Abstract
Keywords
Inducible nitric oxide synthase Anesthesia CA1 region Morris water maze Spatial memory Protein kinase
Introduction
Memory is a complicated function with poorly understood findings. In process of multiple phases of memory phenomena, various distributed neuronal systems, gene expression, protein synthesis and structural alterations of signaling pathways are involved (1, 2).
The critical role of the hippocampus in memory has been shown in many studies (3-7). After reporting of sever amnesia following temporal lobe resection of hippocampus, a growing body of researches has directed at evaluation of the functional role of the hippocampus in different kinds of memory including spatial memory, declarative memory, explicit memory and relational memory (3, 8).
Various studies demonstrate the effects of anesthetic agents on memory (2, 9-13). The impairment effects of general anesthetics on memory function have been indicated in the previous experiments (2, 13). However, some reports show the memory facilitation of general anesthetics during consolidation phase (2, 14).
A substantial body of evidence has suggested that nitric oxide (NO) has an important role in synaptic plasticity in different brain areas such as cerebellum and hippocampus(15, 16). Nevertheless, findings about the importance of hippocampal nitric oxide in spatial learning and memory are controversial (15). Nitric oxide synthase (NOS) exists in at least three isoforms including eNOS (endothelial NOS), nNOS (neuronal NOS) and iNOS (inducible NOS). iNOS is mediated independently to calcium, but eNOS and nNOS are both stimulated in a calcium dependent manner (15). All nitric oxide synthase (NOS) isoforms including (nNOS, eNOS and iNOS) are expressed in brain throughout ageing and associated pathologies (17-20). iNOS is localized in the dentate gyrus and CA1 region of hippocampus that were identified by immunohistochemistry (IHC) studies against iNOS (21). Numerous behavioral and molecular studies indicated that one of the primary causes of cognitive impairments is cholinergic dysfunction (8, 21, 22). In addition, it has been reported that the increase of iNOS expression during hypoxia impairs the memory formation by affecting the cholinergic functions via alteration of acetyl cholinesterase activity (8). Moreover, it has been demonstrated that iNOS inhibitors such as aminoguanidine (AG) can ameliorate cholinergic system dysfunctions induced by amyloid beta (Aβ) injections (21).
The N-methyl-D-Aspartate (NMDA) receptor plays an important role in synaptic plasticity and behavioral learning and memory (23-25), because of its high concentration in the hippocampus, cortex and striatum, the brain regions that were necessary for spatial learning and memory (26, 27).
The aim of the present work was to study the effects of intra-hippocampal infusion of 1400W as a selective iNOS inhibitor in both cannulated non-anesthetized and non-cannulated anesthetized animals on spatial memory in Morris water maze.
Experimental
Animals
Male Albino Wistar rats (180-220 g) were obtained from faculty of pharmacy, Tehran University of Medical Sciences, housed in groups of five in stainless-steel cages, and given food and water ad libitum under a standard 12 h light/12 h dark cycle. The animals were trained and tested during the light cycle. All procedures were carried out in consistent with the guidelines for the Care and Use of Laboratory Animals, Tehran University of Medical Sciences. All efforts were made to create minimum suffering and to trim down the number of animals used in this study.
Drugs
1400W (CALBIOCHEM®, Merck KGaA, Darmstadt, Germany) was dissolved in deionized water. Ketamine (Alfasan, Holland) and xylazine (Pantex Holland B.V.) were used for surgical anesthesia. Other chemicals and materials were obtained from commercial sources.
Behavioral training and testing
In this study, 4-day training trials of animals in the Morris water maze task were performed. 1400W was administered immediately after the last trial of training in the fourth day and spatial memory was tested 48 h after the infusions of 1400W. Spatial memory retention was tested in this task by measuring escape latency, traveled distance, and swimming speed parameters with EthoVision system which was bought from Noldus Information Technology company (Wageningen, the Netherlands), as described in our previous studies (4-7). The testing step included 1 block of 4 trials.
1400W microinjections
The animals were anesthetized with ketamine (80 mg/kg) and xylazine (20 mg/kg) to become prepared for stereotaxic surgeries. In cannulated rats, one week after recovery from the surgery, training of animals started in Morris water maze task. 1400W (10, 50 and 100 μM/side), was microinjected bilaterally in a volume of 1 μL/side into the CA1 region of hippocampus via cannulas placed 3.8 mm posterior, 2.2 mm lateral to bregma and 2.7 mm ventral to the surface of the skull, consistent with the atlas of Paxinos and Watson (28). In non-cannulated rats, bilateral infusions were performed directly via a Hamilton syringe (1 μL/side) into the CA1 region of the hippocampus in anesthetized rats. In all groups, 1400W was infused immediately after the last trial of training in the fourth day. The control groups received deionized water.
