Abstract
Keywords
Rhododendron dauricum flavonoids (RF) Vascular tone Hypoxia Myocardial preservation Vasodilation
Introduction
Because of its low toxicity and good therapeutical performance, traditional Chinese medicine has attracted considerable attention for many fields. Rhododendron dauricum L., referred to as “Man-shan-hong” in Chinese, belongs to the family of Ericaceae, the genera of Rhododendron L. Rhododendron dauricum L. is distributed in the northern part of China, and is a type of medicinal herb often used to cure chronic tracheitis. Chinese Pharmacopoeia (1977 edition) has listed R. dauricum as an official drug (1). Flavonoids are a kind of natural organic compounds with potential physiological activities and effective components of Chinese Herbs, which have attracted more and more research interest and exist in nature extensively. Numerous flavonoids have been reported in the leaves and twigs of R. dauricum. They are mainly composed of farrerol, flavonone, flavonol, flavone and flavonol glucoside (1). Pathological experiments have showed that farrerol moves phlegm and alleviates coughs, and farrerol has been synthesised chemically as an antibechic (2, 3). (Besides moving the phlegm and alleviating cough, other related investigations have shown that flavonoids could have a broad range of physiological activities such as anti- inflammatory, anti-bacterial and antioxidant activity for scavenging radicals).
The mechanisms of R. dauricum flavonoids (RF) on respiratory system have been investigated, in which a decrease in [Ca2+]i of bronchial smooth muscle seems to be involved. It is also well known that intracellular calcium-induced calcium release (CICR) is involved not only in bronchial and vascular smooth muscle, but also in myocardial cells, which could induce the smooth muscle and myocardial cell constriction. It has also been reported that [Ca2+]i overload causes cell damage, as a result of cell undergoing hypoxic and ischemia. Should RF be capable of inhibiting influx of Ca2+ and intracellular calcium release in order to reduce [Ca2+]i, it would be able to avoid damage by calcium overload.
Hence, the present study was designed to examine the effects of on the vascular tone and explore its cardiovascular protective effects, both in vivo and in vitro.
Experimental
Extraction of RF
Rhododendron dauricum (300 g) was extracted twice at 80-100°C, using 3000 mL water for 2 h each time. The extracts were then combined and filtered through filter paper. The filtrate was concentrated at 50-60°C by rotary evaporator to 1800 mL. This crude extract was then dissolved in three volumes 95% ethanol, under constant stirring. The ethanol suspension was laid overnight and then concentrated. Polyamide column chromatography (volume of 400 mL, and a speed of 400 mL/h) was used to enrich the flavonoid components. Non-flavonoid components were first eluted with 1200 mL distilled water and then 1500 mL 95 % ethanol was used to elute the flavonoid components, until reaching a negative reaction of hydrochloric acid-magnesium. The elution speed was about 400 mL/h. The flavonoids suspension was concentrated and then vacuum dried. The flavonoids powder was about 6 g and included hyperoside, quercetin, azaleatin and isorhamnetin.
Assay of flavonoids
The experiments consisted of 3 parts.
(І) Assay of hyperoside
The condition of chromatography as below:
stationary phase: ODS volume (4.6 mm I.D. × 150 mm, 5 μm); mobile phase: methanol: 0.5% phosphoric acid (45: 55) aqueous solution (pH was adjusted with triethylamine to 3); speed was 0.8 mL/min; wave length of detection was 355 nm; and sample size was 10-20 μL. The content of hyperoside was 16.6%.
(ІІ) Assay of azaleatin
The condition of chromatography as below:
stationary phase: ODS volume (4.6 mm I.D. × 150 mm, 5 μm); mobile phase: methanol: water (60: 40); speed was 1 mL/min; wave length of detection was 296 nm; and sample size was 10-20 μL. The content of azaleatin was 1.1%.
(ІІІ) Assay of quercetin
The condition of chromatography as below:
stationary phase: ODS volume (4.6 mm I.D. × 150 mm, 5 μm); mobile phase: methanol: water: glacial acetic acid (50: 50: 2); speed was 1 mL/min; wave length of detection was 370 nm; and sample size was 10-20 μL. The content of quercetin was 3.8%.
