Abstract
Keywords
Quercetin G6PD-deficiency Oxidative stress Oxygen-free radical scavenger Erythrocytes Vitamin C
Introduction
Glucose-6-phosphate-dehydrogenase deficiency is a hereditary disorder with higher potential for oxidative damage, due to chronic redox imbalance in red blood cells, that often results in clinical manifestation of mild to severe haemolysis in patients with these disorders. G6PD deficient subjects are at high risk of developing haemolytic anemia, when exposed to exogenous oxidative agents (1). G6PD deficiency-related haemolysis is particularly serious in the neonates, as the excessive unconjugated bilirubin released from haemolysed erythrocytes can pass through the blood brain barrier and enter the brain, thereby causing neurological complications including mental retardation, convulsion, cerebral palsy, hearing deficit, or even death (2) .
The most effective management strategy for G6PD deficiency is to prevent haemolysis, by avoiding oxidative stressors (such as drugs and Vicia faba beans). This approach, however, requires the patient to be aware of their deficiency, as a result of a previous haemolytic episode or a screening program. Fortunately, acute haemolysis in G6PD-deficient individuals is usually short lived, and does not need a specific treatment. In rare cases (usually children), acute haemolysis leading to severe anemia would require transfusion of red blood cells (3).
In organisms, hydrogen peroxide (H2O2) is naturally produced as a by- product of oxygen metabolism; hence, virtually all possess enzymes known as peroxidases. H2O2 is useful for inducing experimental oxidative stress in the cells and for studying its oxidative status in physiological and pathological situations. Several studies have described the antioxidant activities of flavonoids, as hydrogen-donors and free-radical scavengers. These observations raise the possibility of using flavonoids as therapeutic drugs to prevent oxidative alterations in G6PD-deficient erythrocytes. Antioxidant agents such as selenium, vitamin C and α-tocopherol have been shown to prevent the haemolytic crises of G6PD deficient subjects (4-7). Ginkgo biloba extract has been reported to have antioxidant activity and to prevent oxidative damage in erythrocytes. Its antioxidative capacity has been reported to be more effective than vitamin C (8, 9). Quercetin (3, 30, 40, 5, 7-pentahydroxylflavone) is a typical flavonoid, present in fruits and vegetables, and has attracted a great deal of attention as a potential antioxidant (10). There has been no report of known adverse effects from the use of quercetin in the published medical literature (11, 12). However, its antioxidative effect has not been studied in G6PD deficiency.
The aim of this study was to investigate the protective effects of quercetin against H2O2-induced oxidative damage in human erythrocytes. Antioxidant status was examined and the potential application of quercetin, compared and contrasted with vitamin C.
Experimental
This study was approved by the Clinical Research Ethics Committee, Shiraz University of Medical Sciences. All chemicals were purchased form Merck Chemical Company, with the best and purest grade available.
Subject recruitment
Human peripheral blood samples (20 mL) collected by venipuncture from 10 (mean age of 19 ± 8 years) G6PD-deficient and 10 healthy matched donors, with informed consents, were stored in adenine citrate dextrose at 4 °C. All analyses were carried out within 24 h.
G6PD screening
Qualitative screening of G6PD was carried out based on the Fairbanks and Klee method (13). Blood samples obtained were centrifuged at 3000 rpm for 10 min at 22 °C, then they were washed three times with 0.9% w/v saline and centrifuged ( at 3000 rpm for 10 min) to obtain samples of constantly packed cells. Finally, erythrocyte suspensions (10% v/v) were prepared in pH 7.4 phosphate buffered saline (PBS).
Ten microliters of different blood samples were individually added to tubes containing 100 μl of a solution consisting of 50 mM glucose-6-phosphate, 7.5 mM NADP+, 0.5 g/dL saponin, 4 mM oxidized glutathione and 0.7 M of pH7.8 tris–HCl buffer. The blood samples were individually mixed and then 10 μL aliquots immediately transferred onto a 1×1 cm filter paper. After 5 min, this procedure was repeated. Spots were allowed to dry and then examined under a long-wave (320–400 nm) 150 W lamp. Florescence of specimens was compared with positive and negative controls.