Statistics
One-way analysis of variance (ANOVA) was used for comparison of behavioral data. A Newman–Keuls multiple comparison post hoc test was employed to assess differences in behavioral scores. T-test was also used to compare the statistical differences between cannulated and non-cannulated groups. A P-value of 0.05 or less was considered statistically significant.
Results
Effects of four days training in cannulated animals after surgical recovery and intact (non-cannulated) rats before anesthesia
In this study, all animals including control groups and animals selected to receive bilateral infusions of 1400W in anesthetized (non-cannulated) and non-anesthetized (cannulated) conditions, trained completely for four days in the Morris water maze task (MWM) as pointed out by reduction in time and distance for finding the hidden platform (Table 1).
Effects of four days of training on escape latency, traveled distance and swimming speed in cannulated and non-cannulated animals
Training days | Escape latency (sec) | Traveled distance (cm) | Swimming speed (cm/sec) | |||
---|---|---|---|---|---|---|
Cannulated | Non-cannulated | Cannulated | Non-cannulated | Cannulated | Non-cannulated | |
Day 1 | 29.8 ± 4.2 | 42.8 ± 5.9 | 658.4 ± 21.1 | 862.8 ± 129 | 17.93 ± 1.5 | 18.3 ± 1.8 |
Day 4 | 8.6 ± 2.2 ** | 11.63 ± 2.5 *** | 200.3 ± 49 ** | 210.5 ± 59 *** | 24.4 ± 0.7 | 21.2 ± 1.7 |
There were significant differences between the first and fourth days of training in finding the hidden platform in terms of escape latency and traveled distance in cannulated (** P < 0.01) and non-cannulated (*** P < 0.001) animals. Furthermore, there were not any significant differences between the cannulated and non-cannulated animals in spatial learning parameters during training period. There was no significant difference in swimming speed due to the training trials in any of the animal groups (Table 1).
Effects of 1400W microinjection on time and distance of finding the hidden platform during testing trials in cannulated and non-cannulated rats
