Abstract
Keywords
Drug efflux transporters Mrp2 Drug disposition Acetaminophen Inflammation
Introduction
Multidrug resistant protein 2 (MRP2, ABCC2) is a membrane efflux transporter widely distributed in normal (hepatic canalicular membrane, lung, kidney blood brain barrier and intestinal epithelium) and tumor tissues (1). It protects cells and tissues by transporting various drugs including cytotoxic anticancer agents and endogenous compounds outside the cell. This protein is thought to be potentially important in the disposition of many drugs (e.g. acetaminophen and methotrexate) and their substrates (2-4). Therefore, it is important physiologically and pharmacologically to evaluate the expression patterns of this protein in normal and disease state and the resulting changes in the therapeutic concentration of the medicinal agents are transported through this efflux protein. The present study investigated the pharmacological effects of the inflammatory state on the disposition of acetaminophen (APAP) as substrate of MRP2 in rat as a rodent model.
Experimental
Animals and treatment
Sprague Dawley rats (180-250 g) were obtained from Razi Institute (Mashhad, Iran) and housed in groups of five under standard laboratory conditions of constant temperature (21 ± 2°C) and a 12/12 h (light/dark) cycle for at least 10 days prior to testing. Commercial food pellets and tap water was freely available. Animals were transferred to the testing laboratory at least one day before the beginning of experiments. The use of animals was carried out in accordance with the regulations of the Mashhad University of Medical Sciences (MUMS) Ethics Committee. Polyethylene (PE-50, Clay Adams) cannulas tipped with 2.5 cm of silastic tubing (Dow Corning, Midland, USA) were inserted into the left jugular vein and exteriorized by subcutaneous tunneling in the interscapular area. Rats were injected intraperitoneally with endotoxin (LPS, Sigma-Aldrich) at 5 mg/Kg. Control rats received the equivalent volume of sterile saline buffer.
In-vivo pharmacokinetic studies
Acetaminophen, APAP (sigma) was dissolved in ethanol: PEG 600 : water (5 : 1 : 4) to give a concentration of 50 mg/mL and administered into the jugular vein of cannulated rats at dose of 50 mg/Kg. At various time points following drug administration (15, 30, 60, 120, 180, 240 and 360), 250 μL of blood samples were taken into the heparinized syringes from the cannula and the same volume of sterile saline or saline/heparin was injected through cannula. Blood samples were centrifuged for 5 min at 6000 rpm to obtain plasma. All samples were frozen at -80°C until analysis.
HPLC assay
The analysis of APAP from plasma was conducted according to the method of de Moraes et al. (5). Briefly, the HPLC system consisted of Waters 600E system controller and pump and a Waters 486 Tunable absorbance detector. APAP was separated on a NovaPack C18 column 3.9 x 150 mm. An isocratic procedure was used with the mobile phase consisting of 85% acetic acid, 0.2 N (pH 2.7), 15% methanol and internal standard acetanilide (BDH). A flow rate of 1.3 mL/min was used. Spectrophotometric detection was achieved at a 244 nm wavelength. Peaks were recorded and integrated using Waters Empower software. Calibration curves were obtained through linear regression analysis of the concentration versus the ratio of peak area of APAP and the internal standard (acetanilide). The typical retention time recorded for APAP was 4.9. The AUC for APAP from 0 to last data point was calculated through a trapezoidal rule. The elimination rate was determined from the slope of the line for plasma concentration vs. time.
Analysis of Mrp2 gene expression
Total RNA from two combined liver slices was isolated using High Pure RNA isolation Kit (Roche Applied Science) and cDNA was synthesized from total RNA (5 mg) using the First Strand cDNA Synthesis Kit (Fermentas), according to the manufacturer’s protocol.
The PCR standard curves for each gene product (β-actin and MRP2) were generated from serial dilutions of RT product and the optimal amounts of template were determined from the linear portions of the resulting PCR calibration curves. PCR was performed using 50 pmol of sense and antisense primers (MRP2 forward:
5’-ACCTTCCACGTAGTGATCCT-3’; MRP2 reverse:
5’-ACCTGCTAAGATGGACGGTC-3’; β-actin forward:
5’-GCCCAGAGCAAGAGAGGTAT-3’ and β-actin reverse:
5’-GGCCATCTCTTGCTCGAAGT-3’), 1-2.5 mL of RT product and 2.5 units of Taq DNA polymerase (Cinnagen, Tehran, Iran). PCR products were run on a 2% agarose gel, stained with SYBR Gold Stain (Molecular Probes). Optical intensities of the PCR products were normalized to mRNA levels of β-actin.
