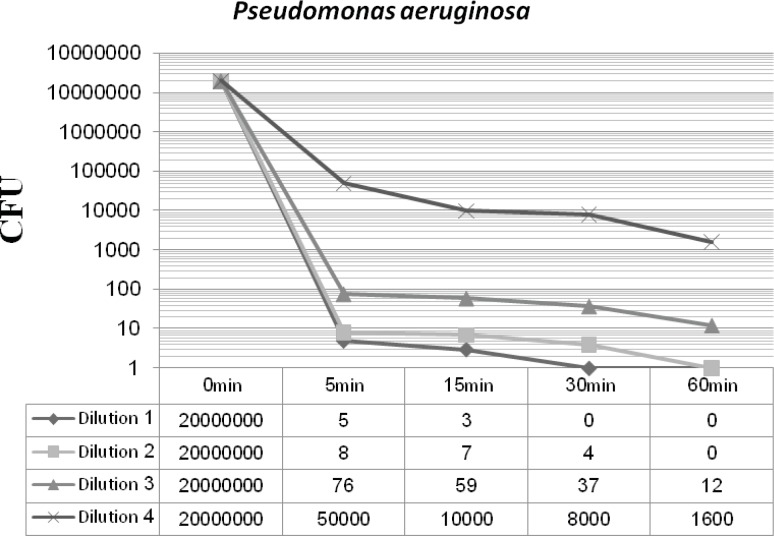
Deconex 53 Instrument showed a significant bactericidal effect by inhibiting the growth of both strains in all four dilutions (0.25, 0.5, 1 and 2%) at the tested times.
Phase 2, step 1
In this phase, two different conditions were considered dirty and clean. It is due to simulating the tested conditions to industrial, domestic and institutional areas. Materials such as bovine serum albumin (BSA), yeast extract and skimmed milk were used for this purpose. In addition to Staphylococcus aureus and Pseudomonas aeruginosa, Escherichia coli was introduced in this phase. The 1st dilution of nanosilver passes the 1st step of 2nd phase with the minimum of 5-log reduction of Staphylococcus aureus and maximum of 7-log reduction of Escherichia coli. On the other hand, the 2nd dilution was in the inactive range (Figures 4 and 5).
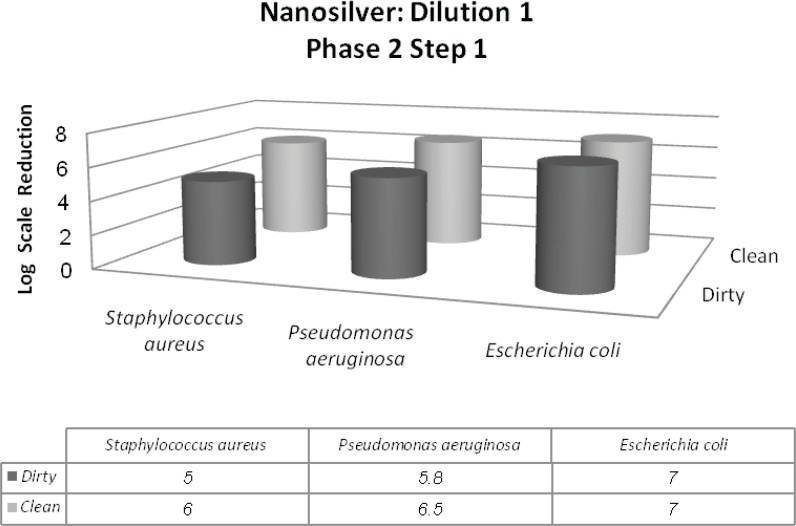
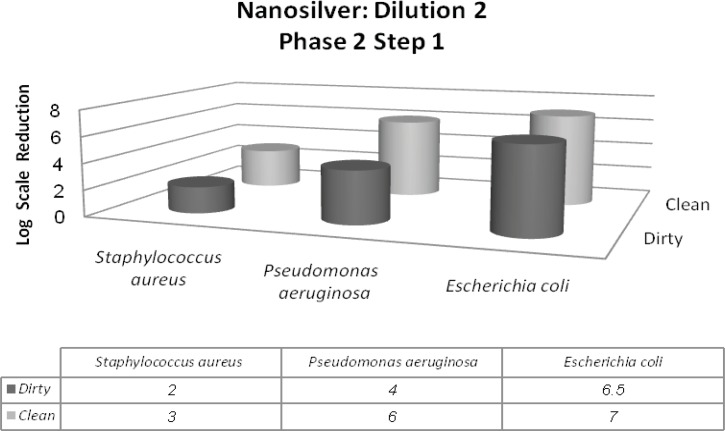
Deconex 53 was diluted to reach the inactive range. Figure 6 shows the 0.1, 0.05 and 0.025% dilutions and their effectiveness in the dirty condition, by which 0.25% dilution was in the inactive range and 0.1 and 0.05% dilutions passed the 1st step of 2nd phase by minimum reduction of Staphylococcus aureus with 5 units in logarithmic scale.