Post-training bilateral microinjections of 1400W (10, 50 and 100 μM/side) into the CA1 region of the hippocampus via a Hamilton syringe did not change the time, distance, and speed of finding the hidden platform in anesthetized animals (non-cannulated rats) compared to control group (Table 2). However, bilateral intra-hippocampal infusions of this agent via a cannula with dose of 100 μM/side after surgical recovery in consciousness condition led to significant reduction in escape latency and traveled distance (* P < 0.05) (Figures 1A and 1B). The swimming speed was similar in all groups, representing no motor disturbances in all treated animals (Table 2 and Figure 1C).
Effects of 1400W infusions on spatial memory in non-cannulated (anesthetized) animals in Morris water maze
Treatment (μM/side) | Escape latency (sec) | Traveled distance (cm) | Swimming speed (cm/sec) |
---|---|---|---|
Deionized water | 14.8 ± 2.6 | 341.2 ± 53.05 | 24.9 ± 1.2 |
1400W 10 μM | 12.5 ± 1.8 | 313.1 ± 43.6 | 23.8 ± 1.3 |
1400W 50 μM | 9.3 ± 0.99 | 193.6 ± 23.7 | 21.3 ± 1.43 |
1400W 100 μM | 9.9 ± 0.54 | 207.6 ± 21.1 | 21.2 ± 1.5 |
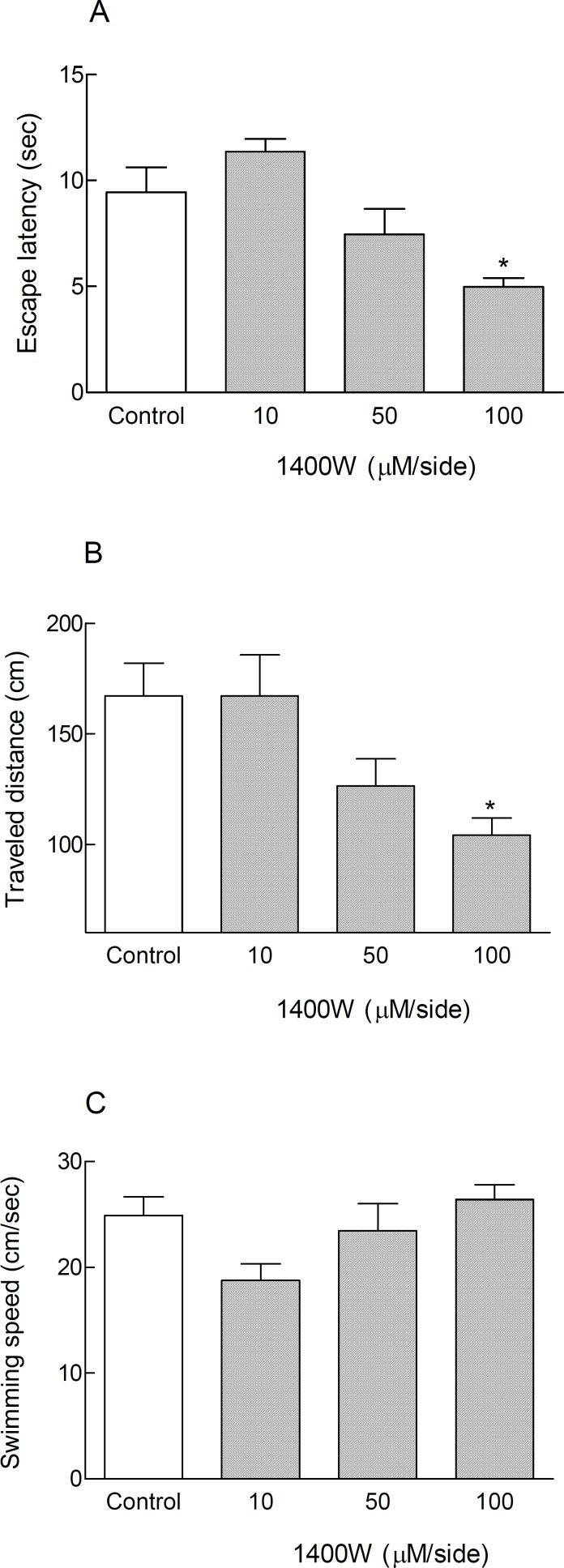
The comparison effects of Post-training administration of 1400W (100 μM/side) between animals in anesthetic condition and post-recovery phase, showed a significant decrease in escape latency and traveled distance (*** P < 0.001) in consciousness rats (Figure 2A and 2B). Moreover, the swimming speed was the same in treated animals (Figure 2C).
In addition, the presence time of the recovered animals during testing trials in target quadrant; the quadrant contained the hidden platform, was 56.7% compared to animals that received 1400W during anesthesia (25.8%) (Figure 2D). In fact, there is a significant difference (*** P < 0.001) between cannulated and non-cannulated rats in time spent in target quadrant (Figure 2D).
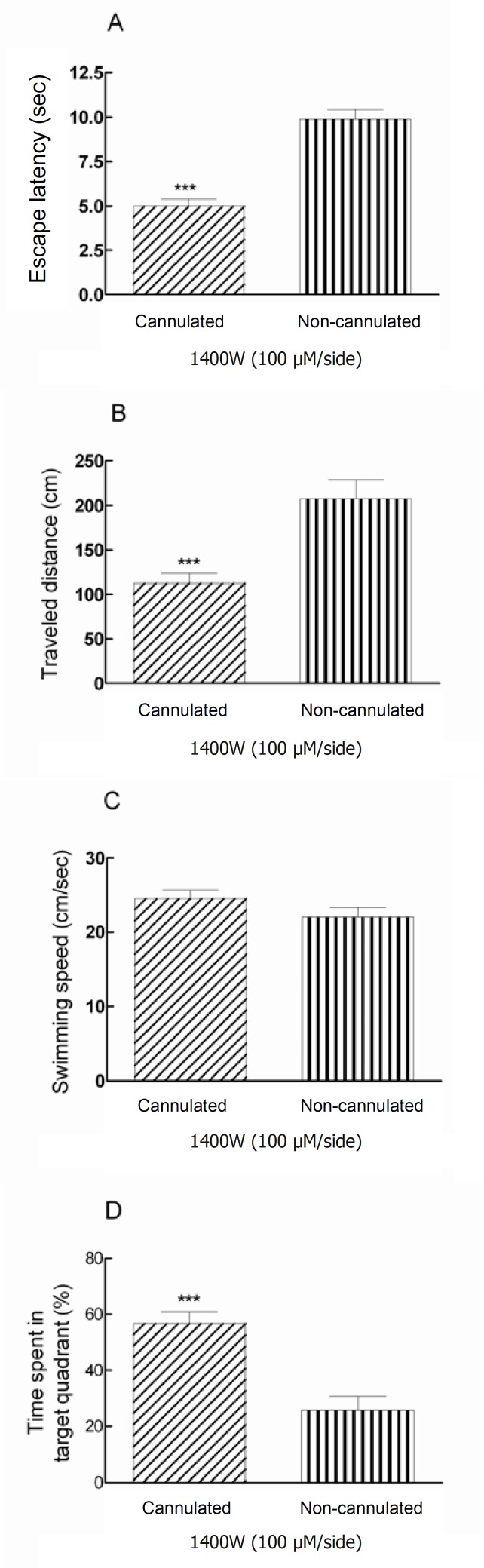
Discussion
In this study, we evaluated spatial memory using Morris water maze, since performing the spatial learning during training and testing trials in this task required the hippocampal neural pathways (4-7, 29). We found no significant differences in escape latency, traveled distance and swimming speed between cannulated animals and intact rats during four days training period. This result confirmed that in both groups of animals, training was completed properly.