The presence of Heinz bodies in freshly drawn blood samples were observed in order to approve G6PD deficient red blood cells after challenging with 0.1mg/mL α–naphthol.
Hb level and RBC count were also measured, using a DAX-48 autoanalyzer in all subjects.
Incubation of RBC suspension with H2O2and quercetin
2.5 mL of the normal and G6PD-deficient erythrocyte suspension samples were treated with quercetin (0.1-150 mM) and vitamin C (250 mM) for 2h (1h before and 1h after incubation with 20 mM of H2O2) at 37 °C. After 1 h of H2O2 treatment, levels of TBA-reactive substances (TBARS) and GSH content were measured.
Determination of lipid peroxidation
The lipid peroxidation of red blood cell (RBC) membrane was assessed, as described by Stocks and Dormandy (14). After a 2 h incubation period of erythrocytes with quercetin and vitamin C in the absence or presence of H2O2 (20 mM) at 37 °C, 0.5 mL of 25% TCA was added to 1 mL of suspension and the mixture was centrifuged at 1000 g for 5 min.
To 1 mL of the resulting supernatant, 1 mL of 1% thiobarbituric acid (TBA) in 0.05 M NaOH was added followed by boiling for 15 min. The formation of TBARS was used as a measure of lipid peroxidation. The TBARS concentration was determined using a spectrophotometer (BioTek Instruments, Winooski, VT, USA) at 532 nm and the results were expressed as ng MDA/g of haemoglobin.
Determination of GSH
Reduced glutation (GSH) was determined, based on the method of Ellman (15). A 1 mL aliquot of the erythrocytes (0.5 mL of cells percipited by 2 mL of 5% TCA) was taken and 0.5 mL of Ellman’s reagent (0.0198% DTNB in 1% sodium citrate) and 3mL of phosphate buffer (pH 8.0) were added. The developed color was then read at 412 nm. The GSH concentrations in test samples were calculated, with reference to the standard curve of GSH (1–100 μg/mL). The results were expressed as μg/g of haemoglobin.
Statistical analysis
All the experiments were carried out at least in triplicate, using RBC from 10 subjects. Results were tested by one-way ANOVA, followed by usig the Dunnett post-hoc test. The difference between the G6PD-deficient and normal groups was analyzed by student’s t-test. All the statistical tests were carried out at a 5% level of significance (p < 0.05). Data obtained have been expressed as mean ± SD.
Results
The mean RBC count was 5.2 ± 0.03 million/μL in the control and 4.6 ± 0.07 million/μL in the G6PD deficient group. The mean haemoglobin content was 13.93 ± 0.2 g/dL in the control group and 11.3 ± 0.8 g/dL in the deficient group, respectively (data not shown). GSH content and TBARS values were not found to be significant in G6PD deficient and normal erythrocytes. Upon challenge with H2O2 (1-100 mM), there was a significant decrease in GSH levels (p < 0.005) and an increase in TBARS levels in G6PD deficient and normal erythrocytes (Table 1).