Preparation of thoracic arterial rings
Male Wistar rats were purchased from Harbin Medical University animal center. The rats (each weighing 250 - 300 g) were sacrificed by decapitation. The thoracic aortas were rapidly and carefully dissected and placed into ice-cold and oxygen-saturated K-H buffer solution (pH=7.4) containing 118 mmol/l NaCl, 4.7 mmol/l KCl, 1.1 mmol/L MgSO4, 1.2 mmol/L KH2PO4, 1.5 mmol/L CaCl2, 25 mmol/L NaHCO3, and 10 mmol/L glucose. The thoracic aortas were cleaned of connective tissue, and cut into approximately 3-4 mm-wide strips. For endothelium-intact strips, extreme care was taken to avoid endothelium injury. For endothelium-denuded strips, the endothelium was removed by rubbing the vessel interior with wet filter paper. Each vascular strip was mounted in a 5-mL organ bath containing K-H buffer solution. One end of the aortic strip was attached to a metal hook and the other end was connected to an isometric force transducer (JH-2, metrical range: 0-10.0 g) in a bath containing K-H buffer solution, maintained at 37°C and bubbled with 95% O2 and 5% CO2. Rings were equilibrated for 45 min at 1.5 g resting tension, and then challenged with PE (1.0×10-5 M) until a maximal contractile response was obtained. The integrity of the endothelium was assessed in all preparations by determining the ability of carbachol (1.0×10-5 M), to induce more than 80% relaxation of rings. The endothelium was considered to be removed when there was less than 10% relaxation response to carbachol. The isometric tension was recorded with MedLab BL-420E+ recording system (Chengdu TME Technology Co., China).
Effects of RF on vascular tone
The experimental set up were consisted of 4 groups, with each group including six test animal.
(I) Endothelium-dependent or independent effects of RF on isolated aortic rings
When the tension was at resting state or reached a plateau induced by PE (1.0×10-5 M) or KCl (60 mM), RF (0.5, 1, 2, 3, 4 g/L) was cumulatively added into the organ bath at 4 min intervals. The rings with intact or denuded endothelium were always tested in parallel.
(II) Effect of L-NAME (10-5 M) pretreatment on RF induced vasorelaxation in rat aortic rings with endothelium
Aortic rings were pretreated with NOS inhibitor L-NAME (10-5 M) 30 min before contraction with PE. Then RF (0.5, 1, 2, 3, 4 g/L ) was cumulatively added into the organ bath at 4 min intervals to examine a role of L-NAME.
(III) Effect of potassium channels blocking agents pretreatment on RF induced vasorelaxation in rat aortic rings without endothelium
Aortic rings were pretreated respectively with potassium channels blocking agents, including 4-AP (1 mM), TEA (1 mM), Gli (10 μM) and BaCl2 (10 μM) 30 min before contraction with PE. Then RF (0.5, 1, 2, 3, 4 g/L) was cumulatively added into the organ bath at 4 min intervals to examine the role of potassium channel blocking agents.
(IV) Effect of RF and KCl pretreatment in K-H buffer solution without Ca2+ on Ca2+-induced contraction in isolated aortic rings
Aortic rings were pretreated with RF (2 mg/mL) and KCl (60 mM) in K-H buffer solution without Ca2+ 30 min before contraction with Ca2+ (4). Then Ca2+ (0.5, 1, 2, 4, 8 mM) was cumulatively added into the organ bath at 4 min intervals to examine the role of RF on Ca2+- induced contraction.
(V) Effect of RF pretreatment in K-H buffer solution without Ca2+ on PE-induced contraction in isolated aortic rings
Aortic rings were pretreated with RF (2 mg/mL) in K-H buffer solution without Ca2+ 30 min before contraction with PE. Then PE (1.0×10-5 M) was added into the organ bath at 4 min intervals to examine the role of RF on PE- induced contraction.
Preparation of rat cardiomyocytes
Myocytes were isolated according to the method established by Yang et al. (5). Briefly, each rat was killed, then the heart was quickly removed and cannulated on a Langendorff apparatus and retrogradely perfused through the aorta with the standard Tyrode’s solution (126 mM NaCl, 5.4 mN KCl, 10 mN HEPES 0.33 mN, NaH2PO4·2H2O 1.0 mN, MgCl2·6H2O, 1.8 mN CaCl2 and 10 mN glucose; pH=7.4) for 5 min, and calcium-free Tyrode’s solution until it stopped beating. The heart was then enzymatically digested with the calcium-free Tyrode’s solution, containing collagenase type II and BSA. The ventricular tissue was minced after being softened and placed in the KB medium (70 mM glutamic acid, 15 mN taurine, 30 mN KCl, 10mN KH2PO4, 10 mN HEPES, 5.4 mN MgCl2·6H2O, 10 mN glucose and 0.5 mN EGTA; pH=7.4). Single cells were obtained by gentle pipetting. It was stored at 4°C for 1–2 h, and then gassed with 95% oxygen and 5% carbon dioxide and warmed to 37 ± 0.5°C. Only rod-shaped myocytes with clear cross-striations were studied.