Results and Discussion
Hepatic expression of MRP2 in endotoxemic rats
To establish the effects of inflammation (induced by bacterial lipopolysaccharide) on the hepatic expression of MRP2 gene, amplification of cDNA was assessed. Administration of LPS resulted in a slight but not significant increase of MRP2 mRNA in the rat livers 24 h after the treatment (Figure 1).
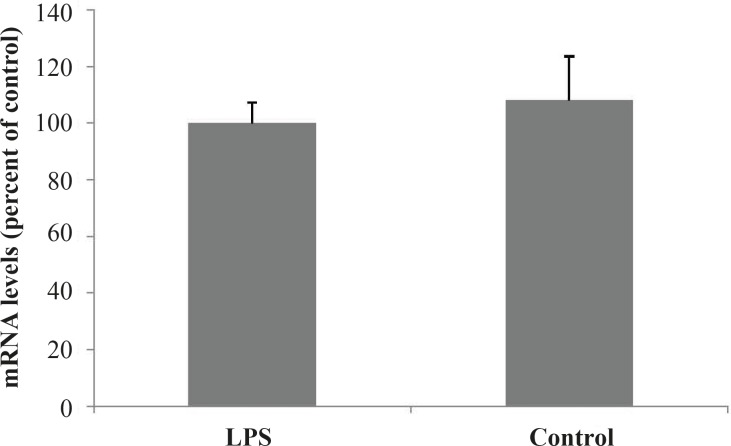
PCR products were separated on a 2% agarose gel, stained with SYBR Gold Stain (Molecular Probes). Optical intensities of the PCR products were normalized to mRNA levels of β-actin.
Functional transport activity measured by plasma concentration of APAP
Compared to controls, in LPS-treated rats at time points up to 60 min, a rapid decrease in plasma concentrations of the MRP2 substrate (APAP) was observed. At later time points, the slope of the substrate concentration reached a plateau, equaled to the controls and remained constant (Figure 2). This sharp drop in plasma concentration would indicate an increase in the excretion of substrate through different excretion mechanisms including higher efflux activity of the MRP2 drug transporter.
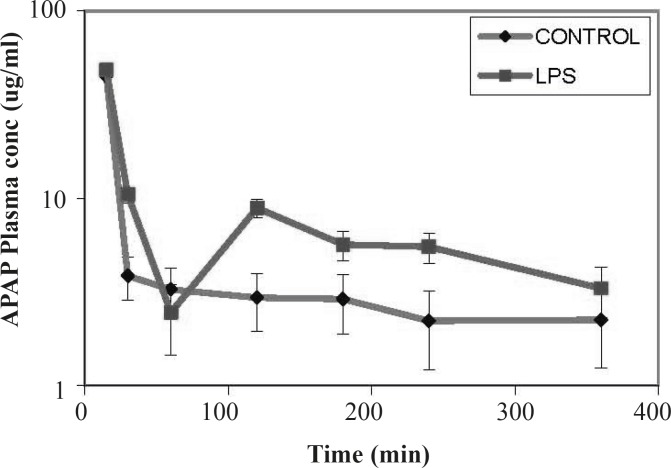
The disposition and clearance of drug substrates are highly regulated by membrane multi drug transport proteins and other mechanisms including cytochromes P450. The drug transporter protein MRP2 was initially discovered in tumors. It was also found to be present in normal tissues including hepatic canalicular membranes, kidney, lung and the blood brain barrier and is involved in the disposition of many drugs (1).
Acetaminophen (APAP) is passively diffused into the hepatocytes and conjugated with glucoronic acid and sulfate (6-8). In-vivo and in-vitro studies indicate that MRP2 is involved in the transport of APAP and its metabolites (9-11).
It has been demonstrated by many studies that systemic inflammation, proinflammatory cytokines and anti-inflammatory drugs would potentially alter drug transporters regulation (12-14). It has been shown that LPS treatment reduces the expression of MRP2 transporter in rat liver (15). However, many reports indicate that in LPS-treated animals compensatory increases of other transporters including MRP1 and MRP3 have been observed (16).