Cytotoxicity of H2O2 in normal and G6PD-deficient erythrocytes. 2.5 mL volumes of the normal and G6PD-deficient erythrocyte suspension samples were treated with different concentrations of H2O2 for 1h at 37 °C. After 1 h, TBARS levels and GSH content were measured as described in the experimental section. Results are mean ±SD of 10 different subjects
Addition | Normal | G6PD-deficient | ||
---|---|---|---|---|
ng MDA/g Hb | μg GSH/g Hb | ng MDA/g Hb | μg GSH/g Hb | |
Control | 33±2 | 275±25 | 37±6 | 340±44 |
H2O2 (0.1 mM) | 37±4 | 271±15 | 40±2 | 345±21 |
H2O2 (0.5 mM) | 35±8 | 263±32 | 44±5 | 331±35 |
H2O2 (1 mM) | 51±2* | 210±28* | 62±6* | 267±33* |
H2O2 (20 mM) | 125±8** | 145±28* | 177±6** | 200±23* |
H2O2 (50 mM) | 138±42** | 116±34** | 189±12** | 145±26** |
H2O2 (100 mM) | 132±28** | 108±42** | 123±31** | 141±35** |
Incubation of RBC with quercetin (15-75 mM) for 2 h caused a significant decrease in TBARS levels and an elevation in GSH levels (p < 0.005) in G6PD deficient and normal erythrocytes. The protective effect of quercetin in the G6PD deficient group was higher than that of the normal subjects (Figures 1 and 2). As shown in these figures, the protective effect of quercetin was dose-dependent and concentrations higher than 75 mM did not show any protective effect against H2O2. Furthermore, quercetin itself caused toxicity towards RBC to concentrations of 100 mM and higher (data not shown).
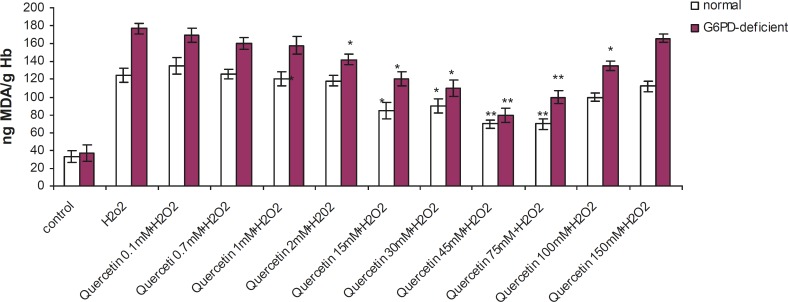
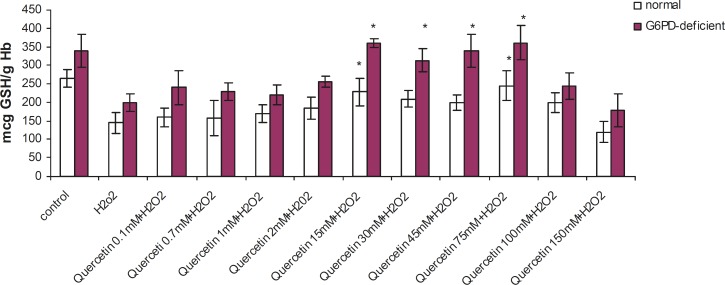
Quercetin was also more efficient than vitamin C in protecting against lipid peroxidation and GSH depletion in G6PD deficient and normal erythrocytes (Table 2).
Protective effect of quercetin and vitamin C against H2O2-induced cytotoxicity in normal and G6PD-deficient erythrocytes. 2.5 mL volumes of the normal and G6PD-deficient erythrocyte suspension samples were treated with quercetin (75 mM) and vitamin C (250mM) for 2h (1h before and 1h after incubation with 20 mM of H2O2) at 37 °C. After 1 h of H2O2 treatment, TBARS levels and GSH content were measured as described in the experimental section. Results are mean + SD of 10 different subjects
Addition | Normal | G6PD-deficient | ||
---|---|---|---|---|
ng MDA/g Hb | μg GSH/g Hb | ng MDA/g Hb | μg GSH/g Hb | |
Control | 33±2 | 275±25 | 37±6 | 340±44 |
H2O2 (20 mM) | 125±8 | 145±28 | 177±6 | 200±23 |
H2O2+ vitamin C (250 mM) | 65±8* | 110±32* | 123±41* | 310±26* |
H2O2+ quercetine (75 mM) | 70±6 ** | 245±40** | 100±7* | 362±45** |
Discussion
There is considerable current interest in the cytoprotective effects of natural antioxidants against oxidative stress and the different defense mechanisms involved (16). This study demonstrates that quercetin, a common dietary flavonoid with a high in-vitro antioxidant activity, has the ability to protect the erythrocytes against an oxidative insult by modulating reduced glutathione concentration and MDA production in the cell.
