Abstract
Keywords
Introduction
Population growth and social and economic development have caused serious damage to the environment in the past 50 years (1). Most medications, including antibiotics, are highly stable in the environment, so their residual presence in the environment, especially in water resources, has become an important problem (2, 3). Antibiotics enter the environment through the household and hospital wastewater, sewage of veterinary clinics and agricultural runoff into sewage collection systems and the environment (4). An average of 70% of the consumed antibiotics enters the sewage without any changes (5). Urban and hospital wastewater treatment plants are not sufficiently effective in eliminating these compounds, therefore the antibiotics can enter the water sources (6).
The expired or unused drug compounds may enter the landfill site. If the right technology is not used in the designing, developing or equipping these sites, their leakage to the surface and groundwater and contaminating the drinking water sources will be inevitable. Biological solids from the sediments in pounds of wastewater treatment plants contain high concentration of these compounds (7, 8). Moreover, there are evidences of accumulation of antibiotics in the environment (9). However, many drug contaminants such as antibiotics and their metabolites can cause complications, such as endocrine dysfunction and microorganism resistance (10, 11). Therefore, today in many review articles the subjects of occurrence and fate of drugs in the environment as well as the effects on humans and other organisms in the environment are emphasized (12). Azithromycin, erythromycin, clarithromycin are among the most important antibiotics in the macrolide group and are widely used in medicine and veterinary clinics (13, 14, 15).
Azithromycin is the result of a change in the erythromycin structure. This compound has different chemical and antimicrobial properties compared to erythromycin, for example, it is more stable in the acidic environments and it is better diffused in tissues. A large amount of this drug is not metabolized in the body and is largely eliminated by the bile (16, 17). Various methods such as adsorbents, chemical compounds such as chlorine and photochemical oxidation are used to remove antibiotics entered into the aquatic environments (18-20). Advanced oxidation processes have been widely used as effective methods for degradation and removal of hazardous and persistent pollutants in many aquatic environments. During these processes free radicals, such as hydroxyl and sulfate radicals, are produced, leading to the decomposition and reduction of toxicity of organic compounds in various environments such as wastewater (21, 22). Sulfate radicals produced in advanced oxidation processes have recently been considered by many researchers and are discussed as an alternative to reduce organic pollutants because they enjoy a high efficiency in removing pollutants that cannot be removed by conventional methods (16). Other advantages of sulfate radicals include high stability, selectivity power, and high electron volts (2.6 ev), moderate costs, and being eco-friendly (22-24). Persulfates are widely used as a precursor of sulfate radicals due to their specific advantages, including relatively low costs, high water solubility, easy storage and pre-activation transportation. Several methods, such as ultraviolet radiation, temperature, intermediate metals, and ultrasonic waves can activate persulfates and produce sulfate radicals (25). Today, advanced oxidation processes are considered in decomposing various pollutants such as medications during which by employing ultrasound, ultraviolet radiation, nanoparticles, and heat. The persulfates are used to produce sulfate radical (Reduction potential equal to 2.6 V) (16, 21, 25-30).
The aim of this study was the comparative removal of azithromycin from sewage using two ultraviolet and ultraviolet/sodium peroxide processes based on the formation of hydroxyl and sulfate radicals. Parameters such as initial concentration of antibiotic, initial persulfate concentration, reaction medium, and reaction time were used as effective factors on system conductance and after obtaining the optimal conditions for the best removal efficiency experiments were evaluated on real wastewater as well.
By obtaining the COD, the removal efficiency of other organic compounds in the sewage was also measured by the combined process of ultraviolet and persulfate radiation. Also, nitrate, phosphate, and bicarbonate anions removal efficiency was also calculated from this process.
Experimental
Chemicals
Azithromycin (C38H72N2O12) 98%, Analytical grade, CAS Number: 117772-70-0 was purchased from the Farma Pharmaceuticals Company Tehran, Iran. The molecular structure of azithromycin is shown in Figure 1. Sodium persulfate (Na2S2O8, 99%), CAS Number: 7775-27-1, of the Sigma Aldrich Company HPLC grade methanol (CH3OH; ≥ 99.9%), CAS Number: 67-56-1, acetonitrile (CH3CN; ≥ 99.5%), CAS Number: 75-05-8, sodium hydroxide (NaOH; ≥ 99.8%), CAS Number: 1310-73-2, and sulfuric acid (H2SO4; ≥ 98%), CAS Number: 7664-93-9, were purchased from Merck-Germany. Standard Stocks Solution of 1000 mgml-1 of antibiotic was prepared by solving 100 mg of azithromycin antibiotic in 100 mL of methanol. Standard Stocks Solution of 50 mmol was prepared by solving 11.9g Sodium persulfate in one liter of deionized water with a resistivity less than 5 Ω. (C18, 6 ml, 500 mg), and also solid phase extraction (SPE) tubes were purchased from INOPAK, Korea. Low pressure mercury lamp (manufactured in OSRAM- Italy) was purchased with a protective quartz coating (6 w, wavelength of 254 nm).
