Abstract
Keywords
Epilepsy Pentylenetetrazol Nutmeg extract Neuroprotection Glial activation
Introduction
Abnormal electrical activity in epileptic seizures results from an imbalance between excitation and inhibition (1). In addition, inflammatory processes have an important role in the pathogenesis of epilepsy (2). Experimental and clinical evidences have suggested that pro-inflammatory factors and cytokines are overexpressed in epileptic conditions and these factors can enhance brain excitability and alter microglia and astrocyte functioning (3). Neuro-inflammation results primarily from the activation of glial cells including astrocytes and microglia. Activated glial cells secrete variety of inflammatory cytokines (3-5). These inflammatory cytokines play an important role in the progression of epilepsy (2). Previous studies indicated that some anti-inflammatory drugs reduce seizure behavior in experimental models and clinical cases of epilepsy (2, 6, 7). Despite the availability of several anti-epileptic drugs, these medications are effective only in about 40% of epileptic patients (8). In addition, anti-epileptic drugs have different side effects (9). Based on these limitations, a demand for finding new types of anticonvulsant drugs exist. Recent studies have demonstrated that herbal medicines (phytomedicine) might be effective in the treatment of some epileptic cases (10). Nutmeg (Myristica fragrans Houtt.) is widely being used as a spice in the food industry worldwide. Nutmeg, or its effective substances, have been shown to possess beneficial effects in the treatment of several diseases such as rheumatoid arthritis, diarrhea, mouth sore, and insomnia (11). Numerous evidences have demonstrated that nutmeg has several pharmacological functions such as anti-bacterial, anti-microbial, anti-fungal (12), and antioxidant effects (13). Additionally, the anti-inflammatory effect of nutmeg has been evaluated both in-vitro and in-vivo (14-16). It has been shown that nutmeg, or its active compounds, effectively alleviate inflammation by inhibiting various inflammatory factors (14-16). The pentylentetrazol (PTZ)-induced kindling model is one of the most reliable models for studying the epileptogenesis process. Repetitive administration of PTZ at sub-convulsive dose leads to the induction of chemical kindling model in animals. The effects of novel anti-epileptic compounds can be evaluated by either applying them before the initiation of kindling (pre-kindling phase) or after becoming fully-kindled (post-kindling phase) (17).
The present study was designed to elucidate the effects of nutmeg extract on the development of kindling and neuronal death following PTZ administration, based on the role of inflammation on epilepsy and the anti-inflammatory properties of nutmeg extract. In addition, glial activation was evaluated in animals under nutmeg extract treatment.
Experimental
Preparation of nutmeg extract
Nutmeg seeds purchased from our local market in Babol and approved by the department of Pharmacology, Babol University of Medical Sciences (herbarium number: 94/301). Preparation of extract was performed based on our previous report (18). In brief, 300 grams of nutmeg seeds was powdered with a mechanical grinder. After addition of 500 mL absolute ethanol, the powder was shaken for 72 h. The supernatant solvent was removed using a rotary evaporator (in a vacuum, temperature below 45 °C). To completely evaporate the solvent, the prepared extract was placed into a plate in the oven.
Gas chromatography-mass spectrometry analysis of nutmeg extract
Nutmeg extract was analyzed using Agilent 7890B gas chromatograph (Agilent Technologies, Palo Alto, CA, USA) equipped with 5977B mass spectrometer. Helium gas, with a flow rate of 1 mL/min, was used as the carrier gas. Volatile components were separated by an HP-5MS capillary column (polydimethylsiloxane5% diphenyl, 30 m×0.32 mm×0.25 mm, Hewlett Packard). The temperature was set to rise from 40 °C (1 min) to 220 °C at 5 °C/min, with a 1 min hold at the end. The compounds were identified by comparing their mass spectra and elution order with those obtained from the National Institute of Standards and Technology (NIST 08) and Wiley 7n library data of the GC-MS system.
Animals
Male NMRI mice (20-30 g) were obtained from the animal house of Babol University of Medical Sciences (Babol, Iran). Animals were kept in 12 h light/dark cycle with free access to food and water. All experimental procedures were approved by the local ethical committee of Babol University of Medical Sciences, which is in according to international guidelines on the use of laboratory animals.
PTZ-induced kindling model
Chemical kindling model was induced as described in our previous study (19). Briefly, PTZ was administrated intraperitoneally (i.p) at dose of 36.5 mg/kg every 48 h for 18 consecutive days. Animals were monitored after each PTZ injection for 20 min and seizure behavior was scored as follows: Stage 0, no response; Stage 1, ear and facial twitching; Stage 2, myoclonic jerks (MJ), Stage 3, clonic forelimb convulsions; Stage 4, generalized clonic seizures along with turning to a side position, and Stage 5, generalized clonic-tonic seizures (GCTS) or death (20). In this study, latency to the onset of MJ (S2 latency), duration of GCTS (S4 & S5 duration) and maximum seizure stage were assessed after each PTZ injection.
