Abstract
Keywords
Micromass culture Enamel matrix derivative Neural crest Teratogen
Introduction
The developing central nervous system is much more susceptible to adverse effects of toxic agents than adults’ CNS system. In fact, among congenital defects, the brain has been introduced as the major target organ of toxicity (1, 2). Moreover, CNS effects are seen most often at lower doses than those required to affect other parts of the body (3-5). Since the use of Enamel matrix derivative (EMD) is being increased every year, there is a great concern about its teratogenic effects in case it is used during the first semester of pregnancy. EMD is a commercially available product isolated from the crowns of six month old pigs. It has been proven to be clinically useful in improving periodontal healing of avulsed and replanted teeth, as it was reported to be effective in treatment of periodontal intra-bony effects (6, 7). Emdogain is a commercial EMD and contains several matrix proteins from the amelogenin family. Several studies have shown that EMD influences.
the migration, attachment, proliferative capacity and biosynthetic activity of periodontal ligament cells (8-11). Furthermore, it is considered to be effective in enhancing the healing process of replanted teeth and has been recommended as therapeutic agent for the management of avulsed permanent teeth (12-14).
During the critical period of organogenesis, the embryo is especially sensitive to toxic insults of certain drugs and other xenobiotics. The mammalian embryo itself (particularly the rat embryo) can be cultured for a limited period of time to be used as an in-vitro teratogenicity assay. Midbrain cells, when grown in high-density cultures, can be differentiated to neurons (15-18).
Rat embryo midbrain cells can be differentiate under particular conditions of culture and produce high density forming neurons (19, 20). Cultures of neural tissue for pharmacological or electrophysiological investigations are normally prepared by dissociating differentiated neurons and glial cells from fetal rat brain (21, 22). One way to optimize the number of high-density cultures obtained from a small quantity of embryonic tissue is using the micromass technique developed by Ahrens, Solursh and Reiter (23). A modification for use with embryonic neural tissue has been described in this paper. Another method which is widely used in teratology is limb bud micromass culture. Limb bud mesenchymal cells, when grown in a micromass culture system, can be differentiated into several of cell types such as cartilage and muscle. Any alteration in expression of the markers which are related to chondrogenesis can be considered as teratogenic characteristics of a xenibiotic. One advantage of this technique is that a homogeneous set of cultures is produced in which differentiation may be followed quantitatively by biochemical or morphometric analysis (24, 25).
Experimental
Chemicals
Mayer’s hematoxylin and neutral red were purchased from Sigma chemicals Co. (St. Louis, Mo, USA). Phosphate buffered saline (PBS), Trypsin, Fetal bovine serum (FBS) and RPMI 1640 medium with L-Glutamine were purchased from Life sciences (Grand Island, NY, USA). EMDs were purchased from Biosera AB, Straumann Emdogain, a company of the straumann Group (SE-205 12 Malmo, Sweden). All other reagents were of the highest available quality and obtained through common commercial sources.
Animals
Female Wistar albino rats (250-300 g) housed in a thermostatically-maintained room (22°C, relative humidity 55.5%) with a 12 h light cycle (light from 6:00 am to 6:00 pm), free access to food and tap water and were mated overnight with males of proven fertility (n = 6). The appearance of a vaginal plug on the next morning was considered as an indication of pregnancy. The day of the appearance of the plug was designated as day 0 of gestation. Pregnant females were scarified on day 13 post coitum and the uteri were explanted aseptically and embryos (34-36 somites stage) placed in phosphate buffered saline prewarmed to 37°C under sterile conditions. Midbrain tissue was removed from the embryos postmortem. All the experiments were conducted in accordance with the accepted principles for laboratory animal use and care in TUMS Animal Research Ethics Committee.
Preparation of neural crest cells
The midbrain tissues were isolated from a number of embryos, placed and washed in sterile phosphate buffered saline (PBS) followed through placing in a culture medium. Isolation was done by centrifugation (200 g) for 5 min. At the next stage, the tissue fragments incubated in 1x trypsin for 4 min and cells were isolated through centrifugation (300 g for 5 min). The cells were then disaggregated with triturating through a Pasteur pipette. This suspension passed through a sterile 50 μm mesh nylon filter. The resulting single cell suspension was collected and cell viability was determined using trypan blue dye exclusion method. For micromass culture, we used 2 × 106 neural crest cells/mL of stock cell suspension.