Experimental procedure
The experiments were carried out in a calibrated glass reactor (250 mL volume). The low pressure mercury lamp (6w, wavelength of 254 nm) with a quartz protective tube was submerged in the middle of the reactor’s central cylinder. The effect of ambient light was prevented by wrapping this set in an aluminum foil. The continuous mixing of the specimen was performed by the magnetic stirrer. After preparation of the reactor, an appropriate volume of antibiotic stocks and sodium persulfate solutions were added. Using the sulfuric acid and sodium hydroxide 0.1 N, the pH of the sample was adjusted within the desired range. By turning the mercury lamp on and during the contact time 30, 60, 90 minutes; the entire contents of the reactor were passed through the cartridge. In order to ensure the decomposability, all tests were repeated twice and the standard deviation was considered to be less than 5%.
In this study also for investigating the effectiveness of advanced oxidation (ultraviolet/persulfate and ultraviolet radiation) on the actual wastewater of hospitals a wastewater specimen was collected from hospital in Shahr-e-Kord and analyzed after the transfer to the laboratory. The specimen was first filtered through 0.45 µm filter in the laboratory. The steps of SPE tube preparation, extraction and analysis of specimens were carried out according to the analytical method described in the next section. The concentration of antibiotic studied in the hospital wastewater 5.04 mgL-1 was reported. To compare the two methods of ultraviolet and ultraviolet/persulfate, all conditions were considered the same.
Characteristics of the real samples collected from hospital wastewater before oxidation and after that
Characteristics of the real wastewater | Concentration (mgL-1) | |
---|---|---|
Before oxidation | After oxidation | |
Chemical Oxygen Demand | 415 | 138 |
Bicarbonate | 300.08 | 0 |
Nitrate | 78.97 | 44.22 |
Phosphate | 2.82 | 1.21 |
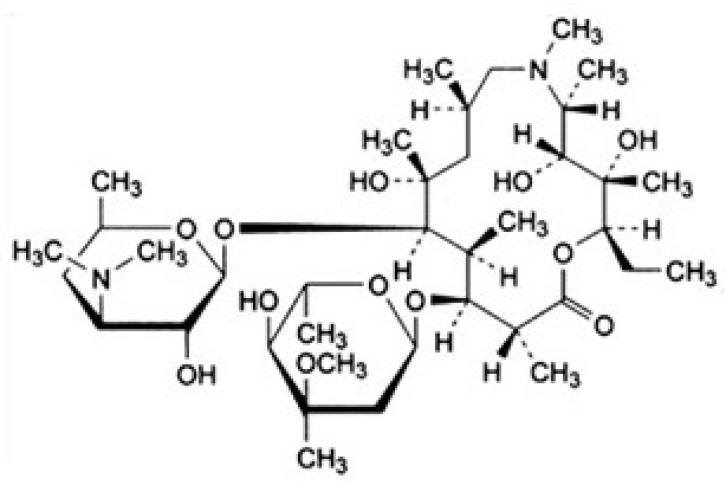
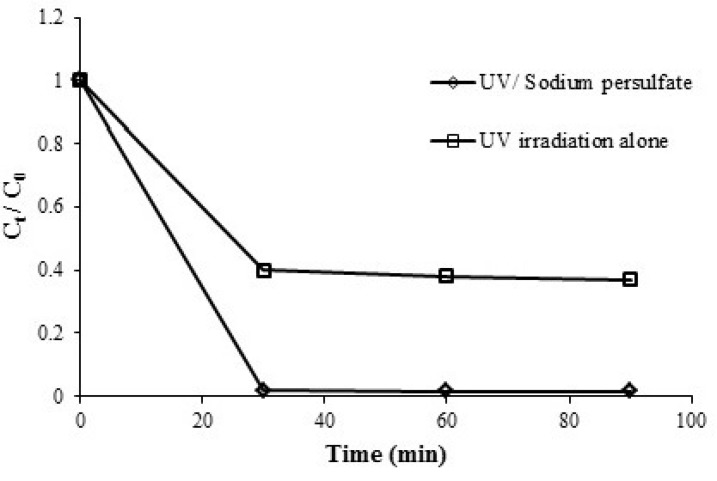
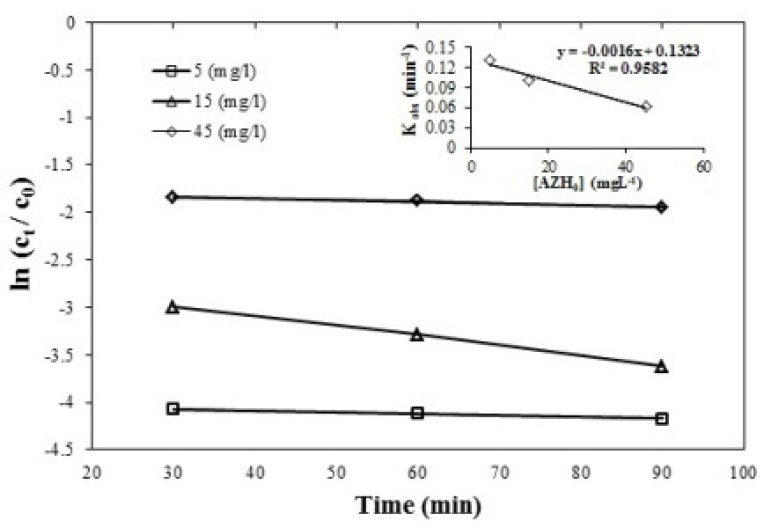
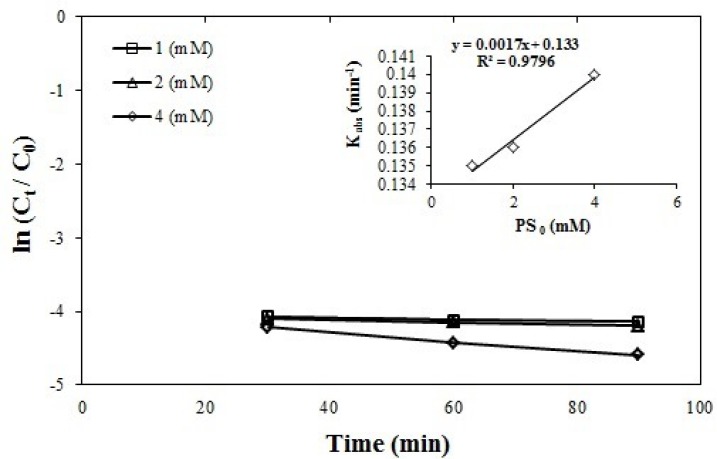
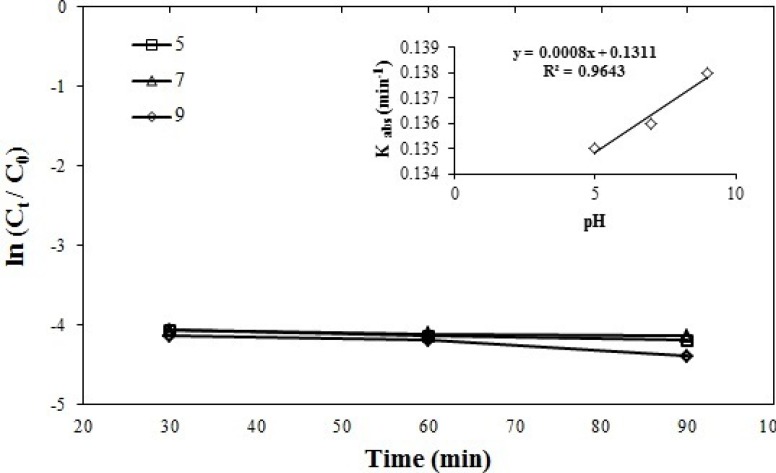
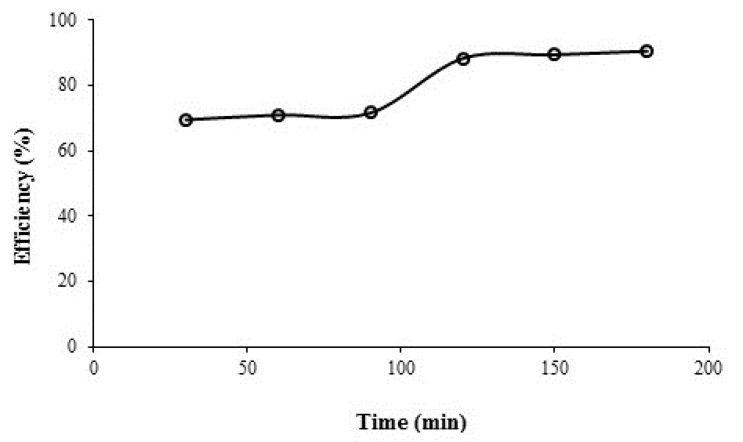
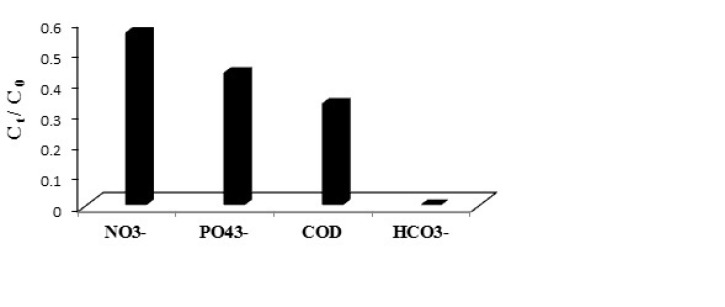
Analytical methods