Experiment design
Forty-two male NMRI mice were randomly divided to 6 experimental groups (n = 7) as follows: Saline +PTZ, which received i.p. injections of sterile saline containing 0.1% Tween-80 as nutmeg extract vehicle, 1 h before each PTZ injection; nutmeg + PTZ group, where nutmeg extract at 50 or 100 mg/kg doses was administrated i.p. 1 h before each PTZ injection. In another approach, animals received nutmeg extract pre-treatment for 1 week and continued as shots 1 h before each PTZ injection. Groups had the same amount of subjects as the first approach. Animals were sacrificed after evaluating seizure behavior and electrophysiological recordings, and the level of glial activation and neuronal density were assessed using immunostaining and nissl staining, respectively.
Electroencephalogram recording
After the 6th PTZ injection, electroencephalogram recordings were carried out based on a previous report by Gol et al., (21). Animals were anesthetized with ketamine (100 mg/kg) and xylazine (10 mg/kg) and fixed in a stereotaxic apparatus (Stoleting, USA). Two monopolar electrodes were implanted into the frontal and parietal lobes of the cortex and fixed with dental cement. Electroencephalogram (EEG) recording was performed in freely moving animals for 10 min after the last injection of PTZ (injection 9). EEG signal was band pass filtered 1-100 Hz and amplified 500 times (D3111 Data Acquisition, ScienceBeam Co., Tehran, Iran).
Immunostaining
After deep anesthesia with ketamine (70 mg/kg) and xylazine (10 mg/kg), animals were transcardially perfused with phosphate buffer saline (PBS) and paraformaldehyde (PFA) 4%. Brain samples were removed and submerged in PFA for 12-16 h at 4 °C. Samples were dehydrated using 30 % sucrose. Brain