Abstract
Keywords
Vitamin D3 Saccharomyces cerevisiae Food supplement Optimization Cholecalciferol
Introduction
Yeast enrichment refers to a method by which micronutrients and essential elements are increased in the yeast biomass (1). The baker’s yeast, Saccharomyces cerevisiae, is one of the popular eukaryotic microorganisms which have been recognized since ancient times (2, 3). Due to the short generation time, growing in minimal media and common environmental conditions and extraordinary fermentation properties, yeast cells are employed in the biotechnology and food industries (2, 4). S. cerevisiae has been introduced as a probiotic (5) for animals and human which has already indicated anti-inflammatory properties in induced colitis in mice (6) and ability in reducing digestive pain in patients with gastrointestinal disorders especially irritable bowel syndrome (IBS) (7).
Calciferol (vitamin D) is a family of fat soluble substances that increases intestinal absorption of magnesium, iron, phosphate, and zinc in vertebrates (8). Under the influence of solar UV-B radiation, this substance is synthesized from cholesterol in the dermal tissues (9). Nowadays, calciferol deficiency is distinguished as a pandemic. The main reason is inadequate sun exposure for most humans and another reason is lack of calciferol in foods (10). Calciferol deficiency causes some diseases in adults and children such as osteoporosis, osteopenia, fractures, and rickets (11). Several studies indicated that taking calciferol supplements had positive effects on pregnancy outcomes and decreased cancer mortality (12, 13).
Parameters employed in screening by the Plackett-Burman design
Parameters | Unit | Code | Low level (‒1) | High level (+1) |
---|---|---|---|---|
Temperature | °C | A | 25 | 40 |
Shaking speed | Rpm | B | 100 | 200 |
Initial cholecalciferol concentration | IU/ml | C | 100000 | 400000 |
Tryptone | g/l | D | 0.5 | 2.0 |
Added dextrose | % w/v | E | 2 | 10 |
Inoculation volume | Cfu | F | 103 | 107 |
Initial pH | - | G | 4.5 | 7.5 |
Sucrose | % w/v | H | 2 | 8 |
The Plackett-Burman screening design and responses*.
Coded parameters | Response | ||||||||||||
---|---|---|---|---|---|---|---|---|---|---|---|---|---|
Trial number | A | B | C | D | E | F | G | H | J | K | L | Cholecalciferol in dry cell weight (IU/g) | |
1 | 40.00 | 200.00 | 400000.00 | 0.5 | 2 | 103 | 7.50 | 2.00 | +1 | -1 | -1 | 1086.81 | |
2 | 40.00 | 100.00 | 400000.00 | 2 | 2 | 103 | 4.50 | 8.00 | +1 | +1 | -1 | 1450.21 | |
3 | 25.00 | 100.00 | 400000.00 | 0.5 | 10 | 107 | 7.50 | 2.00 | +1 | +1 | +1 | 1073.23 | |
4 | 25.00 | 200.00 | 100000.00 | 2 | 10 | 103 | 4.50 | 2.00 | +1 | -1 | +1 | 1285.23 | |
5 | 40.00 | 200.00 | 400000.00 | 2 | 10 | 107 | 4.50 | 2.00 | -1 | +1 | -1 | 1438.1 | |
6 | 25.00 | 100.00 | 400000.00 | 2 | 10 | 103 | 7.50 | 8.00 | -1 | -1 | +1 | 1467.32 | |
7 | 40.00 | 200.00 | 100000.00 | 0.5 | 10 | 103 | 7.50 | 8.00 | -1 | +1 | -1 | 1137.59 | |
8 | 40.00 | 100.00 | 100000.00 | 2 | 2 | 107 | 7.50 | 2.00 | -1 | -1 | +1 | 1050.33 | |
9 | 40.00 | 100.00 | 100000.00 | 0.5 | 10 | 107 | 4.50 | 8.00 | -1 | -1 | -1 | 837.49 | |
10 | 25.00 | 200.00 | 400000.00 | 0.5 | 2 | 107 | 4.50 | 8.00 | +1 | -1 | -1 | 1203.15 | |
11 | 25.00 | 100.00 | 100000.00 | 0.5 | 2 | 103 | 4.50 | 2.00 | +1 | +1 | +1 | 789.32 | |
12 | 25.00 | 200.00 | 100000.00 | 2 | 2 | 107 | 7.50 | 8.00 | -1 | +1 | +1 | 1437.07 | |
13 | 32.50 | 150.00 | 250000.00 | 1.25 | 6 | 5.0005×106 | 6.00 | 5.00 | 0 | 0 | 0 | 1127.54 | |
14 | 32.50 | 150.00 | 250000.00 | 1.25 | 6 | 5.0005×106 | 6.00 | 5.00 | 0 | 0 | 0 | 1149.53 | |
15 | 32.50 | 150.00 | 250000.00 | 1.25 | 6 | 5.0005×106 | 6.00 | 5.00 | 0 | 0 | 0 | 1129.17 | |
16 | 32.50 | 150.00 | 250000.00 | 1.25 | 6 | 5.0005×106 | 6.00 | 5.00 | 0 | 0 | 0 | 1132.34 | |
17 | 32.50 | 150.00 | 250000.00 | 1.25 | 6 | 5.0005×106 | 6.00 | 5.00 | 0 | 0 | 0 | 1123.02 |
The most significant parameters used in Box-Behnken experimental design
Parameters | Unit | Code | Low level (‒1) | Basal level (0) | High level (+1) |
---|---|---|---|---|---|
Initial cholecalciferol concentration | IU/ml | A | 100000 | 250000 | 400000 |
Tryptone | g/l | B | 0.5 | 1.25 | 2.0 |
Sucrose | %w/v | C | 2.0 | 5.0 | 8.0 |
Shaking speed | rpm | D | 100 | 150 | 200 |
Coefficient estimation of selected parameters on cholecalciferol uptake in dry cell weight of S. cerevisiae employing the Plackett-Burman design
Parameters | Symbol | Coefficient estimate | F-value | P-value Prob >F |
---|---|---|---|---|
Temperature | A | -11.74 | 9.80 | 0.0259 |
Shaking speed | B | 76.67 | 627.08 | < 0.0001 |
Initial cholecalciferol concentration | C | 88.99 | 563.16 | < 0.0001 |
Tryptone | D | 176.22 | 2208.32 | < 0.0001 |
Added dextrose | E | 28.00 | 55.76 | 0.0007 |
Inoculation volume | F | -5.27 | 1.97 | 0.2193 |
pH | G | 30.23 | 65.00 | 0.0005 |
Sucrose | H | 76.98 | 421.41 | < 0.0001 |
The Box-Behnken design and results of experiments.