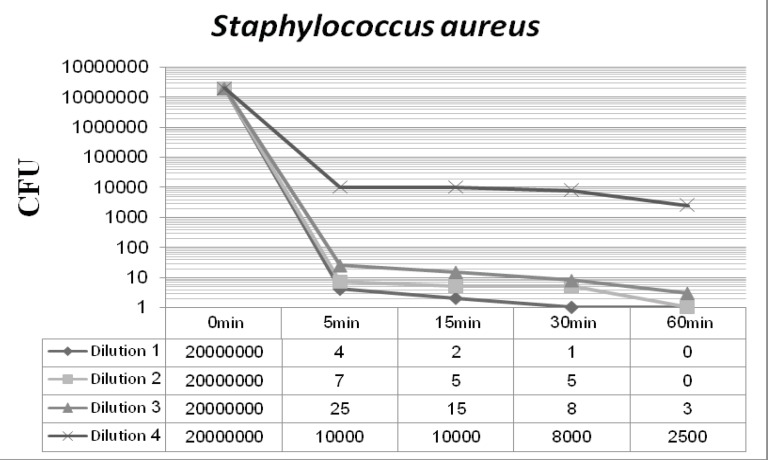
Phase 2, step 2
In spite of previous steps that 107 inoculums were used for all strains, in this step, we should have used 108 inoculums. Two different conditions were also prepared in this step. A 4-log reduction in viability is necessary to pass this step. Only the first dilution of nanosilver passed the 2nd step of phase 2, by displaying a minimum 4-log reduction for Staphylococcus aureus (Figure 7-C) yet inhibiting the growth of Escherichia coli in both clean and dirty conditions (Figure 7-B). It could be understood that interfering agents could not affect Escherichia coli susceptibility because of its high sensitivity to silver nanoparticles. Unfortunately, second dilution caused less than 4-log reduction in viability of Staphylococcus aureus. Hence, second dilution could not pass this step.
From 0.1 and 0.05% dilutions of Deconex 53 that passed the step 1, only 0.1% dilution could pass the 2nd step as well by reducing the initial inoculum by the minimum of 4-log scale .