Culture medium
RPMI 1640 containing 10% fetal bovine serum supplemented with 455 μg/L L-glutamine and 1% Penicillin/Streptomycin (Gibco, UK) and antibiotics (penicillin100 IU/mL, streptomycin 100 μg/mL).
Micromass culture
Discrete 20 μL-droplets of the cell suspension (2 × 106 cells/mL) were placed in the center of each well in a 6 well cell culture plate. The prepared dishes were incubated at 37°C in 5% CO2, 95% air and 100% humidity for 2 h to allow the cells to adhere. It is important not to disturb the cell during this period. After this time, culture medium was added carefully a final volume of 1 mL.
Study design
The 30 mg/mL EMD stock solution was prepared in 1% Trifluoroacetic acid (TFA) and used to make working solutions in culture medium. Neural crest cells were extracted from midbrain and were allowed to grow to confluence for the first 24 h period. The second day of culture, neural crest cells - extracted from midbrain- were treated with various concentrations of EMD (final concentration of 0.5-8 μg/mL of culture medium) for the next 96 h culture period at 37°C.
Mayer’s hematoxylin staining for cell differentiation assay
At the end of the 5 day culture period, medium was aspirated from each well of the plate and the wells were washed with PBS solution. Cells were then fixed with 300 μL of 10% v/v aqueous Formaldehyde for 5 min. Fixed cells were rinsed with PBS and then were stained with Mayer’s hematoxylin for 30 sec. The excess amounts of stain were flicked out of the wells and the dishes were gently washed with water. Midbrain cultures are then left to dry in room temperature. The number of stained and differentiated cells in 250 cells was counted in four different parts of microscope object. The percentage of stained cells, compared to the control, was reported. Retinoic acid at concentration of 20 μg/mL (3 × 10-3 M), which can inhabit more than 90% of midbrain differentiation, was used as positive control (24).
Neutral red staining for cell viability assay
Three hundred μL of 0.1% neutral red solution was added to each well and incubated for 60 min at 37°C. After this period, the dye was removed and cells were washed with prewarmed PBS. The PBS was replaced with 600 μL acid alcohol (absolute ethanol: 0.1 M citrate buffer, pH = 4.2; 1:1 v/v). The final content was transferred to 96-well plates and was shaken for 20 min at room temperature. The optical density was measured at 540 nm using a plate reader (spectrophotometer).
Analysis and interpretation of data
All experiments were repeated at least three times and the results represented as means ± SEM. The effects of the adding concentrations of EMD were assessed using one-way analysis of variance (ANOVA). P- values equal or bellow 0.05 were considered statistically significant difference.
Results and Discussion
The results of four experiments, shown in Figure 1, indicate that EMD at concentrations of 0.5-2 μg/mL did not show a biologically significant effect in the number of stained differentiated neural crest cells (p > 0.05), compared to positive control (retinoic acid). At higher doses, EMD significantly reduced differentiation (p < 0.001), although the number of cells which were exposed to EMD do not show any statistically significant differences compared to the negative control group. The results presented in Figure 2 demonstrated that the addition of EMD in different doses directly to the culture medium, do not make any significant alteration in cell viability (p > 0.05). Furthermore, no significant difference was shown between EMD-treated and retinoic acid-treated groups (p > 0.05).