High Performance Liquid Chromatography equipped with fluorescence detector (HPLC FLD, Agilent series 1200) and column C18 (particle size 5 μm, internal diameter 4.6 mm and length 150 mm) was used in analytical experiments. A mixture of methanol and 0.1% formic acid in the volumetric ratio of (90:10) was used as the mobile phase in a uniform flow of 0.3 ml/min (31). The injected sample size was 20 μl and Isocratic elution was used. In order to separate, purify, and concentrate of contaminants from samples, many methods including SPE were used (32-35). For this purpose, the SPE tube containing the appropriate adsorbent (silica gel) was selected. For the preparation of SPE tubes, 5 ml of methanol and 5 mL of deionized water with less than 5 Ω resistance was passed through them. 200 mL of the filtered sample was added to the flow rate of 1 mL/min to the SPE tube and after the removal of the sample and rinsing the tube, 2 ml of acetonitrile was used for washing and separating the remaining antibiotic from the adsorbent (36, 37). During all these stages the manifold and a vacuum pump were used.
The extracted specimen was collected in a Microtube 2 mL for HPLC injection. 20 μL of the extracted specimens were injected into an HPLC device through a 20 μL loop. Using the equation and calibration diagram, the amount of residual azithromycin was calculated after the advanced oxidation process and using the following equation 1, the amount of azithromycin removal efficiency was measured. The other analysis such as COD, bicarbonate, nitrate, and phosphate were performed based on the protocol in standard methods for the examination of water and wastewater; (38).
Efficiency (%) =
The obtained data were entered into the SPSS software and according to the type of data distribution ANOVA and Bonferroni tests were used. The significance level was considered as pv < 0.05.
Results and Discussion
Comparison of Azithromycin removal by direct photolysis UV alone and UV/persulfate
Azithromycin removal in any of the processes (i.e. UV alone and UV/sodium persulfate) is shown in Figure 2. The removal rate for the initial concentration of 5 mgL-1 obtained under the same conditions in terms of pH = 7, PS = 1 mmol, time = 30 min. 58% and 98% for UV alone and UV/sodium persulfate, respectively. Decrease the amount of Azithromycin followed first order kinetics. In