In addition post training bilateral infusions of iNOS inhibitor via cannula in recovered animals or by Hamilton syringe in anesthetized rats did not reveal any significant differences in swimming speed comparing to control groups. These observations suggested that 1400W did not induce any motor dysfunctions. Such results support our hypothesis that spatial memory retention improvement is caused by iNOS inhibitor.
One of the important findings of the present investigation is that high dose infusion of 1400W, caused a considerable enhancement on spatial memory in the recovered cannulated animals comparing to the 1400W treated anesthetized rats. There is evidence that shows the involvement of different isoforms of NOS in memory function. The use of specific nNOS inhibitor induced deficits in early olfactory associative learning in Morris water maze and radial maze (30, 31). Some previously published reports indicated that inhibition of eNOS caused memory impairment in chicks (32, 33). In contrast, the role of iNOS inhibitor in attenuation of Aβ-induced memory impairment has also been shown by some investigators (21). It was found that infusion of Aβ1-40 in the brain, could induce iNOS expression which is accompanied with memory loss (21). Also it has been demonstrated that increase in Aβ-induced iNOS expression cause cholinergic system dysfunction (21). The interactions between iNOS and AChE activity was also reported in other studies (8). Due to a more invasive drug administration in non-cannulated (anesthetized) animals comparing to the classically cannulated rats, it is possible that memory improvement induced by 1400W was caused partially by interaction with cholinergic function.
As stated earlier, inducible NOS is calcium-independent which mediate immune function of NO (15). In addition, the effects of anesthesia and mechanical trauma produced by external objects like a needle induced an acute inflammatory immune response that increases the expression of iNOS (34). Hence, it is reasonable to assume that non significant improvement of spatial memory we observed in testing trials of non-cannulated animals (infused with 1400W during anesthesia) was caused by an increase in iNOS levels.