Parameters | Response | ||||
---|---|---|---|---|---|
Run | A: Initial cholecalciferol | B: Tryptone (g/l) | C: Sucrose | D: Shaking | Cholecalciferol per |
1 | 250000.00 | 2.00 | 5.00 | 100.00 | 2629.61 |
2 | 250000.00 | 0.50 | 5.00 | 100.00 | 1321.96 |
3 | 250000.00 | 1.25 | 2.00 | 100.00 | 1144.07 |
4 | 100000.00 | 1.25 | 2.00 | 150.00 | 1566.53 |
5 | 250000.00 | 0.50 | 2.00 | 150.00 | 1228.04 |
6 | 250000.00 | 1.25 | 2.00 | 200.00 | 1405.95 |
7 | 250000.00 | 0.50 | 5.00 | 200.00 | 856.59 |
8 | 100000.00 | 0.50 | 5.00 | 150.00 | 1182.94 |
9 | 100000.00 | 2.00 | 5.00 | 150.00 | 1825.58 |
10 | 100000.00 | 1.25 | 5.00 | 100.00 | 1325.95 |
11 | 250000.00 | 1.25 | 5.00 | 150.00 | 3675.29 |
12 | 250000.00 | 1.25 | 5.00 | 150.00 | 3633.74 |
13 | 250000.00 | 1.25 | 5.00 | 150.00 | 3806.85 |
14 | 100000.00 | 1.25 | 8.00 | 150.00 | 1917.69 |
15 | 250000.00 | 2.00 | 5.00 | 200.00 | 1967.35 |
16 | 250000.00 | 0.50 | 8.00 | 150.00 | 1649.03 |
17 | 100000.00 | 1.25 | 5.00 | 200.00 | 785.331 |
18 | 400000.00 | 2.00 | 5.00 | 150.00 | 3880.96 |
19 | 400000.00 | 1.25 | 2.00 | 150.00 | 2263.93 |
20 | 250000.00 | 1.25 | 5.00 | 150.00 | 3629.65 |
21 | 400000.00 | 1.25 | 5.00 | 100.00 | 2380.49 |
22 | 250000.00 | 2.00 | 8.00 | 150.00 | 3753.4 |
23 | 400000.00 | 0.50 | 5.00 | 150.00 | 1775.68 |
24 | 400000.00 | 1.25 | 8.00 | 150.00 | 3625.97 |
25 | 250000.00 | 1.25 | 8.00 | 200.00 | 1659.39 |
26 | 250000.00 | 1.25 | 8.00 | 100.00 | 2751.53 |
27 | 250000.00 | 2.00 | 2.00 | 150.00 | 2234.16 |