[Ca2+]i measurement
After the isolation of single ventricular myocytes, they were adhered to the cover-slips of the chamber. Cells were then rinsed once with the normal Tyrode’s solution and subsequently incubated with a working solution containing Fluo-3/AM (20 mM) and Pluronic F-127 (0.03%) at 37°C for 45 min. After loading, the cells were washed once with the standard Tyrode’s solution to remove the extracellular Fluo- 3/AM. Fluorescent changes of the Fluo-3/AM-loaded cells were detected by a laser scanning confocal microscope at 488 nm for excitation from an argon ion laser and 530 nm for emission and inverted microscope with 20 × objective. Drugs were added between scans 3 and 4, and the images stored on disks. The fluorescent intensities, both before (F0) and after (Fi) the drug administration, were recorded. The change in [Ca2+] i was represented by the ratio of F i /F0.
Effect of RF on cardiomyocytes
The experiments were carried out on 3 groups, each consisting of twenty test animal.
(І) Effect of KCl on [Ca2+]i
F i was measured after adding KCl (60 mM) to the normal Tyrode’s solution containing 1.0 mmol/ L Ca2+.
(ІІ) Effect of RF on [Ca2+]i elevation induced by KCl (60 mM)
The preparation was pretreated with RF (1~2 mg/mL) for 10 min. F i was then measured after adding 60mN KCl.
(ІІІ) Effect of verapamil on [Ca2+]i elevation induced by KCl (60 mM)
The preparation was pretreated with calcium channel inhibitor verapamil (10 μM) for 10 min. F i was then measured after adding 60 mM KCl.
Effect of RF on hypoxia mice
Fourty male mice (average weight of 20±2 g) were divided into 4 groups: 180 mg/kg RFH group, 90 mg/kg RFL group, control group (normal saline) and 10 mg/kg BN52021 group. Each group received intraperitoneal injection with isoprenaline (0.05 mg/10 g) 15 min after injecting drugs through vena caudalis and then each animal moved into a wide-mouthed bottle (180 mL) containing 10 g nitrica calx to examine the survival time, through the observation of asphyxia.
Rat model of myocardial infarction
Male Wistar rats weighing 230–250 g were randomly divided into five groups: control, ischemia (MI), ischemia-low RF (RFL, 7.5 mg/kg), ischemia-high RF (RFH, 30 mg/kg) and BN52021 (10 mg/kg), respectively. The rats were heparinized (300 U) and anesthetized with IP sodium pentobarbital (40 mg/kg), and were then ventilated using a small animal ventilator at a frequency of 70/min and a tidal volume of 3 mL. The chest was surgically opened and the body temperature was maintained at 37°C by placing the animal on a heating pad. The standard limb lead ECG, together with the arterial pressure, was continuously recorded on a recorder (Nihon Kohden RM 6200; Tokyo). A left thoracotomy was performed via the fourth rib intercostal space and a segment of saline-soaked 5-0 suture was looped around the left anterior descending (LAD) coronary artery, near its origin from the left coronary artery and then the chest was closed quickly.
Measurements of infarction size
The heart was removed from the animal after 60 min of infarction, and then the ventricular tissue was dissected and kept at −4°C overnight. The frozen ventricles were sliced into 2 mm thick sections, and then incubated in 1% triphenyltetrazolium chloride at 37°C in 0.2 M Tris buffer (pH=7.4) for 30 min. While normal myocardium was stained brick red, the infarcted areas remained unstained. The size of the infarcted area was estimated by its volume and weight, as a percentage
e of the left ventricle (6).
Statistical analysis
Data were presented as mean ± SD. Statistical comparison was performed by the Student’s t - test and with an analysis of variance (ANOVA), with a value of p < 0.05 considered as significant.
Results
Effects of RF on isolated aortic rings induced by PE and KCl
RF relaxed PE (10-5 M)-precontracted aortic rings in a dose-dependent manner (Figure 1).