tissues were embedded in optimal cutting temperature (OCT) compound and coronal sections (6 µm) from the dorsal part of the hippocampus (bregma from -1.46 mm to -2.30 mm) with 120 µm intervals were prepared using cryostat instrument (MICROM HM 525, Thermo Scientific). Immunostaining was performed according to our previous report (20). Briefly, after washing with PBS, sections were permeablized by Triton X100 0.3 % for 20 min. None-specific bindings were blocked using blocking solution (normal goat serum (NGS) 10% in PBS) for 1 h. Then, sections were incubated with primary antibodies including rabbit anti-GFAP (1:400, Dako, Denmark), rabbit anti-Iba1 (1:200, Wako, USA) or rabbit anti-NeuN (1:500, Abcam, Cambridge, UK) at 4 °C overnight. Then, tissue sections were incubated with appropriate anti-rabbit IgG conjugated with FITC (1:100, Abcam, Cambridge, UK) and anti-rabbit IgG conjugated with Alexa 594 (1:1000, Abcam, Cambridge, UK) for 1 h at room temperature. Nuclear staining was performed by 4′,6-diamidino-2-phenylindole (DAPI). Tissue sections were evaluated under fluorescence microscope (Olympus IX71, Japan) and images were captured using DP-72 camera (Olympus, Japan). Quantification of histological results were performed using image J software (version 1.42 V, NIH, USA) as we have described previously (19). Three sections from each slide and 3 slides from each animal were used for histological analysis. The number of animals for each group was 3 mice (27 sections for each experimental group).
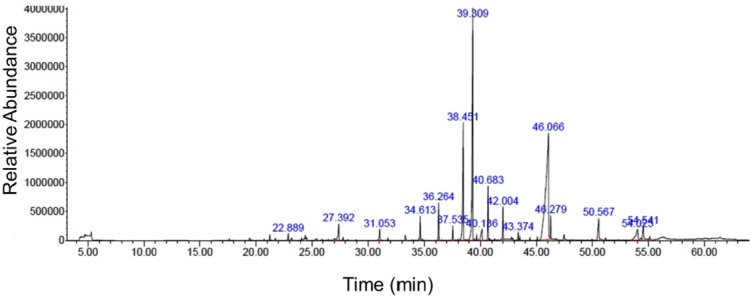
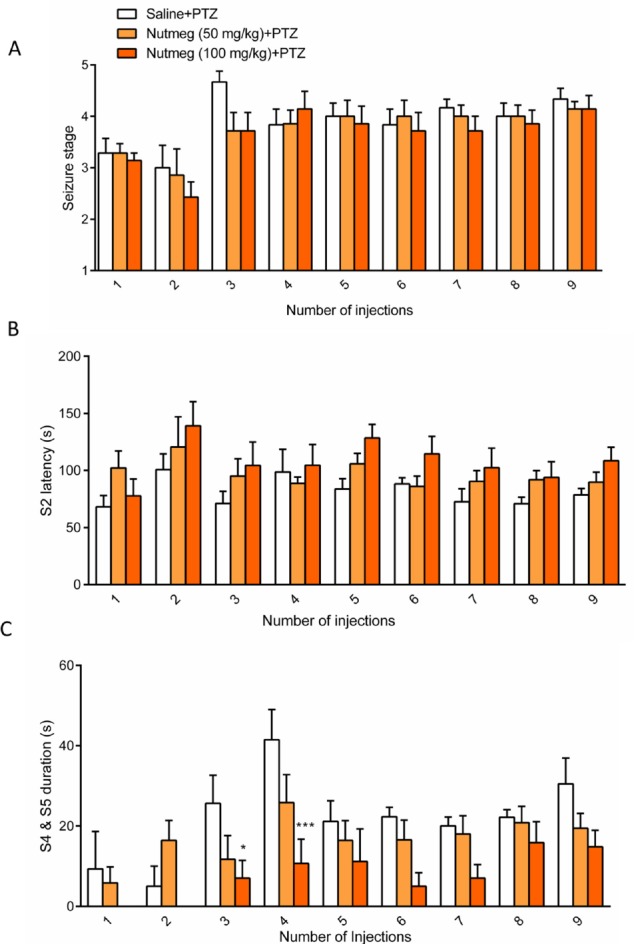
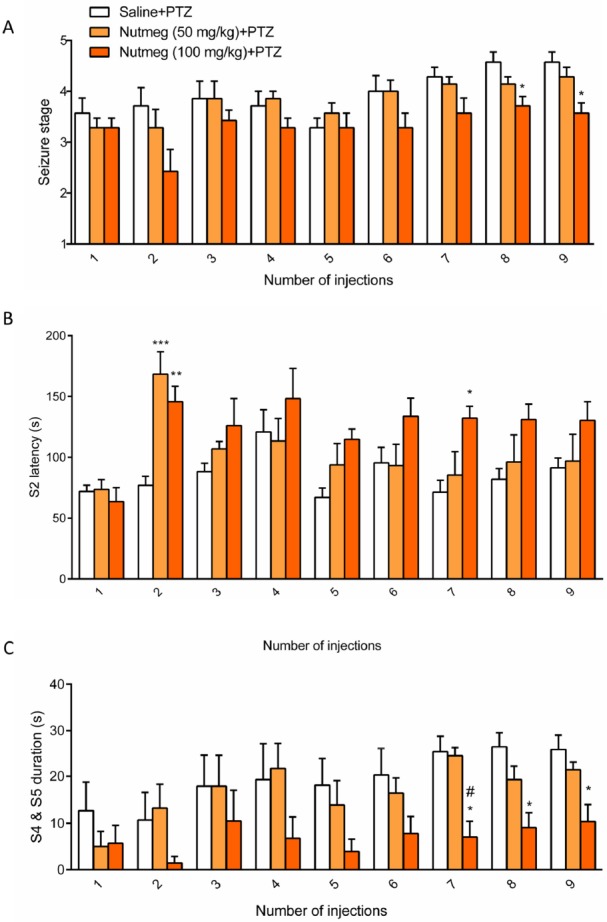
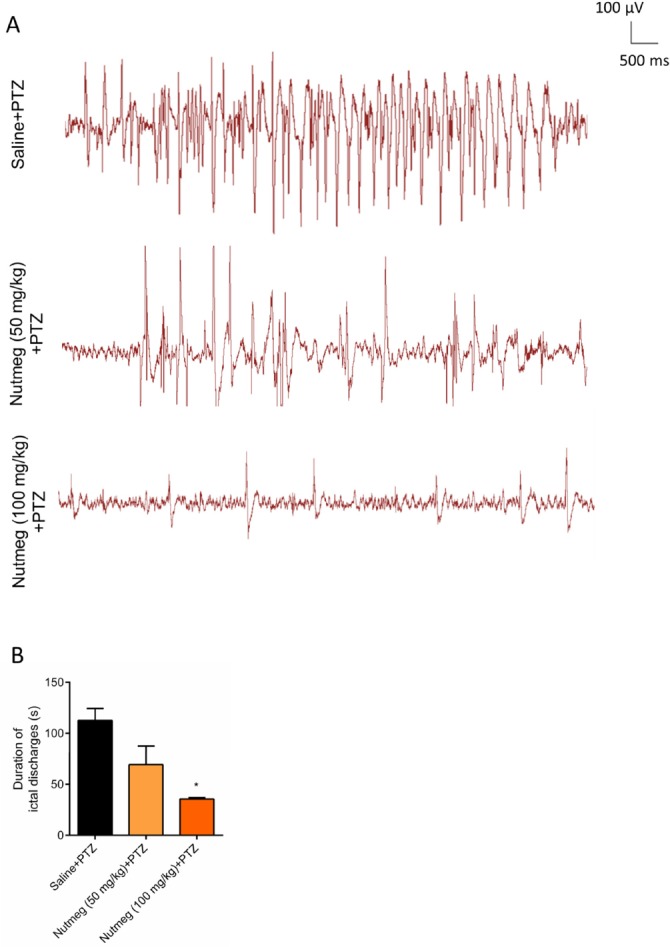
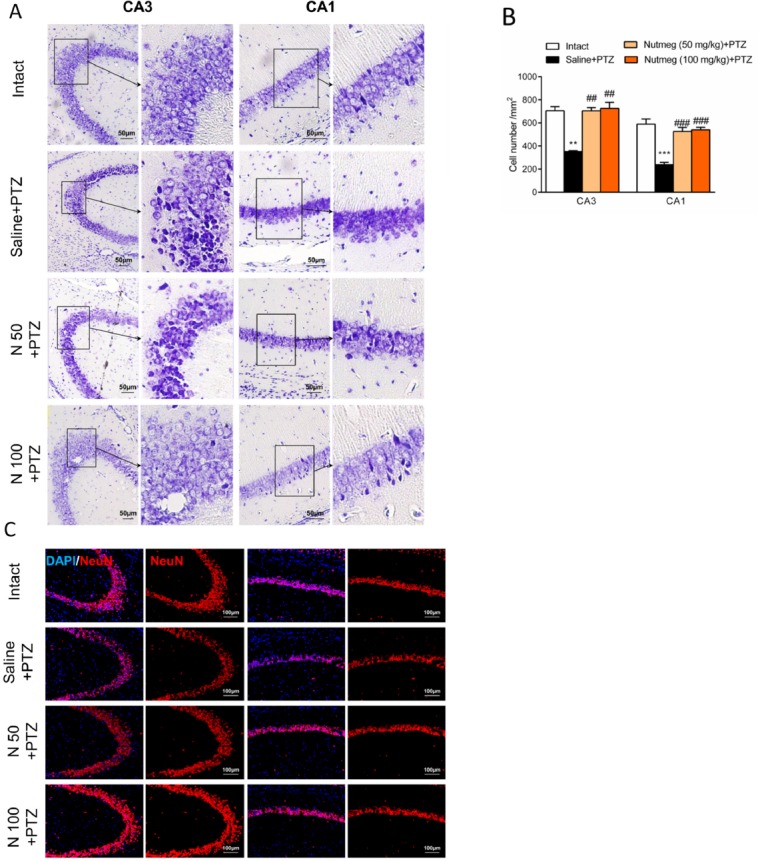
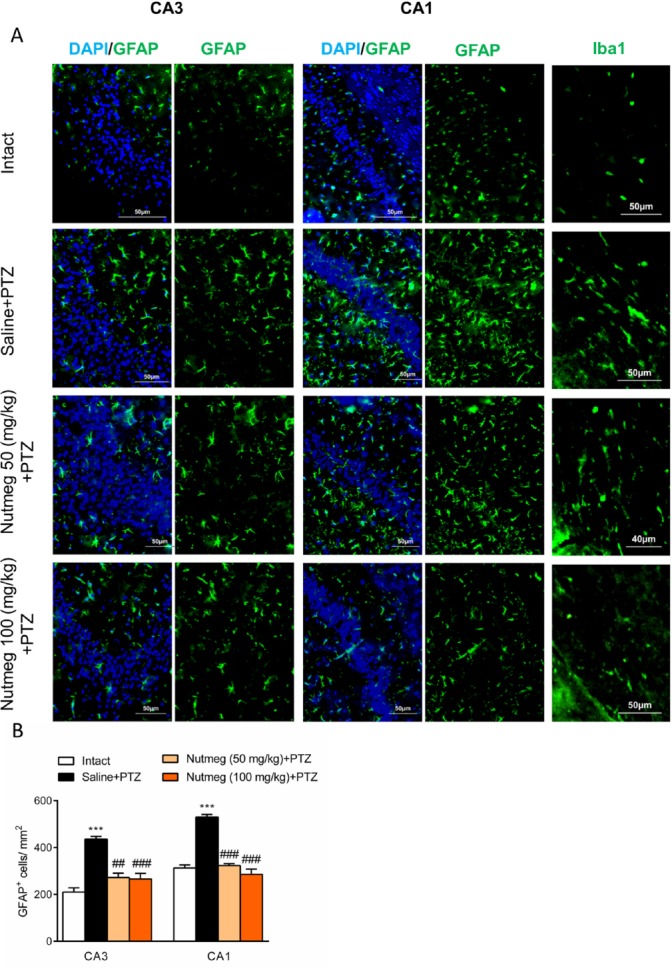