general, the reaction rate constant obtained from the equation 2, was increased when ultraviolet radiation was used alone 0.03 min-1 compared to the case that the ultraviolet light was used with sodium persulfate 0.13 min-1. Increasing the efficiency in UV/sodium persulfate process could be due to the production of sulfate radicals due to the simultaneous use of sodium persulfate and ultraviolet radiation along with the presence of hydroxyl radicals. As a result, the degradation and removal of azithromycin in optimal conditions is increased from 58% (UV alone) to 99% (UV/persulfate).
The study Yu-Qiong Gao et al. on sulfamethazin showed that among the ultraviolet, persulfate, ultraviolet and persulfate and ultraviolet and hydrogen peroxide removal methods the highest removal efficiency was associated with 96.5% the ultraviolet and persulfate process (30). Similar results were observed by Yang Deng et al. on fluorophenicol showed that compared to the UV and UV/persulfate methods, the UV/persulfate method removed 98.4% fluorophenic over an hour (39). The study Moussa Mahdi Ahmed, Serge Chiron on carbamazepine showed that the highest removal efficiency was associated with the UV/persulfate method (40).
-Kabs. t= Ln
where;
[AZH]t: Azithromycin concentration at time
[AZH]0: Initial Azithromycin Concentration
Kabs: First order reactions constant, the minus sign in front of the kabs term is because the concentration of AZH is decreasing over time.
Effect of initial azithromycin concentration on the removal efficiency
Based on the studies, the reaction’s constant was reduced according to the equation
K=) -0.0016 [AZH0] + 0.1323) from 0.13 min-1 at a concentration of 5 mgL-1 to 0.061 min-1 at a concentration of 45 mgL-1 of the azithromycin antibiotic. Azithromycin removal efficiency was compared at three levels of antibiotic concentration 5, 15, 45 mgL-1 by one-way ANOVA. According to the Figure 3 there was a significant difference between the concentrations 5, 45 mgL-1 of azithromycin; the p-value was 0.00 (first type error). With the increase in antibiotic levels, the decrease in antibiotic elimination efficiency is observed. The possible justification of these changes can be because the available sulfate radicals are insufficient to degrade the high concentrations of azithromycin antibiotic (41).