Enhanced susceptibility against oxidative stress of G6PD-deficient erythrocytes has been reported by several authors (17-19). Various studies have indicated that vitamin E, with and without selenium, can significantly improve the haematological manifestation of the patients with G6PD deficiency (4, 7). Our findings have shown that prior and concomitant administration of vitamin C and quercetin, along with an oxidant agent, can significantly reduce the peroxidative damage of erythrocytes in G6PD-deficient subjects. The antiperoxidative effect of quercetin (depicted in Figure 1) showed that 75 mM of quercetin inhibited the formation of TBARS by 56% (from 177 to 100 ng MDA/gHb in G6PD deficient samples). Prior and concomitant addition of quercetin, along with H2O2, increased GSH levels to 245 and 362 μg GSH/g Hb in normal and deficient erythrocytes, respectively. The antiperoxidative effect of quercetin was also found to be greater than vitamin C. Our findings also indicated that despite its preventive effect in peroxidative conditions, quercetin showed an oxidant characteristic when it was applied directly to erythrocytes in high doses (100 mM and higher). These results are in accordance with the reports on the pro-oxidative activities of some flavonoids on various cancer cell lines (20-22). The pro-oxidative effect of 18 commonly used Chinese herbal remedies on human G6PD-deficient red blood cells has been evaluated by Ko et al. They presented the first evidence on the pro-oxidative action of six different Chinese herbal remedies (Rhizoma Coptidis, Cortex Moutan, Radix Rehmanniae, Rhizoma Polygoni Cuspidati, Radix Bupleuri and Flos Chimonanthi) on G6PD-deficient blood samples (23).
Quercetin is converted to o-quinone by autocatalytic oxidation and the o-semiquinone radical is inevitably produced as a reactive intermediate (24). Oxygen molecules can react with this radical, resulting in the production of O2 and H2O2. These reactive oxygen species (ROS) affect cellular redox signaling pathways and are capable of inducing cellular oxidative damage (25). It is therefore possible that quercetin can act as both a pro-oxidant and an anti-oxidant. This paradoxical phenomenon may explain the reason why quercetin accelerates cellular oxidative damage and/or acts as a mutagen, instead of an anti-mutagen, in a variety of in-vitro studies (26).
Data presented here show that quercetin has protective effects on GSH levels and TBARS. Reduced glutathione (GSH) is the main non-enzymatic antioxidant defense within the cell, reducing different peroxides, hydroperoxides and radicals (alkyl, alkoxyl, peroxyl, etc.) (27). It is usually assumed that GSH depletion reflects intracellular oxidation. On the contrary, an increase in GSH concentration could be expected to prepare the cell against a potential oxidative insult (28-30). Furthermore, it has been reported that GSH levels were significantly lower in G6PD-deficient samples, when compared with the G6PD-normal RBC (31). Based on these findings, it can be concluded that cytoprotective activity of quercetin is related to the increase in the reduced glutathione of G6PD-deficient erythrocytes. Considering the high incidence of G6PD deficiency in specific geographic locations such as Southeast Asia, Mediterranean region and parts of Africa, our findings can be foreseen to in-vivo studies aimed at design of suitable flavonoid-based drugs of importance in preventing free radical induced peroxidation and lysis of erythrocytes.
Acknowledgements
References
-
1.
Magon A, Leipzig RM, Bloom K, Brewer GJ. Pharmacogenetic interactions in G6PD deficiency and development of an in vitro test to predict a drug’s hemolytic potential. Prog. Clin. Biol. Res. 1981;55:709-724. [PubMed ID: 7291202].
-
2.
Fok TF, Lau SP, Hui CW. Neonatal jaundice: its prevalence in Chinese babies and associating factors. Aust. Ped. J. 1986;22:215-219.
-
3.