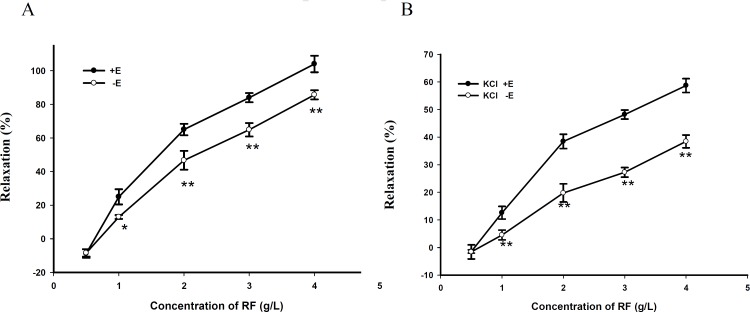
Compared with endothelium - denuded rings, the maximal relaxant effect of RF on rings with endothelium was increased by about 20 ± 1.8% and RF also relaxed KCl (60 mM)-precontracted aortic rings in a dose-dependent manner. Compared with endothelium-denuded rings, the maximal relaxant effect of RF on rings with endothelium was increased by about 22 ± 3/AM. Fluorescent changes of the Fluo-3/AM-loaded cells were detected by a laser scanning confocal microscope at 488 nm for excitation from an argon ion laser and 530 nm for emission and inverted microscope with 20 × objective. Drugs were added between scans 3 and 4, and the images stored on disks. The fluorescent intensities, both before (F0) and after (Fi) the drug administration, were recorded. The change in [Ca2+] i was represented by the ratio of F i /F0.
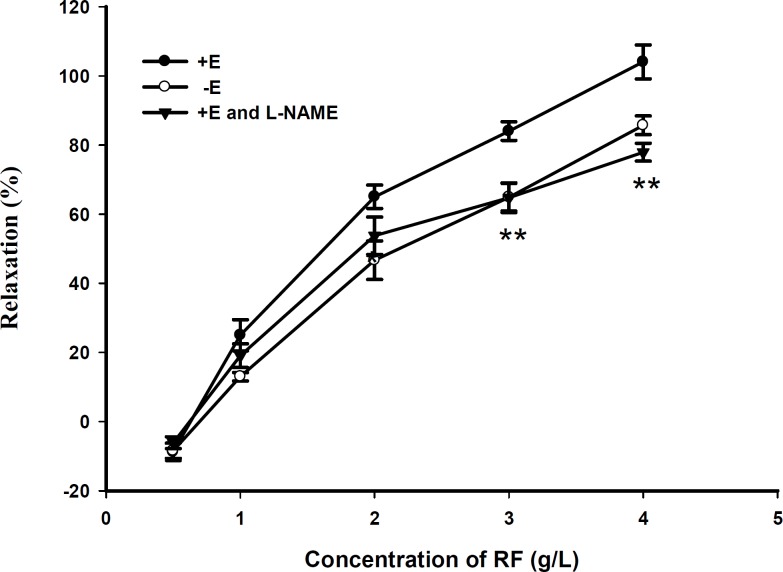
Effect of RF on cardiomyocytes
The experiments were carried out on 3 groups, each consisting of twenty test animal.
(І) Effect of KCl on [Ca2+]i
F i was measured after adding KCl (60 mM) to the normal Tyrode’s solution containing 1.0 mmol/ L Ca2+.
(ІІ) Effect of RF on [Ca2+]i elevation induced by KCl (60 mM)
The preparation was pretreated with RF (1~2 mg/mL) for 10 min. F i was then measured after adding 60mN KCl.
(ІІІ) Effect of verapamil on [Ca2+]i elevation induced by KCl (60 mM)
The preparation was pretreated with calcium channel inhibitor verapamil (10 μM) for 10 min. F i was then measured after adding 60 mM KCl.
Effect of RF on hypoxia mice
Fourty male mice (average weight of 20±2 g) were divided into 4 groups: 180 mg/kg RFH group, 90 mg/kg RFL group, control group (normal saline) and 10 mg/kg BN52021 group. Each group received intraperitoneal injection with isoprenaline (0.05 mg/10 g) 15 min after injecting drugs through vena caudalis and then each animal moved into a wide-mouthed bottle (180 mL) containing 10 g nitrica calx to examine the survival time, through the observation of asphyxia.
Rat model of myocardial infarction
Male Wistar rats weighing 230–250 g were randomly divided into five groups: control, ischemia (MI), ischemia-low RF (RFL, 7.5 mg/kg), ischemia-high RF (RFH, 30 mg/kg) and BN52021 (10 mg/kg), respectively. The rats were heparinized (300 U) and anesthetized with IP sodium pentobarbital (40 mg/kg), and were then ventilated using a small animal ventilator at a frequency of 70/min and a tidal volume of 3 mL. The chest was surgically opened and the body temperature was maintained at 37°C by placing the animal on a heating pad. The standard limb lead ECG, together with the arterial pressure, was continuously recorded on a recorder (Nihon Kohden RM 6200; Tokyo). A left thoracotomy was performed via the fourth rib intercostal space and a segment of saline-soaked 5-0 suture was looped around the left anterior descending (LAD) coronary artery, near its origin from the left coronary artery and then the chest was closed quickly.