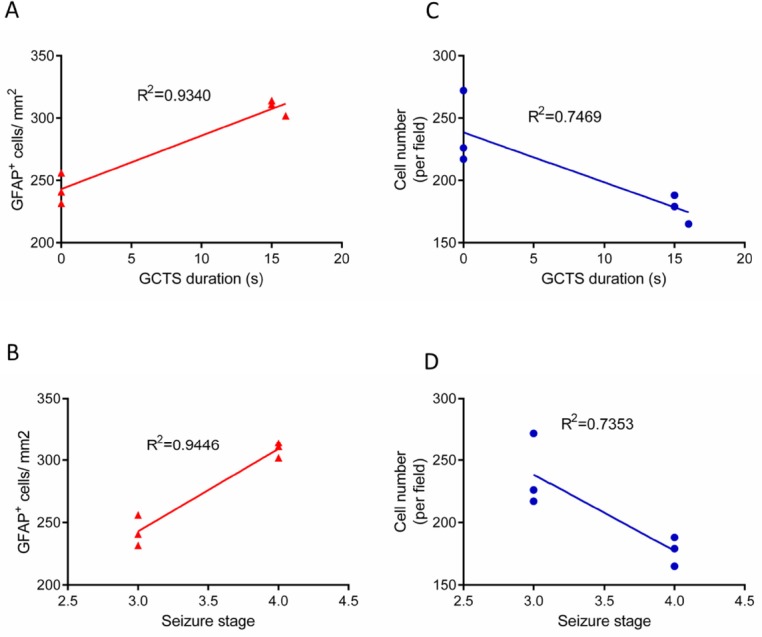
Nutmeg chemical compounds identified using GC-MS
Retention time (min) | Name of compounds | Area (%) |
---|---|---|
22.888 | gamma-Terpinene | 0.48 |
27.389 | 4-Terpinol | 1.79 |
31.054 | Benzene 1,2- (methyllenedioxy)-4-propenyl | 1.02 |
34.611 | 1-cyclopropyl-3,4-dimethyoxyeugenol | 1.76 |
36.270 | lsoeugenol | 2.59 |
37.538 | Cis-Methyl isoeugenol | 0.97 |
38.449 | Myristicin | 11.17 |
39.306 | Elemicin | 22.16 |
40.141 | Lauric acid | 1.90 |
40.683 | Methyl eugenol | 3.80 |
42.006 | Trans-lsoelemicin | 2.14 |
43.372 | Phenol, 2, 6-dimethoxy-4- (2-propeyl) | 0.46 |
46.062 | Myristic acid | 39.93 |
46.279 | Myristic acid ethyl ester | 1.57 |
50.562 | Palmitic acid | 2.86 |
54.021 | 1- Hydroxy-o-methylsterigmatocystin | 3.44 |
54.542 | Oleic acid | 1.96 |
Nissl staining
As mentioned for immunostaining experiments, brain tissues were removed and post-fixed in PFA overnight. Dehydration was done by graded alcohols and tissues were embedded in paraffin. Serial coronal sections (6 µm) were prepared using a microtome (Leica RM2135, Germany). Briefly, after deparaffizination and rehydration with alcohol, nissl bodies in perikaryon were stained using creysl violet (Merck, Germany) for 4 min. Sections were washed with running water, dehydrated, and mounted with entellan (Merck Chemicals, Germany). Images were collected from CA1 and CA3 regions and quantification of nissl staining data was carried out as we have explained for immunostaining procedures.
Statistical analysis