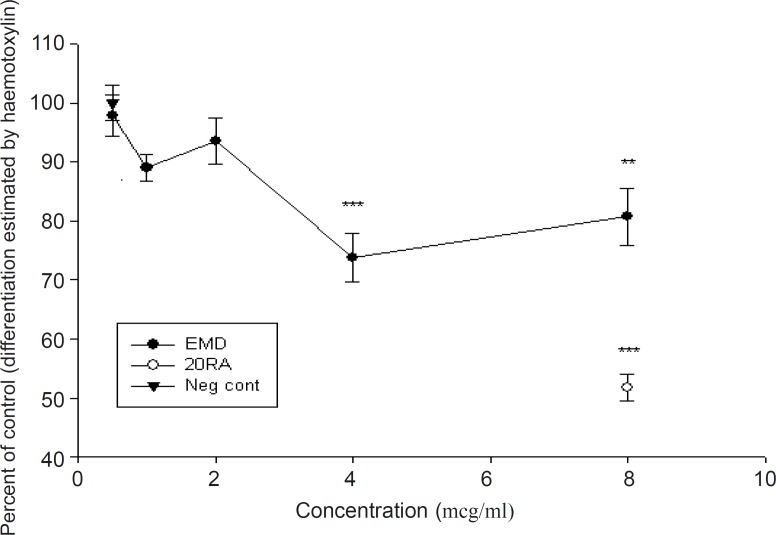
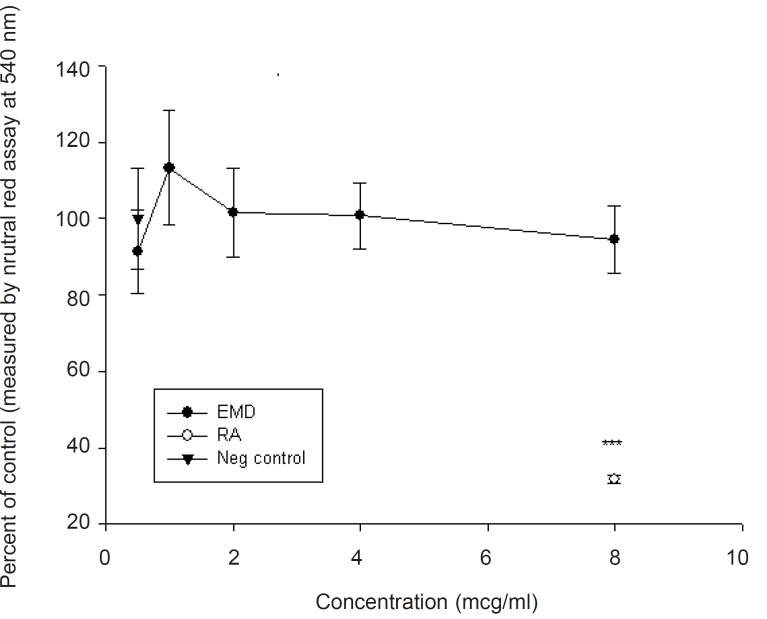
Likewise, EMD did not show a statistically significant reduction when the results of neurogenesis were normalized to neutral red absorbance for the viable cell number (Figure 3)
Nowadays, in-vitro systems have a worldwide use in toxicity testing. An important scope of problem in toxicology studies consists of developmental neurotoxicity. (26-29). Two widely used methods for studying teratogenic characteristics of xenibiotics are neural crest culture which determines the effect of teratogens on the CNS and limb bud culture which shows the effect of teratogens on chondrogenesis. Micromass culture is a suitable tool to improve the number of differentiated cells in both systems (19, 30-33). Neural cell-based models were used in this study to investigate the teratogenic effect of Emdogain.
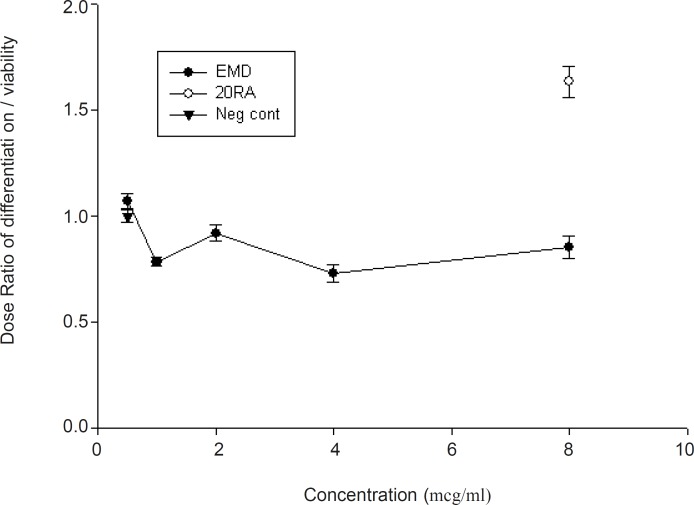
When isolated cells from midbrain of developing embryos are cultured at high density, many of the events (movement, communication, division and differentiation), which are most critical to embryogenesis will occur. Therefore, this model, at least in part, resembles in-vivo developmental process (2). Developmental toxicity (teratogenicity) of Enamel matrix derivative (EMD) was assessed in this study using modified micromass culture method in which neural crest cells from midbrains were used and criteria were analyzed based on one-way analysis of variance (ANOVA). The differentiation was further evaluated utilizing Mayer’s hematoxylin, a stain which aid in visualization of the differentiated neurons nucleus (2, 16).