28 | 250000.00 | 1.25 | 5.00 | 150.00 | 3580.94 |
29 | 400000.00 | 1.25 | 5.00 | 200.00 | 1921.96 |
Analysis of variance for the response surface modified quadratic model
Source | Sum of | Mean | F | P-value | ||
---|---|---|---|---|---|---|
Squares | df | Square | Value | Prob > F | ||
Model | 2.888×107 | 12 | 2.407×106 | 199.72 | < 0.0001 | significant |
A-initial cholecalciferol concentration | 4.374×106 | 1 | 4.374×106 | 363.01 | < 0.0001 | |
B-tryptone | 5.709×106 | 1 | 5.709×106 | 473.77 | < 0.0001 | |
C-sucrose | 2.534×106 | 1 | 2.534×106 | 210.29 | < 0.0001 | |
D-shaking speed | 7.287×105 | 1 | 7.287×105 | 60.47 | < 0.0001 | |
AB | 5.348×105 | 1 | 5.348×105 | 44.39 | < 0.0001 | |
AC | 2.555×105 | 1 | 2.555×105 | 21.20 | 0.0003 | |
BC | 3.015×105 | 1 | 3.015×105 | 25.02 | 0.0001 | |
CD | 4.583×105 | 1 | 4.583×105 | 38.04 | < 0.0001 | |
A2 | 3.519×106 | 1 | 3.519×106 | 292.08 | < 0.0001 | |
B2 | 3.698×106 | 1 | 3.698×106 | 306.91 | < 0.0001 | |
C2 | 2.684×106 | 1 | 2.684×106 | 222.75 | < 0.0001 | |
D2 | 1.054×107 | 1 | 1.054×107 | 874.35 | < 0.0001 | |
Residual | 1.928×105 | 16 | 12049.74 | |||
Lack of Fit | 1.633×105 | 12 | 13606.37 | 1.84 | 0.2921 | not significant |
Pure Error | 29519.28 | 4 | 7379.82 | |||
Cor. Total | 2.907×107 | 28 | ||||
R2 | 0.9934 | |||||
R2adj | 0.9884 | |||||
C.V.% | 4.87 | |||||
Adeq precision | 42.630 |
Validity of the model in optimal conditions