Maximum seizure stages were analyzed by Kruskal-Wallis non-parametric test followed by Dunn′s post-test. Latency to the onset of MJ and GCTS data were assessed using two way-ANOVA, followed by Bonferroni post-hoc test. Histological results were analyzed by one-way ANOVA, followed by Tukey post-test. Linear regression analysis was used to determine the correlation between behavioral and histological results. p values < 0.05 were considered statistically significant.
Results
Myristic acid, elemicin and myristicin as major chemical components of nutmeg extract
Chemical compositions of nutmeg extract were analyzed by GC-MS technique. GC-MS analysis results identified 17 different components representing 100% of the total extract (Table 1). The major compounds of nutmeg extract were myristic acid (39.93%), elemicin (22.16%), and myristicin (11.17%) (Figure1). Other important components are isoeugenol (2.59%), methyl eugenol (3.80%), 1-Hydroxy-o-methylsterigmatocystin (3.44%), trans-Isoelemicin (2.14%) and palmitic acid (2.86%).
Pre-treatment of nutmeg extract decreased seizure behavior in PTZ- induced kindling model
To assess the effect of nutmeg on seizure behavior, alcoholic extracts of nutmeg (50 or 100 mg/kg) were administrated 1 h before every PTZ injection. Behavioral analyses revealed no significant difference in seizure stage between control and nutmeg receiving groups (Figure 2A). Additionally, in animals which received the nutmeg extract, latency to the onset of MJ (S2 latency) was not significantly affected compared to saline group (Figure 2B). Administration of nutmeg extract did not reduce the duration of GCTS and just a significant effect of nutmeg extract was seen at 100 mg/kg dose compared to saline + PTZ at injection 3 (25.667±6.979 in saline +PTZ and 7 ± 4.457 s in nutmeg (100 mg/kg)+PTZ, * p < 0.05) and 4 (41.500±7.478 s in saline +PTZ and 10.667 ± 6.075 s in nutmeg (100 mg/kg) + PTZ, ***p < 0.001) of PTZ (Figure 2C).