Mehta A, Mason PJ, Vulliamy TJ. Glucose-6-phosphate dehydrogenase deficiency. Bailliere’s Best. Pract. Res. Clin. Haematol. 2000;13:21-38. [PubMed ID: 10916676].
-
4.
Corash L, Spielberg S, Bartsocas C, Boxer L, Steinherz R, Sheetz M, Egan M, Schlessleman J, Schulman JD. Reduced chronic hemolysis during high-dose vitamin E administration in Mediterranean-type glucose-6-phosphate dehydrogenase deficiency. New Engl. J. Med. 1980;21:416-420. [PubMed ID: 7393270].
-
5.
Kumerova A, Lece A, Skesters A, Silova A, Petuhovs V. Anaemia and antioxidant defense of the red blood cells. Mater. Med. Pol. 1998;30:12-15. [PubMed ID: 10214469].
-
6.
Koga T, Moro K, Terao J. Protective effect of a vitamin E analog, phosphatidylchromanol, against oxidative hemolysis of human erythrocytes. Lipids. 1998;33:589-595. [PubMed ID: 9655374].
-
7.
Hafez M, Amar ES, Zedan M, Hammad H, Sorour AH, el-Desouky ES, Gamil N. Improved erythrocyte survival with combined vitamin E and selenium therapy in children with glucose-6-phosphate dehydrogenase deficiency and mild chronic hemolysis. J. Pediatr. 1986;108:558-561. [PubMed ID: 3958828].
-
8.
Sarikçioğlu SB, Oner G, Tercan E. Antioxidant effect of EGb 761 on hydrogen peroxide-induced lipoperoxidation of G-6-PD deficient erythrocytes. Phytother. Res. 2004;18:837-840. [PubMed ID: 15551377].
-
9.
Foroughinia F, Karimi M. The protective effects of Ginkgo biloba extract in decreasing hemolysis of RBC in Glucose-6-phosphate dehydrogenase-deficient patients. J. Pediatr. Hematol. Oncol. 2007;29:511-512. [PubMed ID: 17609634].
-
10.
Bischoff SC. Quercetin: potentials in the prevention and therapy of disease. Curr. Opin. Clin. Nutr. Metab. Care. 2008;11:733-740. [PubMed ID: 18827577].
-
11.
Ruiz MJ, Fernández M, Picó Y, Mañes J, Asensi M, Carda C, Asensio G, Estrela JM. Dietary administration of high doses of pterostilbene and quercetin to mice is not toxic. J. Agric. Food Chem. 2009;22:3180-3186. [PubMed ID: 19292443].
-
12.
Dunnick JK, Hailey JR. Toxicity and carcinogenicity studies of quercetin, a natural component of foods. Fundam. Appl. Toxicol. 1992;19:423-431. [PubMed ID: 1459373].
-
13.
Fairbanks VF, Klee GG. Biochemical aspects of hematology. In: Burtis CA, Ashwood ER, editors. Tietz Textbook of Clinical Chemistry. Philadelphia: Saunders; 1994. 1983 p.
-
14.
Stocks J, Dormandy TL. The autoxidation of human red cell lipids induced by hydrogen peroxide. Brit. J. Haematol. 1971;20:95-111. [PubMed ID: 5540044].
-
15.
Sedlak J, Lindsay RH. Estimation of total protein-band and nonprotein sulfhydryl group in tissue with Ellman’s reagent. Anal. Biochem. 1968;25:192-205. [PubMed ID: 4973948].
-
16.
Halliwell B. Oxidative stress, nutrition and health: Experimental strategies for optimization of nutritional antioxidant intake in humans. Free Radical. Res. 1996;25:57-74. [PubMed ID: 8814444].
-
17.
Liu TZ, Lin TF, Hung IJ, Wei JS, Chiu DT. Enhanced susceptibility of erythrocytes deficient in glucose-6-phosphate dehydrogenase to alloxan/glutathione-induced decrease in red cell deformability. Life Sci. 1994;55:PL55-60. [PubMed ID: 8007756].
-
18.