In this study, we evaluated the effect of inflammation induced by LPS on expression and activity of the canalicular MRP2 in rat as a rodent model. Our data indicated that the expression of MRP2 was slightly – but not significantly – decreased after exposing the rat to the LPS. The transport function of the transporter was also studied using APAP as a substrate of the drug efflux pump. It was shown that in LPS-treated rats initially a rapid decrease in plasma concentrations of the MRP2 substrate (APAP) was observed. However, the slope of the substrate concentration eventually reached a plateau, equalized to the controls and remained constant. In this study, the observed down regulation of the MRP2 pump is in accordance to previous reports of other groups indicating down regualtion of the this transporter after treatment by LPS or pro-inflammatory cytokines (15, 17, 18). Endotoxin-dependent decrease in MRP2 expression in the rat liver did not result in substantial changes in the plasma concentrations of APAP compared to control. Although MRP2 is considered an important transporter in APAP and APAP metabolites excretion, other transporters including MRP3 drug transporter have also been implicated in the elimination of these chemicals in rodents (19-21). According to the results, despite the slight down regulation of the MRP2 transporter, the plasma concentration of APAP in LPS treated rats was not lower than that of controls. This observation could be due to the compensatory increases in other transporters such as MRP3-induced by systematic inflammation (22).
In summary, we demonstrated slightly decreased expression of the drug transporter MRP2 in rats treated with LPS. The activity of the pump as judged by the time course changes in the plasma concentration of the APAP was also altered. However, due to the presence of the possible compensatory mechanisms of drug transport in liver and non-hepatic tissues, similar changes to the transporter activity were not observed. Nevertheless, the obtained data indicates that it is crucial to consider the alternate transport mechanisms in predicting plasma concentration in animal studies and therapeutic response of the patients in diseased state. Furthermore, our results highlight the necessity and importance of tissue specific and compensatory transport mechanism to be able to have a more understanding of the patient therapy during the inflammation.
Acknowledgements
References
-
1.
Fardel O, Jigorel E, Le Vee M, Payen L. Physiological, pharmacological and clinical features of the multidrug resistance protein 2. Biomed. Pharmacother. 2005;59:104-14. [PubMed ID: 15795103].
-
2.
Vlaming ML, Pala Z, van Esch A, Wagenaar E, van Tellingen O, de Waart DR. Impact of Abcc2 (Mrp2) and Abcc3 (Mrp3) on the in-vivo elimination of methotrexate and its main toxic metabolite 7-hydroxymethotrexate. Clin. Cancer Res. 2008;14:8152-60. [PubMed ID: 19088030].
-
3.
Vlaming ML, Pala Z, van Esch A, Wagenaar E, de Waart DR, van de Wetering K. Functionally overlapping roles of Abcg2 (Bcrp1) and Abcc2 (Mrp2) in the elimination of methotrexate and its main toxic metabolite 7-hydroxymethotrexate in-vivo. Clin. Cancer Res. 2009;15:3084-93. [PubMed ID: 19383815].
-
4.
Zamek-Gliszczynski MJ, Hoffmaster KA, Tian X, Zhao R, Polli JW, Humphreys JE. Multiple mechanisms are involved in the biliary excretion of acetaminophen sulfate in the rat: role of Mrp2 and Bcrp1. Drug Metab. Dispos. 2005;33:1158-65. [PubMed ID: 15860656].
-
5.
De Morais SM, Wells PG. Deficiency in bilirubin UDP-glucuronyl transferase as a genetic determinant of acetaminophen toxicity. J. Pharmacol. Exp. Ther. 1988;247:323-31. [PubMed ID: 3139868].
-
6.
Grafstrom R, Ormstad K, Moldeus P, Orrenius S. Paracetamol metabolism in the isolated perfused rat liver with further metabolism of a biliary paracetamol conjugate by the small intestine. Biochem. Pharmacol. 1979;28:3573-9. [PubMed ID: 533560].
-
7.
Moldeus P. Paracetamol metabolism and toxicity in isolated hepatocytes from rat and mouse. Biochem. Pharmacol. 1978;27:2859-63. [PubMed ID: 736978].
-
8.