To assess the effect of nutmeg pre-treatment on seizure behavior, nutmeg extract was administrated 1 week before PTZ injection and continued until the end of experiment. Behavioral evaluation indicated that the 100 mg/kg dose of nutmeg significantly decreased the mean seizure stage compared to saline + PTZ at injection 8 (4.571 ± 0.20 in saline + PTZ and 3.714±0.1844 in nutmeg (100 mg/kg) +PTZ, *p < 0.005) and 9 (4.571 ± 0.2020 in saline + PTZ and 3.571 ± 0.2020 in nutmeg (100 mg/kg) +PTZ, *p < 0.05) of PTZ (Figure.3A). Additionally, a significant increase in latency of MJ was observed in animals under nutmeg extract treatment at 100 mg/kg dose compared to saline at injections 2 and 7 (Figure. 3B). Furthermore, pre-treatment of animals with dose of 50 mg/kg could also significantly increase the MJ latency at injection 2 of PTZ (***p < 0.001) (Figure. 3B). For the latency of MJ, two way ANOVA revealed a significant main effect of nutmeg extract treatment [F (2, 18) =5.625,
p =0.0127], time [F (8,144) = 6.071, p < 0.0001], as well as nutmeg treatment × time interaction [F (16,144) = 1.948, p = 0.0205]. Interestingly, the duration of GCTS effectively reduced in animals under pre-treatment of higher dose of nutmeg extract compared to saline + PTZ at injections 7, 8 and 9 of PTZ (*p < 0.05) (Figure.3C). In addition, we could find a significant difference in GCTS duration between nutmeg experimental groups at injection 7 of PTZ (#p < 0.05) (Figure 3C). For the duration of GCTS, two way ANOVA revealed a significant main effect of nutmeg treatment [F (2, 16) = 6.458, p = 0.0088], time [F (8, 128) = 6.039, p < 0.0001], but not a significant effect of nutmeg treatment × time
[F (16, 128) = 1.159, p = 0.3095].
In order to evaluate the effect of nutmeg pre-treatment on duration of ictal discharges, electrophysiological recording (EEG) was performed after the last injection of PTZ. Figure 4A is a sample of EEG recordings in PTZ receiving animals. Analysis of EEG recording data indicated that there was robust ictal discharges in fully-kindled animals receiving vehicle. In contrast to vehicle group, pre-treatment of animals with high dose of nutmeg extract significantly reduced the duration of ictal discharges following PTZ injection (112.6 ± 11.70 s in saline + PTZ and 35.52 ± 1.400 s in nutmeg (100 mg/kg) + PTZ (*p < 0.05) (Figure 4B).
Nutmeg pre-treatment reduces cell death in hippocampus following PTZ injection
It has been shown that PTZ-induced seizures cause a remarkable neuronal loss in different brain regions, especially in the hippocampus (19). In order to evaluate the effect of nutmeg pre-treatment on hippocampal cell density, nissl staining was performed on brain sections. Histological staining results and its quantification showed that a high percentage of cell death was observed in CA3 (705.3 ± 34.78 in intact group and 352.1 ±7.622 in saline + PTZ, **p < 0.01) and CA1 regions (589.4 ± 44.30 in intact and 238.8 ± 19.31 in saline +PTZ, ***p < 0.001) of PTZ receiving animals which were treated with saline compared to intact group. In contrast to vehicle group, both doses of nutmeg extract significantly attenuated cell death in CA3 (115.5 ± 2.5 in saline +PTZ, 231 ± 9 in nutmeg (50 mg/kg)+PTZ , ##p < 0.01 and 238 ± 17.03 in nutmeg (100 mg/kg)+PTZ, ##p < 0.01) and CA1 regions of hippocampus (78.33 ± 6.333 in saline +PTZ, 172.7±11.26 in nutmeg (50 mg/kg) + PTZ, ###p < 0.001; 177.3 ± 6.692 in nutmeg (100 mg/kg) +PTZ, ###p < 0.001) (Figure 5A-B). To further confirm the nissl staining data, NeuN antibody was applied on the dorsal hippocampus, as a mature neuronal marker. Immunostaining data indicated that the number of NeuN positive cells in CA3 and CA1 regions of hippocampus were higher in animals under treatment of nutmeg extract compared to saline + PTZ (Figure 5C).