Response | Software prediction Value (IU/g) | Validity experiment value (IU/g) | 95% CI low | 95% CI high |
---|---|---|---|---|
Cholecalciferol per dry cell weight | 4332.105 | 4428.11 | 4055.44 | 4608.77 |
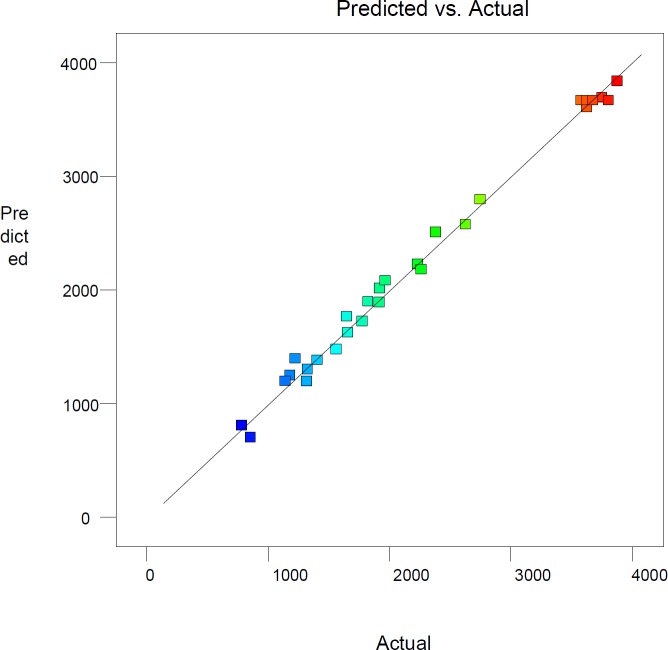
.
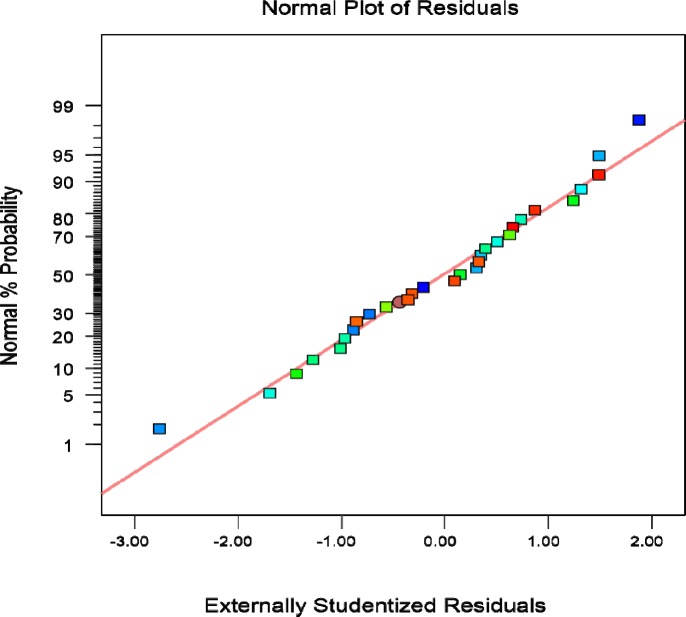
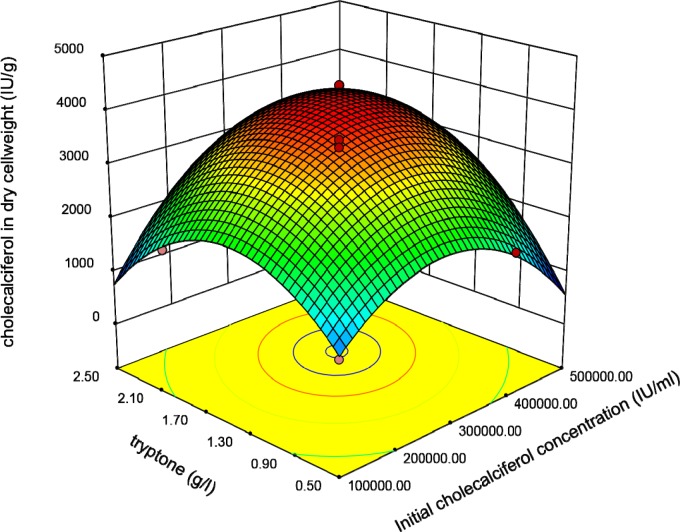
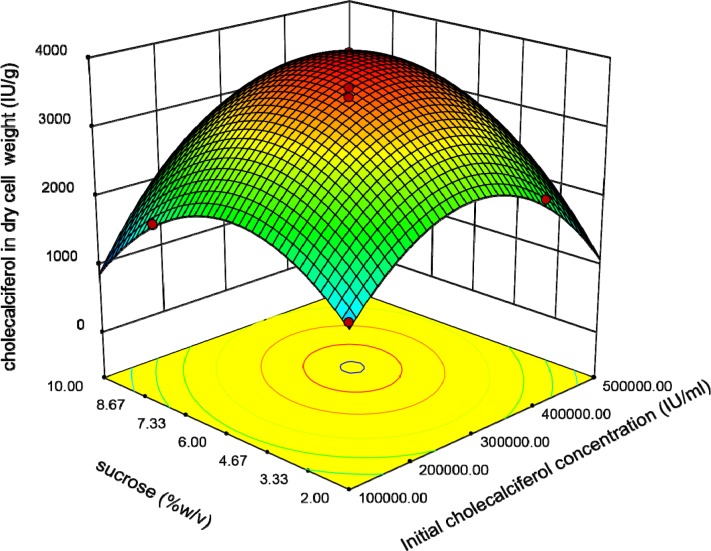
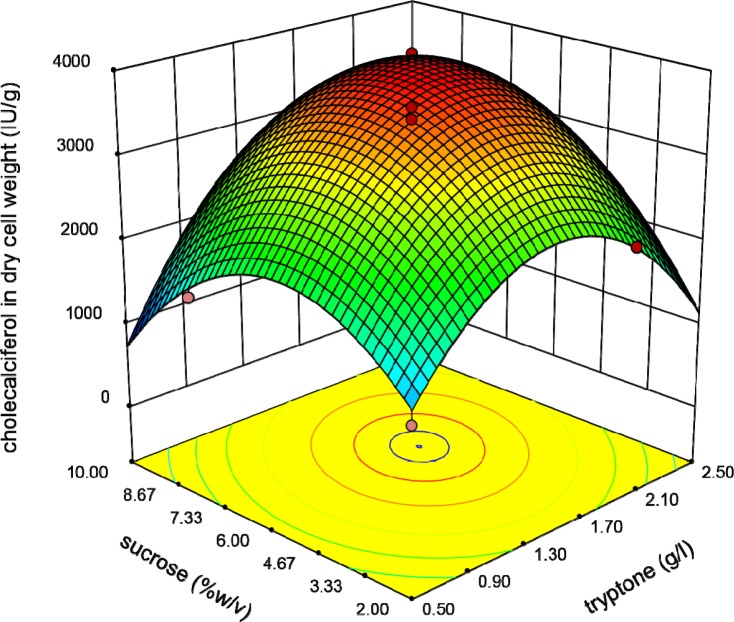
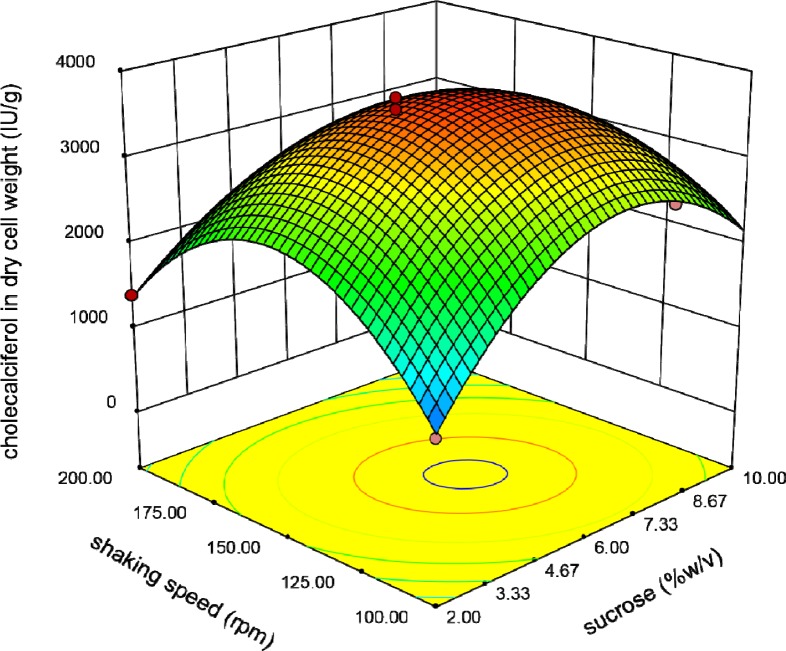
Calciferol exists in several forms. Vitamin D2 (ergocalciferol) and vitamin D3 (cholecalciferol) are two main forms in human derived from ergosterol and 7-dehydrocholesterol, respectively (14). In a recent study, S. cerevisiae was treated with UV-B irradiation to induce alteration of yeast sterol to ergocalciferol for using as food supplement (15). While, some new investigations focused on ergocalciferol disadvantages for human and animals (16-19). Hohmann et al. indicated that the rats fed with ergocalciferol-enriched yeasts had lower levels of 25-hydroxy vitamin D in comparison with the rats fed with cholecalciferol (20). Nieman et al. showed that ergocalciferol supplementation reinforced abnormal exercise-induced muscle damage in some athletes (16). Tripkovic et al. showed that supplementation with cholecalciferol was more effective in increasing serum 25-hydroxy calciferol concentrations than ergocalciferol (17). Therefore, it seems that cholecalciferol supplementation has more beneficial effects for human health than ergocalciferol supplementation.