Kordatou Michael et al. on the study of erythromycin removal efficacy with UV/persulfate process, it was concluded that the removal efficiency was reduced by increasing the antibiotic concentration (42). Similar results were observed by the study Shengnan Su et al. of amoxicillin that with the increase in amoxicillin concentration, the removal efficiency is reduced (22).
Effect of initial sodium persulfate concentration on the removal efficiency
The azithromycin removal efficiency was compared at three levels of sodium peroxide concentration (1, 2, 4 mmol) using one-way ANOVA and no statistically significant difference was observed between the three levels of concentration.
The reactions constant was increase according to the equation K = 0.0017 [PS0] + 0.133 for 0.135 min-1 at a concentration of 1 mmol to 0.14 min-1 at a concentration of 4 mmol of the sodium persulfate. These changes are presented in the Figure 4 which is first-order linear. The statistical distribution of the obtained data was normal and was obtained by performing one-way ANOVA. Considering that there is no significant difference between the selected concentrations, from the economic point of view, the concentration of 1 mmol of sodium persulfate was chosen as the optimum concentration because this concentration provides higher efficiency with low difference with other concentrations 90%.
Yu-qiong, Gao et al. in their study on the removal of sulfamethazine and fluorophenicol from water by persulfate and ultraviolet concluded that the concentration of 1 mmol of sodium persulfate was the optimal concentration (30, 39).
Effect of pH on azithromycin removal efficiency
The reactions constant was obtained in K = -0.0008 pH + 0.1310, 0.135 min-1 at a pH = 5, 0.136 min-1 at pH = 7, 0.138 min-1 at pH = 9. This suggests that UV/persulfate process has similar efficiency in a wide spectrum pH (acidic, neutral and alkaline) which is one of the advantages of the UV/persulfate method. As it can be observed in the Figure 5. since there is no significant difference between the selected levels and all three diagrams are tangent to each other, the best and most economical level in three levels 5, 7, 9 is neutral (pH = 7) due to the lack of use of acid or alkali to adjust with the reactive environment. Irina Appalled et al. in a study conducted on levoflacxin by ferrous and persulfate, they also concluded that they have a better efficiency in near-neutral pH state (43). Similarly, Minghua Nie et al. the study on chloramphenicol antibiotics showed that the highest removal efficiency is obtained in the neutral pH state (44).
Effect of contact time on azithromycin removal efficiency
According to the Figure 3-5. Showing azithromycin removal efficiency versus time for different conditions of pH and initial concentration of azithromycin and persulfate, after exceeding 30 min, more than 90% of the removal process has been completed. Although this process improves over 90 minutes, the impact of and difference between these times are not so significant (PV > 0.05) to cause cost and time.
Xiaoli Zou et al. in a study on sulfadiazine removal efficacy found that at the time of 30-60 minutes the removal efficiencies are close to each other (45). In a study conducted by Shengnan Su et al. on amoxicillin removal using persulfate radicals, in the first 30 minutes, the process of eliminating antibiotics was 50% (22).
Azithromycin removal from real sample
Figure 6 presents the changes in azithromycin removal between contact times 30-180 min in a real sample and optimal conditions (i.e. pH = 7, contact time = 30 min., and persulfate concentration =1 mmol). The amount of removal is approximately constant at 70% and between 30-90 min and 10% there is an incremental process of 90-120 min in removal efficiency and again for 120 min the removal efficiency continues without any substantial change. The results showed that the UV/persulfate process can remove 69.44% of azithromycin in the wastewater in optimal conditions. As noted above, the highest removal efficiency occurred in the synthetic sample in 30 min. However, the removal efficiency in the real sample the same period is reduced by 30% due to the presence of other organic compounds in the real wastewater.
In order to obtain higher azithromycin removal efficacy in real conditions the period of the reaction stay in optimal condition was increased to 60, 90, 120, 150, and 180 min. As it can be observed in Figure 6 efficiency has increased to 70.92%, 71.54%, 83.22%, 85.35%, and 89.46%, respectively. With the increase in contact time, the most significant difference was observed at 120 min., which may be explained by the removal of other organic compounds by sulfate radicals within the time interval 30-90 min., and then the removal of the stable compound of the azithromycin antibiotic occurred which was maximized within a period of 2 h. The curve shows that although with increasing the time we can see that the increase in the removal efficiency was resulted, but this change in the efficiency is very gradual and there isn’t a significant difference between the removal percent at 120 min and those at 180 min. It is observed that there is a significant difference between the efficiency of antibiotic removal in the real wastewater samples and those in the synthetic wastewater samples in the same conditions in terms of the oxidation process. Perhaps the existence of some other anions and organic compounds in the real samples is the reason for this difference. Table 1 shows the effect of UV/persulfate process on the COD, phosphate, nitrate and bicarbonate removal in the real samples of hospital wastewater.