Nutmeg extract pre-treatment reduces glial activation of hippocampus following PTZ injection
Glial activation in hippocampus drastically increases following repetitive administration of PTZ (22). In order to characterize the effect of nutmeg administration on glial activation in PTZ-induced kindling model, immunostaining against GFAP, as an astrocyte marker, was performed on brain sections of the dorsal hippocampus. Immunostaining results and its quantification revealed that the number of GFAP expressing cells increased in fully kindled animals which received saline in comparison to intact animals (CA1: 210.4 ± 17.86 in intact, 436±11.54, ***p < 0.001; CA3: 313 ± 12.97 in intact, 530.5 ± 10.99 in saline +PTZ, ***p < 0.001). Pre-treatment with nutmeg extract at both doses significantly reduced the level of astrocytes activation in both CA3 (436 ± 11.54 in saline + PTZ, 272.4 ± 18.49 in nutmeg (50 mg/kg)+PTZ, ##p < 0.01; 266.3 ± 23.44 in nutmeg (100 mg/kg)+PTZ, ###p < 0.01) and CA1 regions of hippocampus (530.5 ± 10.99 in saline +PTZ, 323.2 ± 8.066 in nutmeg (50 mg/kg) + PTZ, ###p < 0.001; 285.6 ± 22.63 in nutmeg (100 mg/kg)+PTZ, ### p < 0.001) (Figure. 6A-B). In consistence with GFAP staining, immunostaining against Iba1, as a microglia marker, also indicated that Iba1expression remarkably increased in fully-kindled animals and nutmeg administration decreased the activation of microglia in the hippocampus following PTZ injections (Figure 6A-B).
Correlation between seizure behavior and histological results
In order to determine the relationship between seizure behavior, that is, GCTS duration/ seizures stage and GFAP immunostaining results, linear correlation analysis was performed. Linear regression analysis demonstrated that the duration of GCTS and maximum seizures stage were strongly correlated with the level of astrocyte activation in hippocampus of PTZ receiving animals (R2=0.9340, R2=0.9446, respectively) (Figure 7A-B). Furthermore, the correlation between seizure behavior and cell density was determined. Regression analysis data indicated that GCTS and seizures stage were correlated to a lesser extent with hippocampus cell density (R2=0.7469, R2=0.7353) (Figure 7C-D).
Discussion
Accumulating clinical and experimental evidences strongly support the significant role of inflammatory factors in initiation or progression of epilepsy disease (2). Findings of the present study indicate that pre-treatment of nutmeg extract decreased seizure behavior in PTZ receiving animals. Furthermore, the level of glial activation and neuronal loss were attenuated following PTZ application. Previous study by Sonavane et al., showed that a single injection of Myristica fragrans seed extract increased the latency of MJ, and convulsions were completely inhibited in acute seizure model. It seems that the anticonvulsant effect of nutmeg extract might be partly mediated via the alleviation of central dopaminergic activity (23). Additionally, another report by Wahab et al., demonstrated the anticonvulsant activities of nutmeg oil in animal models of seizure. It has been shown that nutmeg oil exhibits a rapid onset and short duration of anticonvulsant effect (24). In contrast to these reports, we did not observe any remarkable effects on seizure behavior when nutmeg extract was administrated 1 h before each PTZ injection. This difference may be due to PTZ dosage, type of extract, and time of nutmeg application. In a study conducted by Sonavane et al., PTZ was administrated at convulsive dose (80 mg/kg) to induce acute seizure model in animals, while in our study, PTZ was administrated at sub-convulsive dose (36.5 mg/kg) to develop kindling model. Additionally, in the former study, nutmeg extract was administrated 30 min before PTZ injection (23). To evaluate the anti-seizure effects of nutmeg extract, we used another approach in which the nutmeg extract was administrated for a week before the injection of PTZ. In contrast to the first approach, pre-treatment of nutmeg extract decreased the frequency and duration of seizures as well as the duration of ictal discharges following PTZ injection. To elucidate the possible molecular mechanism of anti-seizure activity of nutmeg, hippocampal neuronal density was evaluated using histological staining. It has been well-documented that neuronal loss occurs in hippocampus of PTZ receiving animals (20, 21, 25). Our data suggests that the level of cell death was reduced in animals under pre-treatment of nutmeg extract. Furthermore, in agreement with previous studies, PTZ injection increased the level of glial activation in the hippocampus, and nutmeg extract administration ameliorated kindling-induced gliosis (19, 20, 26).