Yeast S. cerevisiae has the ability to uptake and accumulate some micronutrients from enriched liquid media by physicochemical or metabolism-dependent reactions (21). There are some studies which have used S. cerevisiae as a transporter for some essential trace metals which resulted in increased bioavailability and safety of micronutrients such as iron, copper, zinc, and manganese (1, 22-24). Based on previous findings, we hypothesized that combination of yeast and cholecalciferol probably have several advantages including designing an enriched probiotic supplement, increasing half-life of vitamin by protecting against destructor agents in digestive system and sustained release of vitamin into the digestive tract for better gastrointestinal absorption. In this study S. cerevisiae was employed as a cholecalciferol accumulator for the first time. By using Plackett-Burman screening design, four parameters were selected for assessment of optimal conditions for enrichment of S. cerevisiae biomass with cholecalciferol and were optimized by using response surface methodology.
Experimental
Microorganism, culture condition and chemicals
The yeast S. cerevisiae ATCC 9763 was maintained on Sabouraud Dextrose agar (SDA, Merck Co. Darmstadt, Germany) and stored at 4 °C. The cholecalciferol used for enrichment of S. cerevisiae was purchased from DSM Co. Basel, Switzerland. The other chemicals were purchased from Merck Co. Darmstadt, Germany.
Fermentation conditions
Sabouraud dextrose broth (SDB) medium in shake flasks was employed for optimization of culture condition. The SDB composition, that was purchased from Merck Co., (g/L) consisted of peptone from meat, 5.0; peptone from casein, 5.0; and glucose, 20.0. A 24-h culture of S. cerevisiae grown in SDB medium at 30 °C was used as pre-culture. The 500-ml Erlenmeyer flasks containing 200 ml of SDB were inoculated with 5% (v/v) of pre-culture to reach to an initial yeast cell concentration of about 0.1 g/L. Vitamin D3 (cholecalciferol) was added separately to the culture media at initial concentrations ranging from 100000-400000 IU/mL. The flasks were maintained in a shaker incubator (J Labtech, Daihan Labtech Co.) at 100-200 rpm in dark condition. The samples were taken at regular time intervals and tested for dry cell weight (g/L), and cholecalciferol uptake (IU/g).
Dry cell weight determination
For determination of dry cell weight, the yeast cells were isolated from SDB culture media by centrifugation (4000 ×g, 10 min) and washed twice with deionized water. The pellets were dried at 50°C under vacuum and weighed for measurement of dry cell weight.
Measurement of cholecalciferol uptake
Extraction of cholecalciferol
Extraction of cholecalciferol was carried out according to the modified method of Bligh and Dyer (25). The SDB culture medium was centrifuged for 10 min at 4000×g and the pellets were washed twice with PBS. Then, about 0.5 g of the pellets was added to 30 mL of methanol. The suspension was maintained at 25 °C and dark condition for 16 h and was sonicated in an ultrasonic bath (Q Sonica, Q700) for 20 min. The methanol was evaporated at 45 °C under nitrogen steam. Then, 10 mL of n-hexan was added to the residue and sonicated again for 15 min. The solvent was evaporated and the residue was dissolved in 1 mL of methanol and filtered before HPLC analysis.
HPLC conditions
HPLC was carried out with a Waters (US) 2690 system, a tunable absorbance UV-detector 486 (Waters, US) with the range of 190–800 nm, an E-600 binary pump and an auto sampler. Analyses were carried out with Millennium Software (Waters Tech.). Stock solution of standard cholecalciferol (1 mg/mL) was prepared in methanol, filtered through a 0.45 μm filter, and stored at -20 ˚C. The internal standard solution was prepared by diluting the stock solution with the mobile phase.
Cholecalciferol was separated on a Machery Nagel C18 column, 25 cm, 4.6 mm, and 5 μm. The column temperature ranged from 30 to 45 °C. Isocratic elution of mobile phase consisted of 60% methanol, 28% iso-propanol, and 12% deionized water with a flow-rate of 1 mL/min. Total run time was 22 min and the intended peak areas were integrated at 265 nm. The amount of cholecalciferol uptake was expressed as international unit (IU) per gram of dry S. cerevisiae cells.
Design of experiments
Optimization of culture conditions for enrichment of S. cerevisiae with cholecalciferol were carried out in three steps. In the first step, the variables were selected from previous studies. One variable-at-a-time trials were accomplished to find the range of quantitative factors and selection of appropriate qualitative parameters before screening studies. In the second step, the significant parameters were determined by Plackett-Burman screening design. Finally, in the third step, optimal levels of these parameters were evaluated by Box-Behnken technique.
Screening Study
Selection of the most principal parameters among the several factors was performed by Plackett-Burman design (PBD) (26). By this technique, n factors in n + 1 experiment could be studied, where n is a multiple of four. Because of orthogonality in PBD, the parameters can be evaluated alone without considering their interactions.
In Table 1, eight parameters employed in the screening by PBD including temperature, shaking speed, initial concentration of cholecalciferol, inoculation volume, initial pH, Tryptone, sucrose, and dextrose (the concentration which has been added to the SDB medium) concentrations are illustrated. The codes +1 (high level) and-1 (low level) show the two different levels of the independent parameters. In PBD, the interactions between variables were omitted; therefore, a first order equation explains the model:
Y= α0 +Ʃ α i xi (i=1,…, k)
where Y is the cholecalciferol uptake in yeast biomass (response), α0 is a constant value and α i is the regression slope.