The nitrate in the wastewater is converted to the nitrate radicals by UV/persulfate process, and decreases with the efficiency 44%. Due to the creation of an internal filter, it reduces the amount of sulfate radicals and, as a result, reduces azithromycin removal efficiency compared to the time when the synthetic sewage without nitrates was analyzed (32). The conditions for the phosphate present in the sewage are in the same way, with the difference that more phosphate is affected by the superficial process, which is equal to 57%. The carbonates and bicarbonates in the sewage can also compete with antibiotics over the sulfate radicals. So that 100% of these anions are won and removed during the competition. The removal efficiency of organic compounds in the wastewater was obtained 66.74% (COD decrease from 415 to 138 mgL-1), which indicates oxidant demand of the organic compounds and decreasing the removal efficiency of azithromycin in the raw wastewater (Figure 7). The competition between the mentioned above compounds with azithromycin content can be shown as follows.
HCO3-> COD> PO43-> NO3-
Conclusions
The use of UV/persulfate process on a laboratory scale was a safe method to remove azithromycin. In this study, the effect of azithromycin concentration, persulfate concentration, pH and contact time on azithromycin removal efficiency was investigated. In the conducted experiments the azithromycin antibiotic concentration of 5 mgL-1, sodium peroxide concentration of 1 mmol, pH = 7 and the contact time of 30 min were obtained as optimal values. The ultraviolet radiation process removes 60.21% and UV/ sodium persulfate process removes 98.30% of azithromycin antibiotic in synthetic wastewater under the optimal conditions. This study proved that the idealization of the removal process can affect the efficiency of the system so that the removal efficiency in optimal conditions of the real wastewater was obtained as 70% and with the increase of the contact time to 3 h it approached to 90%. The study demonstrated the use of advanced UV/persulfate oxidation is a good way to remove azithromycin and similar antibiotics in the hospital and pharmaceutical plant’s wastewater. We propose more studies to apply this oxidation method and to determine the operation parameters for similar antibiotics in pilot and full scale in the future.
Acknowledgements
References
-
1.
Andreu V, Gimeno-García E, Pascual JA, Vazquez-Roig P, Picó Y. Presence of pharmaceuticals and heavy metals in the waters of a Mediterranean coastal wetland: Potential interactions and the influence of the environment. Sci. Total Environ. 2016;540:278-86. [PubMed ID: 26354171].
-
2.
Dirany A, Sirés I, Oturan N, Oturan MA. Electrochemical abatement of the antibiotic sulfamethoxazole from water. Chemosphere. 2010;81:594-602. [PubMed ID: 20833409].
-
3.
Gagnon C, Lajeunesse A, Cejka P, Gagné F, Hausler R. Degradation of selected acidic and neutral pharmaceutical products in a primary-treated wastewater by disinfection processes ozone. Sci. Eng. 2008;30:387-92.
-
4.
Batchu SR, Panditi VR, O′Shea KE and Gardinali PR. Photodegradation of antibiotics under simulated solar radiation: Implications for their environmental fate. Sci. Total. Environ. 2014;470–471:299-31.
-
5.
Ma Y, Li M, Wu M, Li Z, Liu X. Occurrences and regional distributions of 20 antibiotics in water bodies during groundwater recharge. Sci. Total. Environ. 2015;518–519:498-506.
-
6.
Berglund B, Khan GA, Weisner SE, Ehde PM, Fick J, Lindgren PE. Efficient removal of antibiotics in surface-flow constructed wetlands, with no observed impact on antibiotic resistance genes. Sci. Total. Environ. 2014;476–477:29-37.
-
7.
Lapworth DJ, Baran N, Stuart ME, Ward RS. Emerging organic contaminants in groundwater: A review of sources, fate and occurrence. Environ. Pollut. 2012;163:287-303. [PubMed ID: 22306910].
-
8.
Mandić Z, Weitner Z, Ilijas M. electrochemical oxidation of azithromycin and its derivatives. J. Pharm. Biomed. Anal. 2003;33:647-54. [PubMed ID: 14623590].
-
9.
Gao P, Mao D, Luo Y, Wang L, Xu B, Xu L. Occurrence of sulfonamide and tetracycline-resistant bacteria and resistance genes in aquaculture environment. Water Res. 2012;46:2355-64. [PubMed ID: 22377146].
-
10.
Luo Y, Guo W, Ngo HH, Nghiem LD, Hai FI, Zhang J, Liang S, Wang XC. A review of the occurrence of micropollutants in the aquatic environment and their fate and removal during wastewater treatment. Sci. Total. Environ. 2014;473–474:619-41.
-
11.