The results obtained in this assay indicate that there is no teratogenic effect on fetal cell differentiation when EMD is added to the culture of midbrain cells. The viability was evaluated using neutral red assay method. The results demonstrated that EMD do not produce any significant decrease on embryonic cell viability.
Some previous studies have shown an inhibitory effect of EMD on a variety of epithelial cell types: HT 1080 cell line (34), HeLa cell line (35), epithelial carcinoma cells (36) and oral epithelial cells (37). However, since other studies have shown different proliferative responses to EMD stimulation in diverse cell lines, epithelial cells may also respond in a similar variable manner depending upon their type and embryonic origin.
The majority of viability studies conclude that EMD stimulates the growth, proliferation, and mobility of osteoblasts. EMD also regulates the growth, proliferation and mobility of osteoblastic cell lines and cementoblasts, and their gene expression (38, 39). Besides, EMD plays a role in the differentiation of osteoblasts (40). On the other hand, our results show that EMD did not interfere with embryonic neural stem cells in the case of differentiation.
Taken together, these results indicate that EMD has neither cytotoxic nor teratogenic effect on neural crest cells. These data confirm the results of other studies which show no evidence of toxicity on tongue and dental cells (7, 34-40).
References
-
1.
Roider PM. Developing brain as a target of toxicity. Environ. Health Perspect. 1995;Supp16:73-76.
-
2.
Wilk- Zasadna I, Minta M. Developmental toxicity of ochratoxin A in rat embryo midbrain micromass culture. Int. J. Mol. Sci. 2009;10:37-49. [PubMed ID: 19333434].
-
3.
Choi Y Kim EM, Suh YH. Minocycline blocks c-terminal fragments of amyloid precursor protein-induced neurotoxicity by inhibition of cytochrome c release and caspase-12 activation. Iranian J. Pharm. Res. 2004;3:82-82.
-
4.
Grandjean P, Lanndrigan PJ. Developmental neurotoxicity of industrial chemicals. Lancet. 2006;368:2167-2178. [PubMed ID: 17174709].
-
5.
Mendola P, Selevan SG, Gutter S, Rice D. Environmental factors associated with spectrum of neurodevelopmental deficits. Ment. Retard Dev. Disabil. Res. Rev. 2002;8:188-197. [PubMed ID: 12216063].
-
6.
Venzia E, Goldstein M, Boyan BD, Schwartz Z. The use of enamel matrix derivative in the treatment of periodontal defects: Literature review and meta-analysis. Crit Rev. Oral Biol. Med. 2004;15:382-402. [PubMed ID: 15574680].
-
7.
Esposito M, Grusovin MG, Coulthard P, Worthington HV. Enamel matrix derivative (Emdogain) for periodontal tissue regeneration in intra-bony defects. Cochrane Database syst. Rev. 2005:19.
-
8.
Roidrigues TL Marchesan JT, Coletta RD, Novaes AB Jr, Grisi MF, Souza SL, Taba M Jr, Palioto DB. Effects of enamel matrix derivative and transforming growth factor- beta 1 on human periodontal ligament fibroblasts. J. Clin. Periodontal. 2007;34:514-22.
-
9.
Zeldich E, Koren R, Nemocovsky C, Weinreb M. Enamel matrix derivative stimulates human gingival fibroblast proliferation via ERK. J. Dent. Res. 2007;86:41-6. [PubMed ID: 17189461].
-
10.