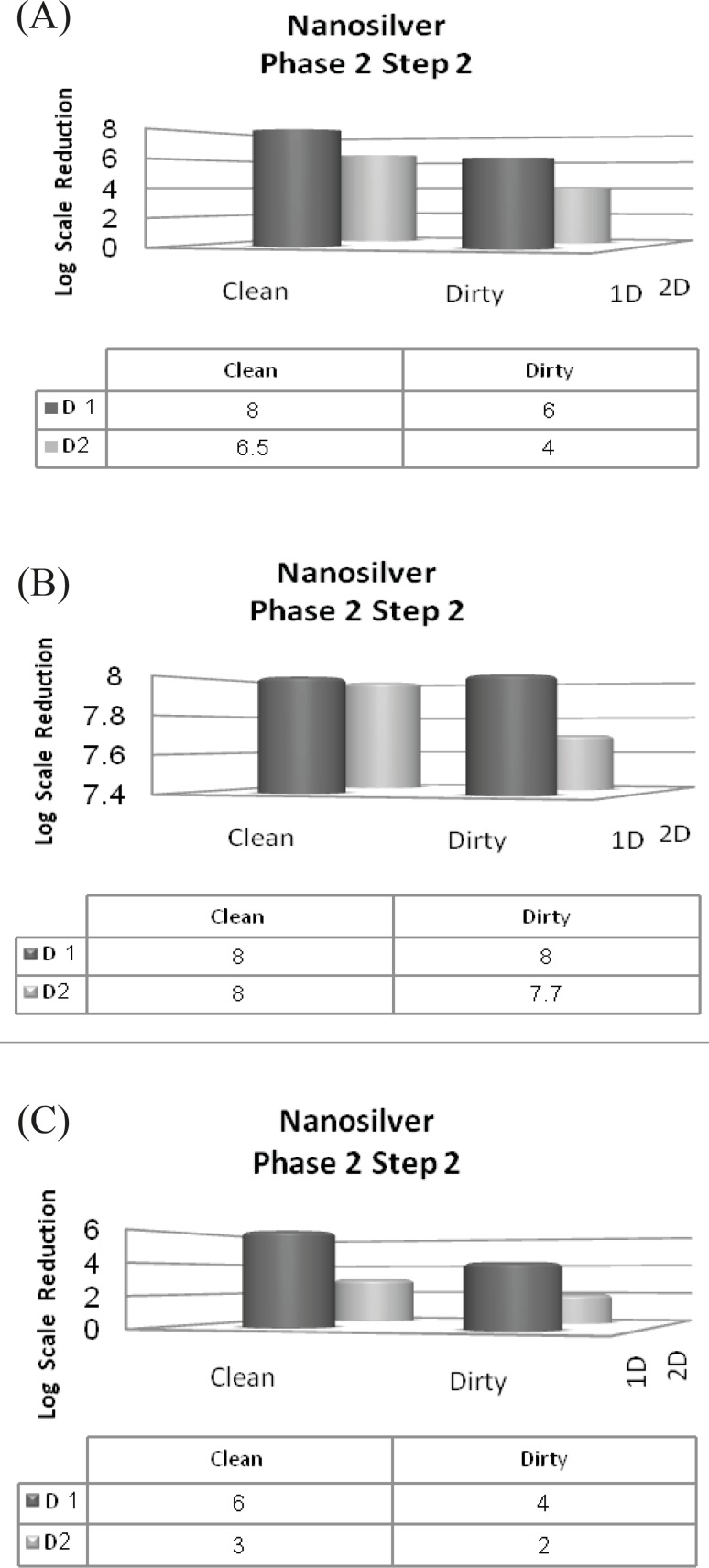
Conclusion
It was observed that among the three standard tested organisms, Escherichia coli showed the highest sensitivity to silver nanoparticles, which supports the results of another study in this topic (6). On the other hand, Staphylococcus aureus was the most stubborn among the three strains. The presence of interfering substances such as albumin was important in the efficacy of the bactericidal properties of nanosilver; it could be said that the more the concentration of interfering substances, the higher the concentration of the nanosilver should be to provide the same bactericidal effect.
Silver nanoparticles produced in this study, proved to have a prominent bactericidal activity and compared to Deconex 53, as a strong chemical disinfectant, are competitive disinfectants.
Further standards could be applied to qualify nanosilver for specific uses in other areas such as medical and veterinary. Moreover, fungicidal and sporicidal activity of silver nanoparticles could be analyzed as well.
Acknowledgements
References
-
1.
Wiley B, Sun Y, Mayers B, Xia Y. Shape-controlled synthesis of metal nanostructures: the case of silver. Chemistry. 2005;11:454-63. [PubMed ID: 15565727].
-
2.
Pal S, Tak YK, Song M. Does the antibacterial activity of silver nanoparticles depend on the shape of the nanoparticle? A study of the gram-negative bacterium Escherichia coli. J. App. Env. Mic. 2007;73:1712.
-
3.