Table 2 represents the Plackett-Burman matrix with 8 factors in 12 experiments. The symbols, (+) and (‒) show high and low levels of variables, respectively. This matrix designed by the statistical software Design-Expert® version 10.0.1, Stat-Ease, Inc., Minneapolis, USA. In addition to the eight main parameters, there are three dummy factors in this matrix. Existence of dummy variables up to one-third of all parameters is helpful to estimate the random trial errors (27).
Optimization Design
The main response of this study, "cholecalciferol uptake in dry cell weight of S. cerevisiae", was optimized by response surface methodology (28). The optimal levels of variables whose effects were found significant in the screening studies, were investigated by Box-Behnken experimental design (29) using Design-Expert® software version 10.0.1, Stat-Ease, Inc., Minneapolis, USA. In Table 3, the significant variables including initial cholecalciferol concentration (A), concentration of Tryptone (B), concentration of sucrose (C) and shaking speed (D) were defined at three levels of low, basal, and high which indicated ‒1, 0 and +1, respectively. For investigation of four mentioned variables, the Box-Behnken design with a set of twenty-nine trials with five central point replicates was performed. The central point trials were conducted for determination of pure error.
A modified quadratic polynomial equation that is shown below, was the most appropriate model for description of statistical behaviors of this system:
Y=α0+∑αiXi+∑αiiXi2+∑ αijXiXj+ɛ
where Y is the expected response, α0 is a constant value, αi and αii indicate linear and quadratic influences respectively, αij is the quadratic influence of the interactions, Xi and Xj are value of the effective variables on response and ε is the error of trials. In this investigation, Y is the cholecalciferol uptake in yeast biomass.
Statistical assessments such as analysis of variance, multiple correlation coefficient (R2), adjusted R2, Adequate precision and relative standard deviation (C.V.%) were assayed by Design-Expert® software version 10.0.1 Stat-Ease, Inc., Minneapolis, USA. The significance of the modified quadratic polynomial model was determined by P value test. In this investigation, response surface plot represented the influence of parameters and their interactions on principal response.
Plot of studentized residual versus predicted response and plot of trial versus predicted response values were analyzed.
Results and Discussion
Plackett-Burman design (PBD) assessment
Primary studies were accomplished to find the range of quantitative factors and selection of appropriate qualitative parameters (data not shown). Then, PBD was used for selection of the most principal parameters affecting cholecalciferol uptake in S. cerevisiae biomass. Statistical investigations by F test illustrated that among the parameters tested by PBD, shaking speed (B), initial concentration of cholecalciferol (C), Tryptone (D), and sucrose concentrations (H) had significant effects on the main response than the other parameters (Table 4).
These four significant parameters had positive sign in estimate coefficient. Thus, rising their values causes increasing the cholecalciferol uptake in S. cerevisiae biomass.
More statistical assessments represented that differences between the means of central points and factorial experiments in PBD were not significant (P > 0.05). This illustrated that optimum levels for the cholecalciferol entrapment in the yeast biomass would be near the experimental ranges selected for PBD and there was no need for steepest ascend methods.
The R2 parameter of this design is approximately 98.14%, which indicates that the model is corresponding with 98.14% of the actual responses. Adjusted R2 is about 95.05%. The inconsistency between predicted R2 and adjusted R2 complains that the first order equation is not efficient for model description.
Box-Behnken design and trial responses
In Box-Behnken design, trial responses, quadratic effect of each parameter and their interactions were estimated statistically. By using this experimental design, each variable was changed at low, basal and high levels when the others were kept stable. The Box-Behnken design and results of experiments are indicated in Table 5.
Response surface methodology (RSM) analysis
Results gained from the design of experiments revealed that the range of cholecalciferol entrapment in yeast biomass was about 785.33-3380.96 IU/g. Analysis of variance (ANOVA) of the results was done to pick out the appropriate model that fit the responses. The most relevant model has a modified second-order (quadratic) polynomial equation. As shown in Table 6, the model was significant which was approved by calculating P-value (P < 0.0001). The model acceptability was shown with lack of fit F-value of 0.292 which proposed that the lack of fit is not significant.
The R2 coefficient of this model is 0.993, which indicates that the model is corresponding with about 99% of the actual responses. Adjusted R2 coefficient is about 0.988. These parameters illustrate that the noise of the system is less than one percent. The predicted R2 of 0.975 reveals an appropriate compatibility between the value anticipated by the modified quadratic polynomial model and the trial responses. Plot of experimental versus predicted values is one of the current alternatives to assess model predictions. The straight line in this plot shows full agreement between experimental and estimated values (Figure 1).
The C.V% of 4.87 shows repeatability and integrity of the model. Adeq precision ratio of 42.630 shows an adequate signal. This parameter determines the signal to noise ratio. A ratio of more than 4 is acceptable which was seen in this model.
Plot of studentized residual versus the value predicted by the modified quadratic model presents that deviation in the responses is acceptable and no outlier was seen in trial results (Figure 2).
The first-order, second-order and interaction coefficients of quadratic polynomial model were checked via software. Despite of interaction between initial concentration of cholecalciferol and shaking speed (AD) and interaction between Tryptone concentration and shaking speed (BD) which were insignificant (P > 0.05), other linear, squared, and interaction coefficients were significant (P < 0.05) (Table 6).
Thus, the full quadratic polynomial equation was not appropriate for model description and the modified quadratic polynomial model was employed for optimization.