Takayanagi K, Osava G, Naakaya H, Cochran DL, Kamoi K, Oates TW. Effects of enamel matrix derivative on bone- related mRNA expression in human periodontal ligament cells in-vitro. J. Periodontal. 2006;77:891-8.
-
11.
Sculean A, Schwarz F, Becker J, Brecx M. The application of enamel matrix derivative (Emdogain) in regenerative periodontal therapy: a review. Med. Princ. Pract. 2007;16:167-80. [PubMed ID: 17409750].
-
12.
Wiegand A, Attin T. Efficacy of enamel matrix derivatives (Emdogain) in treatment of replanted teeth a systematic review based on animal studies. Dental Tramatology. 2008;24:498-502.
-
13.
Trope M. Clinical management of the avulsed tooth: Present strategies and future directions. Dent. Traumatol. 2002;18:1-11. [PubMed ID: 11841460].
-
14.
Flores MT, Andreasen, JO, Bakland, LK. Guidelines for the evaluation and management of traumatic dental injuries. Dent. Traumatol. 2001;17:193-8. [PubMed ID: 11678536].
-
15.
Teramoto S, Takahashi Kikuta M, Kobayashi H. Potential teratogenecity of 3-chloro-4-(dichloromethyl)-5-hydroxyd-2(5H)-furanone (MX) in micromass in-vitro test. J. Toxicol. Environment. Health. 1998;53:607-614.
-
16.
Flint OP. In-vitro tests for teratogens: Desirable endpoints, test batteries and current status of the micromass teratogen test. Reprod. Toxicol. 1993;7:103-111. [PubMed ID: 8400628].
-
17.
Kistler A, Howard WB. Testing of retinoids for teratogenecity in-vitro; use of micromass limb bud cell culture. Methods Enzymol. 1990;190:427-433. [PubMed ID: 2087194].
-
18.
Ostad SN, Khakinegad B, Sabzevari O. Evaluation of the teratogenecity of fennel essential oil (FEO) on the rat embryo limb buds culture. Teratology in-vitro. 2004;18:623-627.
-
19.
Flint OP. A micromass culture method for rat embryonic neural cells. Cell Sci. 1983;61:247.
-
20.
Umansky R. The effect of cell population density on the developmental fate of reaggregating mouse limb bud mesenchyme. Dev. Biol. 1966;13:31-36. [PubMed ID: 5332239].
-
21.
Giacobini E. Descrepancies and differences between nerve cells growing in-vitro and in-situ: A discussion. In: Giacobini E, Vernadakis A, Shahar A, editors. Tissue Culture in Neurobiology. New York: Raven press; 1980. p. 187-204.
-
22.
Nelson PG, Bergey G. Pharmacological and developmental studies on mammalian central neurons in cell culture. In: Giacobini E, Vernadakis A, Shahar A, editors. Tissue Culture in Neurobiology. New York: Raven; 1980. p. 221-227.
-
23.
Ahrens PB, Solursh M, Reiter RS. Stage related capacity for limb chondrogenesis in cell culture. Dev. Biol. 1977;60:69-80. [PubMed ID: 198274].
-
24.
Hassell JR, pennypacker JP, Lewis CA. Chondrogenesis and cell proliferation in limb bud cultures treated with cytosine arabinoside and vitamin A. Expl. Cell Res. 1978;112:409-412.
-
25.
Flint OP. The effects of sodium salicylate, cytosine arabinoside, and serine sulfate on rat limb buds in culture. In: Merker HJ, Nau H, Neubert D, editors. Teratology of the Limbs. 4th ed. 1980. p. 325-338.
-
26.
Coeck S, Eskes C, Gartlon J, Kinsner A, Price A, Vliet EV, Prieto P, Boveri M, Bremer S, Adler S, Pellizzer C, Wendel A, Hartung T. The value of alternative testing for neurotoxicity in the context of regulatory needs. Environ. Toxical. Pharmacol. 2006;21:153-167.
-
27.