Talegaonkar S, Yakoob Khan A, Kishan Khar R, Jalees Ahmad, Z Khan. Development and characterization of paracetamol complexes with hydroxypropyl-β-cyclodextrin. Iranian J. Pharm. Res. 2007;6:95-99.
-
9.
Xiong H, Turner KC, Ward ES, Jansen PL, Brouwer KL. Altered hepatobiliary disposition of acetaminophen glucuronide in isolated perfused livers from multidrug resistance-associated protein 2-deficient TR (-) rats. J. Pharmacol. Exp. Ther. 2000;295:512-8. [PubMed ID: 11046083].
-
10.
Silva VM, Thibodeau MS, Chen C, Manautou JE. Transport deficient (TR-) hyperbilirubinemic rats are resistant to acetaminophen hepatotoxicity. Biochem. Pharmacol. 2005;70:1832-9. [PubMed ID: 16271353].
-
11.
Ghanem CI, Gomez PC, Arana MC, Perassolo M, Ruiz ML, Villanueva SS. Effect of acetaminophen on expression and activity of rat liver multidrug resistance-associated protein 2 and P-glycoprotein. Biochem. Pharmacol. 2004;68:791-8. [PubMed ID: 15276087].
-
12.
Elahian F, Kalalinia F, Behravan J. Dexamethasone downregulates BCRP mRNA and protein expression in breast cancer cell lines. Oncol. Res. 2009;18:9-15. [PubMed ID: 19911699].
-
13.
Mosaffa F, Lage H, Afshari JT, Behravan J. Interleukin-1 beta and tumor necrosis factor-alpha increase ABCG2 expression in MCF-7 breast carcinoma cell line and its mitoxantrone-resistant derivative, MCF-7/MX. Inflamm. Res. 2009;58:669-76. [PubMed ID: 19333723].
-
14.
Morgan ET, Goralski KB, Piquette-Miller M, Renton KW, Robertson GR, Chaluvadi MR. Regulation of drug-metabolizing enzymes and transporters in infection, inflammation, and cancer. Drug Metab. Dispos. 2008;36:205-16. [PubMed ID: 18218849].
-
15.
Elferink MG, Olinga P, Draaisma AL, Merema MT, Faber KN, Slooff MJ. LPS-induced downregulation of MRP2 and BSEP in human liver is due to a posttranscriptional process. Am. J. Physiol. Gastrointest. Liver Physiol. 2004;287:G1008-16. [PubMed ID: 15205115].
-
16.
Petrovic V, Teng S, Piquette-Miller M. Regulation of drug transporters during infection and inflammation. Mol. Interv. 2007;7:99-111. [PubMed ID: 17468390].
-
17.
Siewert E, Dietrich CG, Lammert F, Heinrich PC, Matern S, Gartung C. Interleukin-6 regulates hepatic transporters during acute-phase response. Biochem. Biophys. Res. Commun. 2004;322:232-8. [PubMed ID: 15313196].
-
18.
Hartmann G, Cheung AK, Piquette-Miller M. Inflammatory cytokines, but not bile acids, regulate expression of murine hepatic anion transporters in endotoxemia. J. Pharmacol. Exp. Ther. 2002;303:273-81. [PubMed ID: 12235261].
-
19.
Belinsky MG, Dawson PA, Shchaveleva I, Bain LJ, Wang R, Ling V. Analysis of the in-vivo functions of Mrp3. Mol. Pharmacol. 2005;68:160-8. [PubMed ID: 15814571].
-
20.
Manautou JE, de Waart DR, Kunne C, Zelcer N, Goedken M, Borst P. Altered disposition of acetaminophen in mice with a disruption of the Mrp3 gene. Hepatology. 2005;42:1091-8. [PubMed ID: 16250050].
-
21.
Zelcer N, van de Wetering K, de Waart R, Scheffer GL, Marschall HU, Wielinga PR. Mice lacking Mrp3 (Abcc3) have normal bile salt transport, but altered hepatic transport of endogenous glucuronides. J. Hepatol. 2006;44:768-75. [PubMed ID: 16225954].
-
22.
Lee G, Piquette-Miller M. Influence of IL-6 on MDR and MRP-mediated multidrug resistance in human hepatoma cells. Can. J. Physiol. Pharmacol. 2001;79:876-84. [PubMed ID: 11697747].