Feint K, Weston AA, Caminada D. Ecotoxicology of human pharmaceuticals. Aqua. Toxicol. 2006;76:122-59.
-
12.
Haddad T, Baginska E, Kümmerer K. Transformation products of antibiotic and cytostatic drugs in the aquatic cycle that result from effluent treatment and abiotic/biotic reactions in the environment: An increasing challenge calling for a higher emphasis on measures at the beginning of the pipe. Water Res. 2015;72:75-126. [PubMed ID: 25600206].
-
13.
Galvidis I, Lapa G, Burking M. Group determination of 14-membered macrolide antibiotics and azithromycin using antibodies against common epitopes. Anal. Biochem. 2015;46:75-82.
-
14.
Seifrtová M, Nováková L, Lino C, Pena A, Solich P. An overview of analytical methodologies for the determination of antibiotics in environmental waters. Anal. Chim. Acta. 2009;649:158-79. [PubMed ID: 19699391].
-
15.
Lotfipour F, Valizadeha H, Hallaj-Nezhadia S, Milania M, Zakeri-Milanib P. Comparison of Microbiological and High-Performance Liquid Chromatographic Methods for Determination of Clarithromycin Levels in Plasma. Iran. J. Pharm. Res. 2010;9:27-35. [PubMed ID: 24363703].
-
16.
Minghua N, Yi Y, Zhijian Z, Caixia Y, Xiaoning W, Hongjing LW. Degradation of chloramphenicol by thermally activated persulfate in aqueous solution. Chem. Eng. J. 2014;246:373-82.
-
17.
Ibáñez M, Guerrero C, Sancho JV, Hernández F. Screening of antibiotics in surface and wastewater samples by ultra-high-pressure liquid chromatography coupled to hybrid quadrupole time-of-flight mass spectrometry. J. Chromatogr. A. 2009;1216:2529-39. [PubMed ID: 19215931].
-
18.
Rickman KA, Mezyk SP. Kinetics and mechanisms of sulfate radical oxidation of β-lactam antibiotics in water. Chemosphere. 2010;81:359-65. [PubMed ID: 20701949].
-
19.
Homem V, Santos L. Degradation and removal methods of antibiotics for aqueous matrices – A review. J. Environ. Mngmt. 2011;92:2304-47.
-
20.
Antonopoulou M, Giannakas, A Deligiannakis Y, Konstantinou I. Kinetic and mechanistic investigation of photocatalytic degradation of the N, N-diethyl-m-toluamide. Chem. Eng. J. 2013;231:314-25.
-
21.
Mahdi-Ahmed M, Chiron S. Ciprofloxacin oxidation by UV-C activated peroxymonosulfate in wastewater. J. Hazard Mater. 2014;265:41-6. [PubMed ID: 24333713].
-
22.
Su S, Guo W, Yi C, Leng Y, Ma Z. Degradation of amoxicillin in aqueous solution using sulfate radicals under ultrasound irradiation. Ultraso Sonochem. 2012;19:469-74.
-
23.
Yan J, Lei M, Zhu L, Anjum MN, Zou J, Tang H. Degradation of sulfamonomethoxine with Fe3O4 magnetic nanoparticles as heterogeneous activator of persulfate. J. Hazard Mater. 2011;186:1398-404. [PubMed ID: 21237557].
-
24.
Ji Y, Ferronato C, Salvador A, Yang X, Chovelon JM. Degradation of ciprofloxacin and sulfamethoxazole by ferrous-activated persulfate: Implications for remediation of groundwater contaminated by antibiotics. Sci. Total. Environ. 2014;472:800-8. [PubMed ID: 24342085].
-
25.
Liu Y, He X, Fu Y, Dionysiou DD. Kinetics and mechanism investigation of the destruction of Oxytetracycline by UV-254 NM activation of persulfate. J. Hazard Mater. 2016;305:229-39. [PubMed ID: 26686482].
-
26.
He X, Mezyk SP, Michael I, Fatta-Kassinos D, Dionysiou DD. Degradation kinetics and mechanism of β-lactam antibiotics by the activation of H2O2 and Na2S2O8 under UV-254 NM irradiation. J. Hazard Mater. 2014;279:375-83. [PubMed ID: 25086235].
-
27.
He X, de la Cruz AA, O′Shea KE, Dionysiou DD. Kinetics and mechanisms of cylindrospermopsin destruction by sulfate radical-based advanced oxidation processes. Water Res. 2014;63:168-78. [PubMed ID: 25000199].
-
28.
He X, Cruz AA, de la, Dionysiou DD. Destruction of cyanobacterial toxin cylindrospermopsin by hydroxyl radicals and sulfate radicals using UV-254 NM activation of hydrogen peroxide, persulfate and peroxymonosulfate. J. Photoch. Photobiol. A: Chem. 2013;251:160-6.