The system equation that Design-Expert software® proposed is as follows:
Y = 3665.29 + 603.75 A + 689.73 B + 459.53 C – 246.42 D + 365.66 AB + 252.72 AC + 274.56 BC – 338.50 CD – 736.61 A2 – 755.07 B2 – 643.27 C2 – 1274.46 D2
where Y is the cholecalciferol uptake in dry cell weight of yeast cells. A, B, C and D are initial concentration of cholecalciferol in culture medium, concentration of Tryptone , concentration of sucrose and shaking speed, respectively. In this equation, linear coefficients of A, B, and C and interaction coefficients of AB, AC, and BC have positive sign that indicate their positive influences on the main response. Other interactions and second-order coefficients of parameters and shaking speed (D) have negative sign which could reduce the main response. All interactions except interactions between concentration of cholecalciferol and shaking speed and between Tryptone concentration and shaking speed were significant.
Interaction between initial cholecalciferol concentration and Tryptone concentration (AB) is the most significant in comparison with the other interactions. The importance of each interaction on the final response is dependent on its significance in this pattern: AB>CD>BC>AC.
Three-dimentional response plots
Graphical plots especially three-dimensional response surface plots are appropriate facilities for assessment of variables. The interaction between initial concentration of cholecalciferol and Tryptone when other parameters (sucrose and shaking speed) were constant at the basal value is shown in Figure 3.
This plot reveals that the interaction coefficients of these parameters are significant. The peaked shape of the plot indicates that we could estimate the optimal level of the cholecalciferol entrapped in the yeast biomass in the range of variables. Figure 4 indicates that when the initial cholecalciferol and sucrose concentrations raised, the cholecalciferol uptake in S. cerevisiae biomass increased to an optimal point. The shape of plot reveals that the interaction between initial cholecalciferol and sucrose concentrations is significant too.
The relationship between Tryptone and sucrose concentrations is shown in Figure 5. It shows that raising these variables resulted in increasing of the response to an optimal level.
Finally, Figure 6 reveals the interaction between shaking speed and sucrose concentration. In all of these Figures, the shape of three-dimensional plots is convex which means positive effects of the analyzed interactions on cholecalciferol entrapment in yeast biomass. Existence of optimal levels in the range of variables confirmed the suitability of the model.
Optimal point
According to the results obtained from the experiments, optimum levels of the variables to maximize the main response were cholecalciferol initial concentration of 358021.16 IU/mL, Tryptone concentration of 1.82 g/l, sucrose concentration of 7.13 % (w/v) and shaking speed of 140.46 rpm. The maximum amount of cholecalciferol in dry cell weight of S. cerevisiae when the other variables were considered constant was anticipated to be 4332.105 IU/g.
Validity experiment
To assess the validity of the optimal points obtained via the model, a trial was accomplished with the parameters proposed by the software. In this experiment, 4428.11 international unit of cholecalciferol was obtained from each gram of dried cells; which was 97.83% of the value calculated by the modified second-order model (Table 7).
The observed value is highly congruent with the software value which confirms the accuracy and reliability of the model. In this study, the most important factors that showed significant effects on the enrichment of S. cerevisiae with cholecalciferol were carbon source, nitrogen source, and shaking speed which are similar with the results of previous investigations about enrichment of S. cerevisiae by metallic elements. They reported nitrogen and carbon sources, shaking speed, temperature, and pH as the most significant variables (1, 22, 24). Although there are several studies about enrichment of yeast biomass by metallic elements, this study is the first report about entrapment of fat soluble components in the yeast cells.
Conclusion
This investigation is the first study that employed the probiotic yeast, S. cerevisiae, as an accumulator of cholecalciferol. Combination of yeast and cholecalciferol probably have several advantages including designing an enriched probiotic supplement, increasing half-life of cholecalciferol by protecting against destructor agents in digestive system and sustained release of cholecalciferol into the digestive tract for better gastrointestinal absorption. PBD studies showed that cholecalciferol absorption in yeast cells was affected by initial cholecalciferol concentration, Tryptone concentration, sucrose concentration, and shaking speed, significantly. Box-Behnken designs indicated that the reliable model which fitted to responses was a modified quadratic polynomial equation. By using RSM, cholecalciferol entrapment in yeast biomass increased from 2379.81 IU/g in SDB medium to 4428.11 IU/g in optimized medium (about 2-folds increase).
References
-
1.
Stehlik-Tomas V, Zetić VG, Stanzer D, Grba S, Vahčić N. Zinc, copper and manganese enrichment in yeast Saccharomyces cerevisiae. Food Technol. Biotechnol. 2004;42:115-20.
-
2.
Volkov V. Quantitative description of ion transport via plasma membrane of yeast and small cells. Front. Plant Sci. 2015;6:425. [PubMed ID: 26113853].
-
3.
Lodolo EJ, Kock JLF, Axcell BC, Brooks M. The yeast Saccharomyces cerevisiae: the main character in beer brewing. FEMS Yeast Res. 2008;8:1018-36. [PubMed ID: 18795959].
-
4.
Moslehi-Jenabian S, Pedersen LL, Jespersen L. Beneficial effects of probiotic and food borne yeasts on human health. Nutrients. 2010;2:449-73. [PubMed ID: 22254033].
-
5.
Fazeli MR, Bahmani S, Jamalifar H, Samadi N. Effect of probiotication on antioxidant and antibacterial activities of pomegranate juices from sour and sweet cultivars. Nat. Prod. Res. 2011;25:288-97. [PubMed ID: 21294041].
-
6.