Pandolfi PP, Sonati F, Rivi R, Mason P, Grosveld F, Luzzatto L. Targeted disruption of the housekeeping gene encoding glucose 6-phosphate dehydrogenase (G6PD): G6PD is dispensable for pentose synthesis but essential for defense against oxidative stress. EMBO J. 1995;14:5209-5215. [PubMed ID: 7489710].
-
19.
Arese P, Deflora A. Pathophysiology of hemolysis in glucose 6-phosphate dehydrogenase deficiency. Semin. Hematol. 1990;27:1-40. [PubMed ID: 2405494].
-
20.
Galati G, Sabzevari O, Wilson JX, O’Brien PJ. Prooxidant activity and cellular effects of the phenoxyl radicals of dietary flavonoids and other polyphenolics. Toxicol. 2002;177:91-104.
-
21.
Nam TW, Yoo CI, Kim HT, Kwon CH, Park JY, Kim YK. The flavonoid quercetin induces apoptosis and inhibits migration through a MAPK-dependent mechanism in osteoblasts. J. Bone Miner. Metab. 2008;26:551-560. [PubMed ID: 18979154].
-
22.
Choi EJ, Bae SM, Ahn WS. Antiproliferative effects of quercetin through cell cycle arrest and apoptosis in human breast cancer MDA-MB-453 cells. Arch. Pharm. Res. 2008;31:1281-1285. [PubMed ID: 18958418].
-
23.
Ko CH, Li K, Ng PC, Fung KP, Wong RPO, Chui KM, Gu GJS, Yung E, Fok TF. Pro-oxidative effects of Chinese herbal medicine on G6PD-deficient erythrocytes in vitro. Toxicology in vitro. 2008;22:1222-1227. [PubMed ID: 18515042].
-
24.
Metodiewa D, Jaiswal AK, Cenas N, Dickancaité E, Segura-Aguilar J. Quercetin may act as a cytotoxic prooxidant after its metabolic activation to semiquinone and quinoidal product. Free Radic. Biol. Med. 1999;26:107-116. [PubMed ID: 9890646].
-
25.
Rodgers EH, Grant MH. The effect of the flavonoids, quercetin, myricetin and epicatechin on the growth and enzyme activities of MCF7 human breast cancer cells. Chem. Biol. Interact. 1998;116:213-228. [PubMed ID: 9920463].
-
26.
Kawanishi S, Oikawa S, Murata M. Evaluation for safety of antioxidant chemopreventive agents. Antioxid. Redox Signal. 2005;7:1728-1739. [PubMed ID: 16356133].
-
27.
Vin˜a J. Glutathione: Metabolism and Physiological Functions. Boston: CRC Press; 1990. p. 222-228.
-
28.
Scharf G, Prustomersky S, Knasmuller S, Schulte-Hermann R, Huber WW. Enhancement of glutathione and g-glutamylcysteine synthetase, the rate limiting enzyme of glutathione synthesis, by chemoprotective plant-derived food and beverage components in the human hepatoma cell line HepG2. Nutr Cancer. 2003;45:74-83. [PubMed ID: 12791507].
-
29.
Myhrstad MC, Carlsen H, Nordstrom O, Blomhoff R, Moskaug JO. Flavonoids increase the intracellular glutathione level by transactivation of the gamma-glutamylcysteine synthetase catalytical subunit promoter. Free Rad. Biol. Med. 2002;32:386-393. [PubMed ID: 11864778].
-
30.
Alı´a M, Ramos S, Mateos R, Bravo L, Goya L. Response of the antioxidant defense system to t-butyl hydroperoxide and hydrogen peroxide in a human hepatoma cell line (HepG2). J. Biochem. Mol. Toxicol. 2005;19:119-128. [PubMed ID: 15849717].
-
31.
Sushil KJ. Glutathione and glucose-6-phosphate dehydrogenase deficiency can increase protein glycosylation. Free Rad. Biol. Med. 1998;24:197-201. [PubMed ID: 9436631].