Measurements of infarction size
The heart was removed from the animal after 60 min of infarction, and then the ventricular tissue was dissected and kept at −4°C overnight. The frozen ventricles were sliced into 2 mm thick sections, and then incubated in 1% triphenyltetrazolium chloride at 37°C in 0.2 M Tris buffer (pH=7.4) for 30 min. While normal myocardium was stained brick red, the infarcted areas remained unstained. The size of the infarcted area was estimated by its volume and weight, as a percentage of the left ventricle (6).
Statistical analysis
Data were presented as mean ± SD. Statistical comparison was performed by the Student’s t - test and with an analysis of variance (ANOVA), with a value of p < 0.05 considered as significant.
Effects of RF on isolated aortic rings induced by PE and KCl
RF relaxed PE (10-5 M)-precontracted aortic rings in a dose-dependent manner (Figure 1). Compared with endothelium - denuded rings, the maximal relaxant effect of RF on rings with endothelium was increased by about 20 ± 1.8% and RF also relaxed KCl (60 mM)-precontracted aortic rings in a dose-dependent manner. Compared with endothelium-denuded rings, the maximal relaxant effect of RF on rings with endothelium was increased by about 22 ± channels (KATP) inhibitor glybenclamide (Gli) and the inward rectifier K+ channels inhibitor BaCl2 did not play a role on the relaxant effect of RF, compared with the control group (Figure 3).
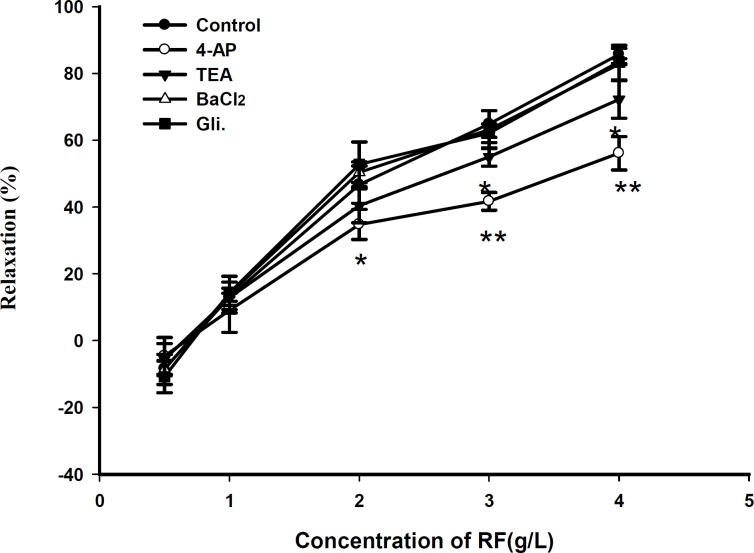
Effect of RF pretreatment in Krebs solution without Ca2+ on Ca2+-induced contraction in isolated aortic rings
The Ca2+- induced contraction and PE induced contraction were obviously attenuated after pretreated with RF (2 mg/mL) for 30 min in Krebs solution without Ca2+ containing 10-4 mol EGTA, compared with the control group (Figure 4).
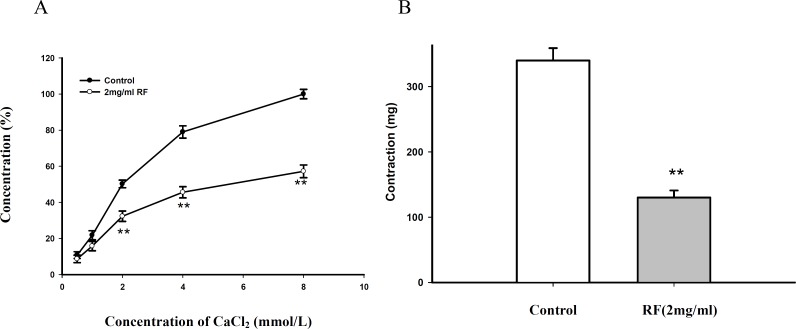
Effect of RF on cardiomyocytes [Ca2+]i
Prior to application of KCl, cardiac myocytes were incubated with either 10 μM verapamil or different doses of RF. Both verapamil and RF inhibited the increase in [Ca2+]i induced by KCl. At the time point of 50s, KCl (60 mM) evoked a F i /F0 increase of 2.94 ± 0.11 fold, [Ca2+]i (Figure 5). In contrast, in the group where cardiac myocytes were pre-incubated with RF, KCl had modest effects on the increase in [Ca2+]i. However, the inhibitory effect of RF on the increase in [Ca2+]i induced by KCl was in a dose-dependent manner. As shown in Figure 6, the value of F i /F0 in the presence of 1 mg/mL RF + KCl was 1.37 ± 0.04. In contrast, the value of F i /F0 in the presence of 2 mg/mL RF plus KCl was 1.06 ± 0.09 ( p < 0.05, n = 20) (Figure 5).