-
29.
Shah NS, He X, Khan HM, Khan JA, O′Shea KE, Boccelli DL, Dionysiou DD. Efficient removal of endosulfan from aqueous solution by UV-C/peroxides: A comparative study. J. Hazard Mater. 2013;263:584-92. [PubMed ID: 24231332].
-
30.
Gao Y, Gao N, Deng Y, Yang Y, Ma Y. Ultraviolet (UV) light-activated persulfate oxidation of sulfamethazine in water. Chem. Eng. J. 2012;195–196:248-53.
-
31.
Ghari T, Kobarfard F, Mortazavi SA. Development of a Simple RP-HPLC-UV Method for Determination of Azithromycin in Bulk and Pharmaceutical Dosage forms as an Alternative to the USP Method. Iran. J. Pharm. R. 2013;12:57-63.
-
32.
Eslamizad S, Yazdanpanah H, Javidnia K, Sadeghi R, Bayat M, Shahanipour S, Khalighian N, Kobarfard F. Validation of an Analytical Method for Determination of Benzo[a]pyrene Bread using QuEChERS Method by GC-MS. Iran. J. Pharm. Res. 2016;15:465-74. [PubMed ID: 27642317].
-
33.
Sadeghi R, Yazdanpanah H, Kobarfard F, Eslamizad S, Bayat M. Simultaneous Determination of 13 Priority Polycyclic Aromatic Hydrocarbons in Tehran’s Tap Water and Water for Injection Samples Using Dispersive Liquid-Liquid Micro Extraction Method and Gas Chromatography-Mass Spectrometry. Iran. J. Pharm. Res. 2016;15:475-81. [PubMed ID: 27642318].
-
34.
Eslamizad S, Kobarfard F, Javidnia K, Sadeghi R, Bayat M, Shahanipour S, Khalighian N, Yazdanpanah H. Determination of Benzo[a]pyrene in Traditional, Industrial and Semi- industrial Breads Using a Modified QuEChERS Extraction, Dispersive SPE and GC-MS and Estimation of its Dietary Intake. Iran. J. Pharm. R. 2016;15:165-74.
-
35.
Sadeghi R, Kobarfard F, Yazdanpanah H, Eslamizad S, Bayat M. Validation of an Analytical Method for Determination of 13 priority polycyclic aromatic hydrocarbons in mineral water using dispersive liquid-liquid microextraction and GC-MS. Iran. J. P. R. 2016;15:157-68.
-
36.
Fernandez-Torres R, Consentino MO, Lopez MA, Mochon MC. Simultaneous determination of 11 antibiotics and their main metabolites from four different groups by reversed-phase high-performance liquid chromatography–diode array–fluorescence (HPLC–DAD–FLD) in human urine samples. Atlan. 2010;81:871-80.
-
37.
Yang ZY, Wang L, Tang X. Determination of azithromycin by ion-pair HPLC with UV detection. J. Pharm. Biomed. Anal. 2009;49:811-5. [PubMed ID: 19162426].
-
38.
APHA. WWA, and WEF. 22nd edition.
-
39.
Yin DD, Gao N, Li L, Deng Y. Degradation of florfenicol in water by UV/Na2S2O8 process. Environ Sci. Pollut Res. 2015;22:8693-701.
-
40.
Ahmed MM, Chiron S. Solar photo-Fenton like using persulphate for carbamazepine removal from domestic wastewater. Water Res. 2014;48:229-36. [PubMed ID: 24095595].
-
41.
Ghauch A, Ayoub G, Naim S. Degradation of sulfamethoxazole by persulfate assisted micrometric Fe0 in aqueous solution. Chem. Eng. J. 2013;228:1168-81.
-
42.
Michael K, Iacovou M, Frontistis Z, Hapeshi E, Dionysiou DD, Fatta-Kassinos D. Erythromycin oxidation and ERY-resistant Escherichia coli inactivation in urban wastewater by sulfate radical-based oxidation process under UV-C irradiation. Water. Res. 2015;85:346-58. [PubMed ID: 26360228].
-
43.
Appalled I, Trapido M, Dulova N. Degradation of levofloxacin in aqueous solutions by Fenton, ferrous ion-activated persulfate and combined Fenton/persulfate systems. Chem. Eng. J. 2015;279:452-62.
-
44.
Nie M, Yan C, Li M, Wang X, Bi W, Dong W. Degradation of chloramphenicol by persulfate activated by Fe2+ and zerovalent iron. Chem. Eng. J. 2015;279:507-15.
-
45.
Zou X, Zhou T, Mao J, Wu X. Synergistic degradation of antibiotic sulfadiazine in a heterogeneous ultrasound-enhanced Fe0/persulfate Fenton-like system. Chem. Eng. J. 2014;257:36-44.