Coeck S, Goldberg AM, Allen S, Buzanska L, Calamandrei G, Crofton K, Hareng L, Hartung T, Knaut H, Honegger P, Jacobs M, Lein P, Li A, Mundy W, Owen D, Schneider S, Silbergeld E, Reum T, Trnovec T, Monnet-Tschudi F, Bal-Price A. Work group report: Incorporating in-vitro alternative methods for developmental neurotoxicity into interactional hazard and risk assessment strategies. Environ. Health. Perspect. 2007;115:924-931. [PubMed ID: 17589601].
-
28.
Lein P, Silbergeld E, Locke P, Goldberg AM. In-vitro and other alternative approaches to developmental neurotoxicity testing (DNT). Environ. Toxicol. Pharmacol. 2005;19:735-744. [PubMed ID: 21783550].
-
29.
Lein P, Lock P, Goldberg A. Meeting report: Alternatives for developmental neurotoxicity testing. Environ. Health Perspect. 2007;115:764-768. [PubMed ID: 17520065].
-
30.
Flint OP, Orton TC. An in-vitro assay for teratogens with culture of rat embryo midbrain and limb bud cells. Toxicol. Appl. Pharmacol. 1984;76:383-395. [PubMed ID: 6495342].
-
31.
Reinhardt CA. Neurodevelopmental toxicity in-vitro: Primary cell culture methods for screening and risk assessment. Reprod. Toxical. 1993;7:165-170.
-
32.
Walum E, Flint OP. Midbrain micromass cultures: Model for studies of teratogenic and sub-teratogenic effects on CNS development. Acta Physiol. Scand. 1990;592(Suppl. 1):61-72.
-
33.
Whittaker SG, Wroble JT, Silbernagel SM, Faustman EM. Characterization of cytoskeletal and neuronal markers in micromass cultures of rat embryonic midbrain cells. Cell Biol. Toxicol. 1993;9:359-375. [PubMed ID: 8039012].
-
34.
Gestrelius S, Andersson C, Lidstrom D, Hammarstrom L, Somerman M. In-vitro studies on periodontal ligament cells and enamel matrix derivative. J. Clin. Periodontol. 1997;24:685-92. [PubMed ID: 9310873].
-
35.
Kawase T, Okuda K, Yoshie H, Burns DM. Cytostatic action of enamel matrix derivative (EMDOGAIN) on human oral squamous cell carcinoma-derived SCC25 epithelial cells. J. Periodontal. Res. 2000;35:291-300. [PubMed ID: 11005157].
-
36.
Rincon JC, Haase HR, Bartold PM. Effect of Emdogain on human periodontal fibroblasts an in-vitro wound-healing model. J. Periodontal Res. 2003;38:290-5. [PubMed ID: 12753367].
-
37.
Kawase T, Okuda K, Yoshie H, Burns DM. Anti-TGF-beta antibody blocks enamel matrix derivative-induced upregulation of p21WAFl/cipl and prevents its inhibition of human oral epithelial cell proliferation. J. Periodontal. Res. 2002;37:255-62. [PubMed ID: 12200968].
-
38.
Laaksonen M, Sorsa T, Salo T. Emdogain in carcinogenesis: A systematic review of in-vitro studies. J. Oral Sci. 2010;52:1-11. [PubMed ID: 20339227].
-
39.
Klein MO, Reichert C, Koch D, Horn S, Al-Nawas B. In-vitro assessment of motility and proliferation of human osteogenic cells on different isolated extra cellular matrix components compared with enamel matrix derivative by continuous single cell observation. Clin. Oral Implants Res. 2007;18:40-45. [PubMed ID: 17224022].
-
40.
Jiang J, Goodarzi G, He J, Li H, Safavi KE, Spangberg LS, Zhu Q. Emdogain-gel stimulates proliferation of odontoblasts and osteoblasts. Oral Surg. Oral Med. Oral Pathol. Oral Radiol. Endod. 2006;102:698-702. [PubMed ID: 17052650].
-
41.
Galli C, Macaluso GM, Guizzardi S, Vescovini R, Passeri M, Passeri G. Osteoprotegerin and receptor activator of nuclear factor-kappa B ligand modulation by enamel matrix derivative in human alveolar osteoblasts. J. Periodontol. 2006;77:1223-1228. [PubMed ID: 16805686].