Nitric oxide as a component of the various neurotransmitter pathways is involved in neural plasticity contributing to memory in different areas of brain including the hippocampus (15). The NO/cGMP pathway is influenced by anesthesia (35). Among the anesthetics affecting the NO pathway, ketamine that used in combination with xylazine as an analgesic is widely reported in the published documents and literatures (35). Ketamine-induced cGMP accumulation has been observed in the CNS that suggested its action on the neuronal nitric oxide pathway (35, 36). Ketamine is a non-competitive blocker of the glutamate subtype of the N-methyl-D-Aspartate (NMDA) receptors (23, 35). NMDA receptors that play an important role in neural physiology, synaptic plasticity and behavioral learning and memory (23, 35) are concentrated in the hippocampus (23, 26, 27, 35). A substantial body of evidence also shows the impairment effects of NMDA receptor blockers such as ketamine in different kinds of memory (23). Since, in the study, spatial memory retention 48 h after 1400W infusions, were evaluated in animal tests. Therefore, it is reasonable to deduce that in anesthetic rats the impairment effects of ketamine still remained during testing trials. Furthermore, it is possible in anesthetized animals ketmine-induced hippocampal iNOS increase after 48 h was not inhibited by 100 μM/side of 1400W sufficiently. Moreover, although the effects of ketamine-on nitric oxide in the brain are rather conflicting, caution should be taken when dealing with learning and memory function, in which NO may play an important role. In addition, our findings suggest that ketamine can affect receptors, membranes, ion channels, neurotransmitters, brain blood flow and metabolism in memory processes. In addition, based on the time of spatial memory evaluation after anesthesia, several important factors such as type and distribution of neurotransmitters, metabolic function, capacity for plasticity, depth of anesthesia and root of administration may show different involvement to ketamine-mediated changes. The involvement of cAMP/PKA signaling in relationship between anesthesia and memory in Drosophila has already been reported by Tanaka et al. (13). In their study it was demonstrated that many mutants of general anesthesia and those of memory were overlapped suggested that common molecules and signal pathways were involved in both phenomena (13). We previously showed that cAMP/PKA signaling had important function in spatial memory (5-7). Besides, behavioral studies in Aplysia California, confirmed the pivotal function of cAMP/PKA signaling in the short and long-lasting forms of learning and memory (7, 37–39). Therefore, it is possible that ketamine via affecting on PKA and inhibition of cAMP/PKA pathway may prevent the 1400W-induced memory improvement in anesthetic rats. It is also proposed that cAMP/PKA pathway would increase cholinergic activity (5, 7, 40–42). Consequently, it is probable that ketamine via affecting cAMP/PKA signaling decrease cholinergic function and attenuated memory improvement of 1400W in anesthetic animals.
In conclusion, our findings like those of others, provide documents in support of the interacting effects of anesthesia and iNOS inhibitors on the learning and memory processes in animals. Finding the exact cellular, molecular and neurotransmitters mechanism(s) of these results requires more knowledge of anesthetic agents and 1400W and cAMP/PKA pathway roles in learning and memory, which should be obtained in our future experiments.
References
-
1.
Bailey CH, Bartsch D, Kandel ER. Toward a molecular definition of long-term memory storage. Proc. Natl. Acad. Sci. 1996;93:13445-52. [PubMed ID: 8942955].
-
2.
Culley DJ, Baxter M, Yukhananov R, Crosby G. The memory effects of general anesthesia persist for weeks in young and aged rats. Anesth. Analg. 2003;96:1004-9. [PubMed ID: 12651650].
-
3.
Konkel A, Cohen NJ. Relational memory and the hippocampus: representations and methods. Front. Neurosci. 2009;3:166-174. [PubMed ID: 20011138].
-
4.
Sharifzadeh M, Naghdi N, Khosrovani S, Ostad SN, Sharifzadeh K, Roghani A. Post-training intrahippocampal infusion of the COX-2 inhibitor celecoxib impaired spatial memory retention in rats. Eur. J. Pharmacol. 2005;511:159-166. [PubMed ID: 15792784].
-
5.
Sharifzadeh M, Sharifzadeh K, Naghdi N, Ghahremani MH, Roghani A. Posttraining intrahippocampal infusion of a protein kinase AII inhibitor impairs spatial memory retention in rats. J. Neurosci. Res. 2005;79:392-400. [PubMed ID: 15622518].
-
6.
Sharifzadeh M, Tavasoli M, Naghdi N, Ghanbari A, Amini M, Roghani A. Post-training intrahippocampal infusion of nicotine prevents spatial memory retention deficits induced by the cyclo-oxygenase-2-specific inhibitor celecoxib in rats. J. Neurochem. 2005;95:1078-1090. [PubMed ID: 16150053].
-
7.
Sharifzadeh M, Zamanian AR, Gholizadeh S, Tabrizian K, Etminani M, Khalaj S, Zarrindast MR, Roghani A. Post-training intrahippocampal infusion of nicotine-bucladesine combination causes a synergistic enhancement effect on spatial memory retention in rats. Eur. J. Pharmacol. 2007;562:212-220. [PubMed ID: 17379207].
-
8.
Udayabanu M, Kumaran D, Unnikrishnan Nair R, Srinivas P, Bhagat N, Aneja R, Ketyal A. Nitric oxide associated with iNOS expression inhibits acetylcholinesterase activity and induces memory impairment during acute hypobaric hypoxia. Brain. Res. 2008;230:138-149. [PubMed ID: 18639532].
-
9.