![Dose-effect responses of verapamil and RF on increasing [Ca2+]i induced by KCl. Verapamil 10 μm, RF 1 mg/mL and RF 2 mg/mL inhibited [Ca2+]i evoked by KCl, respectively. Verapamil or RF was applied 10 min before KCl (* p < 0.05, versus KCl group, n=20 for each group).](https://services.brieflands.com/cdn/serve//315db/ec1bbbdcebc44882b52c154d31166b055a97380d/ijpr-09-303-g005.jpg)
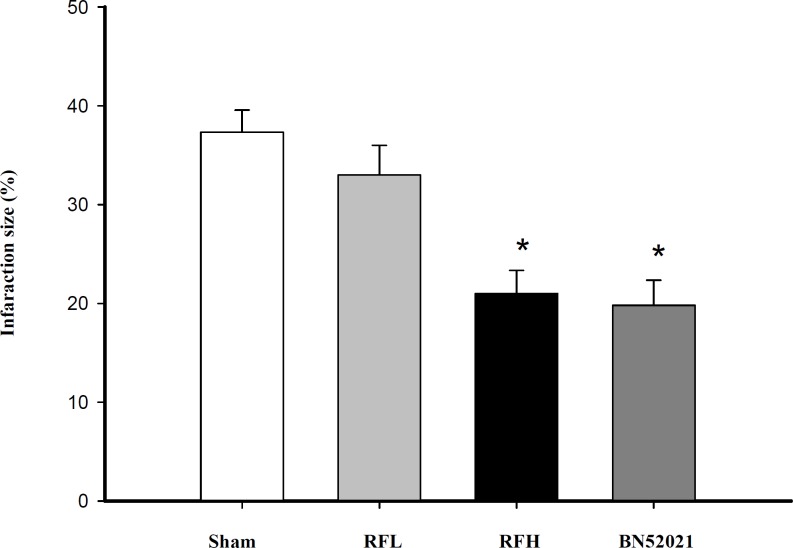
Effect of RF on survival time of hypoxia mice
Compared with the control group, the mice survival time of 180mg/kg RFH group and the positive control group were prolonged by 4 min and 6 min (p < 0.05), respectively. This finding suggested that RF could obviously prolong the survival time in hypoxia mice (Table 1).
Effect of RF on survival time of hypoxic mice (* p < 0.05, versus the control group, n=10 for each group).
Group | Dose | Weight (g) | Time (min) |
---|---|---|---|
Control | 0.2 mL | 20.15 ± 1.09 | 14 ± 5 |
RFH | 180 mg/kg | 19.45 ± 0.75 | 18 ± 3* |
RFL | 90 mg/kg | 21.14 ± 1.47 | 15 ± 3 |
BN52021 | 10 mg/kg | 20.20 ± 0.87 | 20 ± 4* |
Effect of RF on cardial infarction size during ischemia
The results, as shown in Figure 6, indicated that the administration of RFL had a modest effect on the size of infarcted area of rat heart (reduced by 4.32 %, p > 0.05). However, the size of infarcted area was reduced by 16.14% at a higher concentration of RF (30 mg/kg). As a channels (KATP) inhibitor glybenclamide (Gli) and the inward rectifier K+ channels inhibitor BaCl2 did not play a role on the relaxant effect of RF, compared with the control group (Figure 3).
Effect of RF pretreatment in Krebs solution without Ca2+ on Ca2+-induced contraction in isolated aortic rings
The Ca2+- induced contraction and PE induced contraction were obviously attenuated after pretreated with RF (2 mg/mL) for 30 min in Krebs solution without Ca2+ containing 10-4 mol EGTA, compared with the control group (Figure 4).