Sosa I O, Noguez C, Barrera RG. Optical properties of metal nanoparticles with arbitrary shapes. J. Phys. Chem. B. 2003;107:6269–6275.
-
4.
Duran N, Marcarto PD, De Souza GIH, Alves OL, Esposito E. Antibacterial effect of silver nanoparticles produced by fungal process on textile fabrics and their effluent treatment. J. Biomed. Nanotechnol. 2007;3:203-8.
-
5.
Gong P, Li H, He X, Wang K, Hu J, Tan W, Zhang S, Yang X. Preparation and antibacterial activity of Fe3O4@Ag nanoparticles. Nanotechnology. 2007;18:604-11.
-
6.
Shrivastava S, Bera T, Roy A, Singh G, Ramachandrarao P, Dash D. Characterization of enhanced antibacterial effects of novel silver nanoparticles. Nanotechnology. 2007;18.
-
7.
Kim JS, Kuk E, Yu KN, Kim JH, Park SJ, Lee HJ, Kim SH, Park YK, Park YH, Hwang CY, Kim YK, Lee YS, Jeong DH, Cho MH. Antimicrobial effects of silver nanoparticles. Nanomed. Nanotechnol. Biol. Med. 2007;3:95-101.
-
8.
Sondi I, Salopek-Sondi B. Silver nanoparticles as antimicrobial agent: a case study on Escherichia coli as a model for gram-negative bacteria. J. Colloid Interface Sci. 2004;275:177. [PubMed ID: 15158396].
-
9.
Yamanaka M, Hara K, Kudo J. Bactericidal actions of a silver ion solution on Escherichia coli, studied by energy-filtering transmission electron microscopy and proteomic analysis. Appl. Env. Microbiol. 2005;71:7589-93. [PubMed ID: 16269810].
-
10.
Shahverdi AR, Fakhimi A, Shahverdi HR, Minaian S. Synthesis and effect of silver nanoparticles on the antibacterial activity of different antibiotics against Staphylococcus aureus and Escherichia coli. Nanomed. Nanotechnol. Biol. Med. 2007;3:168-71.
-
11.
Panacek A, Kvitek L, Prucek R, Kolar M, Vecerova R, Pizurova N, Sharma VK, Nevecna T, Zboril R. Silver colloid nanoparticles: synthesis, characterization and their antibacterial activity. J. Phys. Chem. B (2006;110:16248-53.
-
12.
Orafai H, Kallinteri P, Garnett M, Huggins S, Hutcheon G, Pourcain C. Novel poly (glycerol-adipate) polymers used for nanoparticle making: a study of surface free energy. Iranian J. Pharm. Res. 2008;7:11-19.
-
13.
Cheng D, Yang J, Zhao Y. Antibacterial materials of silver nanoparticles application in medical appliances and appliances for daily use. Chin. Med. Equip. J. 2004;4:26-32.
-
14.
Lee KS, El-Sayed MA. Gold and silver nanoparticles in sensing and imaging: sensitivity of Plasmon response to size, shape, and metal composition. J. Phys. Chem. 2006;110:19220-25.
-
15.
Lansdown AB. Silver in health care: antimicrobial effects and safety in use. Curr. Probl. Dermatol. 2006;33:17-34. [PubMed ID: 16766878].
-
16.
Fazeli MR, Hosseini V, Shamsa F, Jamalifar H. Preparation and in-vitro antibacterial evaluation of electroless silver coated polymers. Iranian J. Pharm. Res. 2010;9:259-264.
-
17.
Dabbagh MA, Moghimipour E, Ameri A, Sayfoddin N. Physicochemical characterization and antimicrobial activity of nanosilver containing hydrogels. Iranian J. Pharm. Res. 2008;7:21-28.
-
18.
Cremieux A, Freney J, Davin-Regli A. Methods of testing disinfectants. In: Block SS, editor. Disinfection, Sterilization and Preservation. 5th ed. Philadelphia: Lippincott Williams and Wilkins; 2001. p. 1305-25.
-
19.
Mie G. Contributions to the optics of turbid media, especially colloidal metal solutions. Ann. Phys. 1908;25:377-445.