Moller JT, Cluitmans P, Rasmussen LS, Houx P, Rasmussen H, Canet J, Rabbitt P, Jolles, Karsen K, Hanning CD, Langeron O, Johnson T, Lauven PM, Kristensen PA, Biedler A, van Beem H, Fraidakis O, Silverstein JH, Beneken JEW, Gravenstein JS. Long-term postoperative cognitive dysfunction in the elderly ISPOCD1 study: ISPOCD investigators- International study of post-operative cognitive dysfunction. Lancet (1998;351:857-61.
-
10.
Franks NP, Lieb WR. Molecular and cellular mechanisms of general anaesthesia. Nature. 1994;367:607-14. [PubMed ID: 7509043].
-
11.
Magnusson KR, Scanga C, Wagner AE, Dunlop C. Changes in anesthetic sensitivity and glutamate receptors in the aging canine brain. J. Gerontol. A Biol. Sci. Med. Sci. 2000;55:B448-54. [PubMed ID: 10995042].
-
12.
Ingram DK, Garofalo P, Spangler EL, Mantione CR, Odano I, London ED. Reduced density of NMDA receptors and increased sensitivity to dizocilpine induced learning impairment in aged rats. Brain. Res. 1992;580:273-80. [PubMed ID: 1387035].
-
13.
Tanaka Y, Takase M, Gamo S. Relationship between general anesthesia and memory in Drosophila involving the cAMP/PKA pathways and adhesion-related molecules. Curr. Med. Chem. 2007;14(13):1479-88. [PubMed ID: 17584057].
-
14.
Komatsu H, Nogaya J, Anabuki D, Yakondo S, Kinoshita H, Shirakawa Y, Ogli K. Memory facilitation by posttraining exposure to halothane, enflurane, and isoflurane in ddN mice. Anesth. Analg. 1993;76:609-12. [PubMed ID: 8452275].
-
15.
Majlessi N, Choopani S, Bozorgmehr T, Azizi Z. Involvement of hippocampal nitric oxide in spatial learning in the rat. Neurobiol. Learn. Mem. 2008;90:413-419. [PubMed ID: 18508394].
-
16.
Hawkins RD, Son H, Arancio O. Nitric oxide as a retrograde messenger during long term potentiation in hippocampus. Prog. Brain Res. 1998;118:155-172. [PubMed ID: 9932440].
-
17.
Law A, O’Donnell J, Gauthier S, Quirion R. Neuronal and inducible nitric oxide synthase expressions and activities in the hippocampi and cortices of young adult, aged cognitively unimpaired, and impaired Long-Evans rats. Neuroscience. 2002;112:267-275. [PubMed ID: 12044445].
-
18.
Obrenovitch TP, Urenjaka J, Zilkhaa E, Jayb TM. Excitotoxicity in neurological disorders: the glutamate paradox. Int. J. Dev. Neurosci. 2000;18:281-287. [PubMed ID: 10715582].
-
19.
Siles E, Martinez-Lara E, Canuelo A, Sanchez M, Hernandez R, Lopez-Ramos JC et al. Age-related changes of the nitric oxide system in the rat brain. Brain Res. 2002;956:385-392. [PubMed ID: 12445710].
-
20.
Salter M, Knowles RG, Moncada S. Widespread tissue distribution, species distribution and changes in activity of Ca2+-dependent and Ca2+-independent nitric oxide synthases. FEBS Lett. 1991;291:145-149. [PubMed ID: 1718778].
-
21.
Tran MH, Yamada K, Olariu A, Mizuno M, Ren XH, Toshttaka N. Amyloid ß-peptide induces nitric oxide production in rat hippocampus: association with cholinergic dysfunction and amelioration by inducible nitric oxide synthase inhibitors. FASEB J. 2001;15:1407-9. [PubMed ID: 11387239].
-
22.
Tran MH, Yamada K, Nakajima A, Mizuno M, He J, Kamei H, Nabeshima T. Tyrosine nitration of a synaptic protein synaptophysin contributes to amyloid β peptide induced cholinergic dysfunction. Mol. Psychiatry. 2003;8:407-12. [PubMed ID: 12740598].
-
23.
Watson DJ, Stanton ME. Intrahippocampal administration of an NMDA-receptor antagonist impairs spatial discrimination reversal learning in weanling rats. Neurobiol. Learn. Mem. 2009;92:89-98. [PubMed ID: 19248837].
-
24.