Effect of RF on cardiomyocytes [Ca2+]i
Prior to application of KCl, cardiac myocytes were incubated with either 10 μM verapamil or different doses of RF. Both verapamil and RF inhibited the increase in [Ca2+]i induced by KCl. At the time point of 50s, KCl (60 mM) evoked a F i /F0 increase of 2.94 ± 0.11 fold, [Ca2+]i (Figure 5). In contrast, in the group where cardiac myocytes were pre-incubated with RF, KCl had modest effects on the increase in [Ca2+]i. However, the inhibitory effect of RF on the increase in [Ca2+]i induced by KCl was in a dose-dependent manner. As shown in Figure 6, the value of F i /F0 in the presence of 1 mg/mL RF + KCl was 1.37 ± 0.04. In contrast, the value of F i /F0 in the presence of 2 mg/mL RF plus KCl was 1.06 ± 0.09 ( p < 0.05, n = 20) (Figure 5).
Effect of RF on survival time of hypoxia mice
Compared with the control group, the mice survival time of 180mg/kg RFH group and the positive control group were prolonged by 4 min and 6 min (p < 0.05), respectively. This finding suggested that RF could obviously prolong the survival time in hypoxia mice (Table 1).
Effect of RF on cardial infarction size during ischemia
The results, as shown in Figure 6, indicated that the administration of RFL had a modest effect on the size of infarcted area of rat heart (reduced by 4.32 %, p > 0.05). However, the size of infarcted area was reduced by 16.14% at a higher concentration of RF (30 mg/kg). As a positive control, 10 mg/kg of BN52021 was applied, which reduced the size of infarcted area of heart by 17.52% (Figure 6).
Discussion
Rhododendron dauricum is a type of medicinal herb often used to cure chronic tracheitis. The related literatures have demonstrated that the flavonoids present in the leaves of R. dauricum (such as Farrerol) could, relieve coughs and move phlegm. These flavonoids are thought to reduce the [Ca2+]i , which can induce relaxation of tracheal smooth muscle and decrease gland excretion. There are several novel fingings in this present study: (i) the RF exerted a vasorelaxant effect on the phenylephrine and KCl contracted aortic ring with or without intact endothelium. (ii) Several mechanisms were involved, such as inhibition of influx of extracellular Ca2+ and release of Ca2+ from sarcoplasmic reticulum, contributing to NO release from intact endothelium, activation of voltage dependent K+ channels (KV) and calcium dependent K+ channels (KCa). (iii) In the rat myocardial cells, incubation with RF could dose-dependently decrease [Ca2+]i elevation by KCl, as observed by laser scanning confocal microscopy. (iv) In the animal experiments, RF could significantly prolong the hypoxia endurance time in mice and protect cardiac myocytes in the coronary artery ligation experiment. Based on these findings, we concluded that flavonoids are the active components of Rhododendron dauricum which can exert vasodilatation and myocardial preservation. The potentials of RF on cardiovascular disorder are closely related to a decreased [Ca2+]i content in vascular smooth and cardiac muscle cells.
First of all, NO is a potent vasodilator synthesized in the endothelium by the enzyme NO synthase, and causes vascular smooth muscle cell relaxation through the activation of soluble guanylate cyclase (7). The present study demonstrated that RF, dose-dependently, inhibited the contraction induced by PE and KCl in intact aorta isolated from rats. This vasorelaxant action was partially inhibited by pretreatment with L-NAME. Endothelium-dependent relaxation of RF seemed to be associated with NO signaling, via guanylate cyclase activation, since L-NAME could attenuate this response. The direct endothelium-dependent and independent vasorelaxation should be taken into account, based on this study. Secondly, RF dose-dependently relaxed endothelium-denuded rings contracted with PE and KCl. The cellular mechanism of contraction involved in the response to KCl and PE is different. KCl induces Ca2+ influx via voltage-dependent Ca2+ channels, which further activates Ca2+-induced Ca2+ release through ryanodine-receptor. PE increases intracellular Ca2+ concentration via two mechanisms: (i) it activates receptor-gated Ca2+ channels, and (ii) it mobilizes Ca2+ from intracellular stores via the inositol-1, 4, 5- trisphosphate (IP3) receptor or induces secondly myosin light chain phosphorylation via activating PKC (8, 9). This means that, the vasorelaxant action of RF seems to occur in both the receptor-dependent and voltage-dependent manners in thoracic aortas. This hypothesis was confirmed through further experiments, in which RF inhibited the dose-independent contraction by CaCl2 and dose-independently decreased PE-induced vasocontraction in Ca2+-free Krebs solution. Thirdly, many types of K+ channels have been identified in vascular smooth muscle cells (VSMC) (10), and moreover, activated K+ channels induces hyperpolarization in order to reduce the [Ca2+]i content in smooth muscle cells, which can exert vasodilatation. The TEA, a calcium-activated K+ channel inhibitor, and 4-AP, a voltage-dependent K+ channel inhibitor, weakened the vasodilatory effect of RF. But glibenclamide, an ATP-sensitive K+ channel inhibitor, and BaCl2, an inward rectifier of K+ channel inhibitor, had no influence on the vasodilatory effect of RF. It is concluded that RF could activate the voltage-dependent K+ channel and the calcium-activated K+ channel and encourage the K+ efflux to reduce the [Ca2+]i content in smooth muscle cells.