Foligne´ B, Dewulf J, Vandekerckove P, Pigne`de G, Pot B. Probiotic yeasts: anti-inflammatory potential of various nonpathogenic strains in experimental colitis in mice. World J. Gastroenterol. 2010;16:2134-45. [PubMed ID: 20440854].
-
7.
-
8.
Holick MF. High prevalence of vitamin D inadequacy and implications for health. Mayo. Clin. Proc. 2006;81:353-73. [PubMed ID: 16529140].
-
9.
Wacker M, Holick MF. Sunlight and Vitamin D: A global perspective for health. Dermatoendocrinology. 2013;5:51-108.
-
10.
Zaidi SAH, Singh G, Owojori O, Kela R, Spoors S, Abbas M, Barton F, Rogers C. Vitamin D Deficiency in Medical Inpatients: A Retrospective Study of Implications of Untreated Versus Treated Deficiency. Nutr. Metab. Insights. 2016;9:65-9. [PubMed ID: 27688711].
-
11.
Sahay M, Sahay R. Rickets–vitamin D deficiency and dependency. Indian J. Endocrinol. Metab. 2012;16:164-76. [PubMed ID: 22470851].
-
12.
De-Regil LM, Palacios C, Ansary A, Kulier R, Peña-Rosas JP. Vitamin D supplementation for women during pregnancy. Cochrane database Syst. Rev. 2012;2:CD008873.
-
13.
Bjelakovic G, Gluud LL, Nikolova D, Whitfield K, Krstic G, Wetterslev J, Gluud C. Vitamin D supplementation for prevention of cancer in adults. Cochrane Database of Syst. Rev. 2014;6:CD007469.
-
14.
Bouillon R, Verlinden L, Verstuyf A. Is Vitamin D2 Really Bioequivalent to Vitamin D3? Endocrinology. 2016;157:3384-7. [PubMed ID: 27580803].
-
15.
Scientific Opinion on the safety of vitamin D-enriched UV-treated baker’s yeast. EFSA J. 2014;12:3520.
-
16.
Nieman DC, Gillitt ND, Shanely RA, Dew D, Meaney MP, Luo B. Vitamin D2 Supplementation Amplifies Eccentric Exercise-Induced Muscle Damage in NASCAR Pit Crew Athletes. Nutrients. 2014;6:63-75.
-
17.
Tripkovic L, Lambert H, Hart K, Smith CP, Bucca G, Penson S, Chope G, Hyppöne E, Berry J, Vieth R, Lanham-New S. Comparison of vitamin D2 and vitamin D3 supplementation in raising serum 25-hydroxyvitamin D status: a systematic review and meta-analysis. American J. Clin. Nutr. 2012;95:1357-64.
-
18.
Logan VF, Gray AR, Peddie MC, Harper MJ, Houghton LA. Long-term vitamin D3 supplementation is more effective than vitamin D2 in maintaining serum 25-hydroxyvitamin D status over the winter months. Briti. J. Nutr. 2013;109:1082-8.
-
19.
Houghton LA, Vieth R. The case against ergocalciferol (vitamin D2) as a vitamin supplement. American J. Clin. Nutr. 2006;84:694-7.
-
20.
Hohman EE, Martin BR, Lachcik PJ, Gordon DT, Fleet JC, Weaver CM. Bioavailability and Efficacy of Vitamin D2 from UV-Irradiated Yeast in Growing, Vitamin D-Deficient Rats. J. Agric. Food Chem. 2011;59:2341-6. [PubMed ID: 21332187].
-
21.
Azad SK, Shariatmadari F, Torshizi MAK. Production of zinc-enriched biomass of Saccharomycescerevisiae. J. Elem. 2014;19:313-26.
-
22.
Gaensly F, Wille GMFC, Brand D, Bonfim TMB. Iron enriched Saccharomyces cerevisiae maintains its fermenting power and bakery properties. Ciênc. Tecnol. Aliment. 2011;31:980-3.
-
23.
Kaur T, Bansal MP. Selenium enrichment and anti-oxidant status in baker’s yeast, Saccharomyces cerevisiae at different sodium selenite concentrations. Nutr. Hosp. 2006;21:704-8. [PubMed ID: 17147070].
-
24.
Esmaeili S, Khosravani-Darani K, Pourahmad R, Komeili R. An Experimental Design for Production of Selenium-Enriched Yeast. World Appl. Sci. J. 2012;19:31-7.
-
25.
Bligh EG, Dyer WJ. A rapid method of total lipid extraction and purification. Canadian J. Biochem. Physiol. 1959;37:911-7.
-
26.
Tasharrofi N, Adrangi S, Fazeli M, Rastegar H, Khoshayand MR, Faramarzi MA. Optimization of chitinase production by Bacillus pumilus using Plackett-Burman design and response surface methodology. Iran. J. Pharm. Res. 2011;10:759-68. [PubMed ID: 24250411].
-
27.
Desai KM, Akolkar SK, Badhe YP, Tambe SS, Lele SS. Optimization of fermentation media for exopolysaccharide production from Lactobacillus plantarum using artificial intelligence-based techniques. Process Biochem. 2006;41:1842-8.
-
28.
Ashengroph M, Nahvi I, Amini J. Application of Taguchi design and response surface methodology for improving conversion of isoeugenol into vanillin by resting cells of Psychrobacter sp. CSW4. Iran. J. Pharm. Res. 2013;12:411-21. [PubMed ID: 24250648].
-
29.
Box GEP, Behnken DW. Some new three level designs for the study of quantitative variables. Technometrics. 1960;2:455-75.