Morris RG, Anderson E, Lynch GS, Baudry M. Selective impairment of learning and blockade of long-term potentiation by an N-methyl-D-aspartate receptor antagonist, AP5. Nature. 1986;319:774-776. [PubMed ID: 2869411].
-
25.
Shapiro M. Plasticity, hippocampal place cells, and cognitive maps. Arch. Neurol. 2001;58:874-881. [PubMed ID: 11405801].
-
26.
Wong EH, Kemp JA, Priestley T, Knight AR, Woodruff GN, Iversen LL. The anticonvulsant MK-801 is a potent N-methyl-D-aspartate antagonist. Proc. Natl. Acad. Sci. 1986;83:7104-7108. [PubMed ID: 3529096].
-
27.
Wong EH, Knight AR, Woodruff GN. [3H] MK-801 labels a site on the N-methyl-D-aspartate receptor channel complex in rat brain membranes. J. Neurochem. 1988;50:274-281. [PubMed ID: 2826686].
-
28.
Paxinos G, Watson C. The Rat Brain in Stereotaxic Coordinates. San Diego: Academic Press; 1997.
-
29.
Brandeis R, Brandys Y, Yehuda S. The use of the Morris water maze in the study of memory and learning. Int. J. Neurosci. 1989;48:29-69. [PubMed ID: 2684886].
-
30.
Samama B, Boehm N. Inhibition of nitric oxide synthase impair early olfactory associative learning in newborn rats. Neurobiol. Learn. Mem. 1999;71:219-231. [PubMed ID: 10082641].
-
31.
Holscher C, McGlinchey L, Anwyl R, Rowan MG. 7-Nitro indazole, a selective neuronal nitric oxide synthase inhibitor in vivo, impairs spatial learning in the rat. Learn. Memory. 1996;2:267-278.
-
32.
Rickard NS, Gibbs ME, NG KT. Inhibition of endothelial isoform of nitric oxide synthase impair long-term memory formation in the chick. Learn. Memory. 1999;6:458-466.
-
33.
Rickard NS, Gibbs ME. Hemispheric dissociation of the involvement of NOS isoforms in memory for discriminated avoidance in the chick. Learn. Memory. 2003;10:314-318.
-
34.
Holguin A, Frank MG, Biedenkap JC, Nelson K, Lippert D, Watkins LR, Rudy JW, Maier SF. Characterization of the temporo-spatial effects of chronic bilateral intrahippocampal cannulae on interleukin-1β. J. Neurosci. Methods. 2007;161:265-272. [PubMed ID: 17241670].
-
35.
Alva N, Palomeque J, Carbonell T. Nitric oxide induced by ketamine/xylazine anesthesia maintains hepatic blood flow during hypothermia. Nitric Oxide. 2006;15:64-69. [PubMed ID: 16384721].
-
36.
Galley HF, Le Cras AE, Logan SD, Webster NR. Differential nitric oxide synthase activity, cofactor availability and cGMP accumulation in the central nervous system during anesthesia. Br. J. Anaesth. 2001;86:388-394. [PubMed ID: 11573530].
-
37.
Kandel ER, Schwartz JH. Molecular biology of learning modulation of transmitter release. Science. 1982;218:433-443. [PubMed ID: 6289442].
-
38.
Kandel ER. The molecular biology of memory storage: a dialogue between genes and synapses. Science. 2001;297:1030-1038. [PubMed ID: 11691980].
-
39.
Nguyen PV, Woo NH. Regulation of hippocampal synaptic plasticity by cyclic AMP- dependent protein kinases. Neurobiology. 2003;71:401-437.
-
40.
Inoue H, Li YP, Wagner JA, Hersh LB. Expression of the cholineacetyl transferase gene depend on protein kinase A activity. J. Neurochem. 1995;64:985-990. [PubMed ID: 7861180].
-
41.
Berrard S, Varoqui H, Cervini R, Israel M, Mallet J, Diebler MF. Coregulation of two embedded gene products, choline acetyltransferase and the vesicular acetylcholine transporter. J. Neurochem. 1995;65:939-942. [PubMed ID: 7616258].
-
42.
Berse B, Blusztajn JK. Coordinated up-regulation of choline acetyltransferase and vesicular acetylcholine transporter gene expression by the retinoic acid receptor alpha, cAMP, and leukemia inhibitory factor/ciliary neurotrophic factor signaling pathways in a murine septal cell line. J. Biol. Chem. 1995;270:22101-22104. [PubMed ID: 7673184].