In this study, isolated vascular rings experiments indicated that RF-induced vasodilative effects partially contributed to the inhibition of [Ca2+]i, and these facts prompted us to testify the RF influence on isolated myocytes. Through the use of laser scanning confocal microscope, we observed that RF could dose-dependently inhibit the increase in [Ca2+]i induced by KCl, and the inhibitory effect did not change with 10 μM verapamil at the concentration of 2 mg/mL. This result confirmed that RF functioned to inhibit the [Ca2+]i on both vascular smooth muscle cells and myocytes, which supported the additional study, proposing that RF could exert cardioprotector effects. It has been proven that reducing the [Ca2+]i content protects myocytes during damage by hypoxia and ischemia (11, 12). The results of his study indicated that RF prolonged survival time of hypoxic mice pretreated with isoprenaline and reduced the myocardial infarction size in rat coronary artery ligation, demonstrating that RF has a potential as a cardioprotector.
In conclusion, it could be said that explored the cadiovascular potency of RF in isolated myocytes, and vascular tissue in this study. Based on these studies, it could be said that so far the pharmacological activities of R. dauricum exceed that of the traditional pharmacodynamic limitations. Hence, RF seem to have the potential for development into a potent drug for use in the cardiovascular system.
Acknowledgements
References
-
1.
Committee of Jiangsu New Medical College. Encyclopedia of Traditional Chinese Medicine. Shanghai: Shanghai Science and Technology Press; 1995. 2506 p.
-
2.
Committee of Sichuan Medical College. Pharmaceutical Chemistry. Beijing: People Hygiene Press; 1979. 92 p.
-
3.
Yin J. Studies and Clinic Application of Traditional Chinese Medicines. Beijing: Chinese Medicine Press; 1997. 311 p.
-
4.
David FL, Montezano ACI, Reboucas NA. Gender differences in vascular expression of endothelin and ETA/ETB receptors, but not in calcium handling mechanisms, in deoxycorticosterone acetate-salt hypertension. Braz. J. Med. Biol. Res. 2002;359:1-8.
-
5.
Yang BF, Shan HL, Gong DM. Study on the best targets of antiarrhythmic drugs. J. Harbin Univ. Commer. (Nat. Sci. Ed.). 2002;18:1-5.
-
6.
Stamm C, Friehs I, Cowan DB. Post-ischemic PKC inhibition impairs myocardial calcium handling and increases contractile protein calcium sensitivity. Cardiovasc. Res. 2001;51:108-121. [PubMed ID: 11399253].
-
7.
Tanaka Y, Koike K, Toro L. MaxiK channel roles in blood vessel relaxations induced by endothelium-derived relaxing factors and their molecular mechanisms. Smooth Muscle Res. 2004;40:125-153.
-
8.
Eekert RE, Karsten AJ, Utz J. Regulation of renal artery smooth muscle tome by alpha 1-adrenoceptors: role of voltage-gated calcium stores. Urol. Res. 2000;28:122-127. [PubMed ID: 10850635].
-
9.
Kobayashi S, Gong MC, Somlyo AV, Somlyo AP. Ca2+ channel blockers distinguish between G protein-coupled pharmacomechanical Ca2+ release and Ca2+ sensitization. Am. J. Physiol. 1991;260:364-370.
-
10.
Nelson MT, Quayle, JM. Physiological roles and properties of potassium channels in arterial smooth muscle. Am. J. Physiol. 1995;268:799-822.
-
11.
Murphy E, Cross H, Steenbergen C. Sodium regulation during ischemia versus reperfusion and its role in injury. Circ Res. 1999;84:1469-1470. [PubMed ID: 10381900].
-
12.
Piper HM, Meuter K, Schafer C. Cellular mechanisms of ischemia reperfusion injury. Ann. Thorac. Surg. 2003;75:644-648.