Activated astrocytes and microglia secrete a variety of pro-inflammatory factors which actively participate in the progression of neurodegenerative diseases (27-29). Our data is consistent with previous reports which showed the beneficial anti-inflammatory effects of nutmeg extract, both in-vitro and in-vivo. In parallel with other studies, GC-MS analysis suggested that myristic acid, elemicin and myristicin are major chemical components of nutmeg extracts, which result in important anti-inflammatory effects (30). The anti-inflammatory effects of nonacetyleted fatty acids such as myristic acid has been well understood (31). It has been proposed that myristic acid exerts its anti-inflammatory action through inhibition of phospholipase A2 enzyme (32). Myristicin significantly ameliorates inflammation via its inhibitory role on production of nitric oxide (NO), cytokines, chemokine, and growth factors in dsRNA-stimulated macrophages (33). Furthermore, the anti-inflammatory effect of myristicin has been investigated in carrageenin-induced edema in rats and acetic acid-induced vascular permeability in mice. It was observed that the anti-inflammatory activity of myristicin was the same as indomethacin (14). Additionally, phenylpropanoids including elemicin and myristicin inhibit the production of IL-1β and IL-6 which lead to beneficial anti-inflammatory effects in lung inflammation (34). Myristica fragrans suppressed the production of NO and cyclooxygenase (COX-2) which are crucial inflammation mediators in lipopolysaccharide (LPS)-induced inflammation model (35). In addition, nutmeg oil can potentially alleviate the chronic inflammatory pain by inhibiting COX-2 expression and releasing substance P (15). Furthermore, other components of nutmeg extract, such as eugenol, also possess important anti-inflammatory activity. It has been well addressed that eugenol inhibits the production of pro-inflammatory cytokines and suppresses redox signaling pathway in LPS-induced lung inflammation (36).
Overall, this study indicates that myristic acid, elemicin, and myriticin are the major components of ethanolic extract of nutmeg. Pre-treatment of nutmeg extract reduce seizure behavior in PTZ receiving animals. The anti-convulsant activity of nutmeg extract is partly mediated through the amelioration of glial activation. Furthermore, the level of hippocampal neuronal loss was alleviated in animals under treatment of nutmeg extracts. According to these findings, nutmeg extract might be useful as a natural supplementary compound in epileptic patients.
Acknowledgements
References
-
1.
Badawy RA, Harvey AS, Macdonell RA. Cortical hyperexcitability and epileptogenesis: understanding the mechanisms of epilepsy - part 1. J.Clin.Neurosci. 2009;16:355-65. [PubMed ID: 19124246].
-
2.
Vezzani A, French J, Bartfai T, Baram TZ. The role of inflammation in epilepsy. Nat. Rev. Neurol. 2011;7:31-40. [PubMed ID: 21135885].
-
3.
Vezzani A, Granata T. Brain inflammation in epilepsy: experimental and clinical evidence. Epilepsia. 2005;46:1724-43. [PubMed ID: 16302852].
-
4.
Vezzani A, Aronica E, Mazarati A, Pittman QJ. Epilepsy and brain inflammation. Exp. Neurol. 2013;244:11-21. [PubMed ID: 21985866].
-
5.
Vezzani A, Balosso S, Ravizza T. The role of cytokines in the pathophysiology of epilepsy. Brain. Behav. Immun. 2008;22:797-803. [PubMed ID: 18495419].
-
6.
Jung KH1, Chu K, Lee ST, Kim J, Sinn DI, Kim JM, Park DK, Lee JJ, Kim SU, Kim M, Lee SK, Roh JK. Cyclooxygenase-2 inhibitor, celecoxib, inhibits the altered hippocampal neurogenesis with attenuation of spontaneous recurrent seizures following pilocarpine-induced status epilepticus. Neurobiol. Dis. 2006;23:237-46. [PubMed ID: 16806953].
-
7.
Somera-Molina KC, Nair S, Van Eldik LJ, Watterson DM, Wainwright MS. Enhanced microglial activation and proinflammatory cytokine upregulation are linked to increased susceptibility to seizures and neurologic injury in a ′two-hit′ seizure model. Brain. Res. 2009;1282:162-72. [PubMed ID: 19501063].
-
8.
Schmidt D. Efficacy of new antiepileptic drugs. Epilepsy Curr. 2011;11:9-11. [PubMed ID: 21461260].
-
9.
Ortinski P, Meador KJ. Cognitive side effects of antiepileptic drugs. Epilepsy. Behav. 2004;5:60-5.
-
10.
Nsour W, Lau C-S, Wong I. Review on phytotherapy in epilepsy. Seizure. 2000;9:96-107. [PubMed ID: 10845732].
-
11.
Nagja T, Vimal K andSanjeev A. Myristica fragrans: a comprehensive review. Int. J. Pharm. Pharm. Sci. 2016;8:27-30.
-
12.
Gupta AD, Bansal VK, Babu V, Maithil N. Chemistry, antioxidant and antimicrobial potential of nutmeg (Myristica fragrans Houtt). J. Genet. Eng. Biotechnol. 2013;11:25-31.
-
13.
Olaleye M, Akinmoladun C, Akindahunsi A. Antioxidant properties of Myristica fragrans (Houtt) and its effect on selected organs of albino rats. Afr. J. Biotechnol. 2006;5:1274-78.
-
14.
Ozaki Y, Soedigdo S, Wattimena YR, Suganda AG. Antiinflammatory effect of mace, aril of Myristica fragrans Houtt and its active principles. Jpn. J. Pharmacol. 1989;49:155-63. [PubMed ID: 2487032].
-
15.
Zhang WK, Tao SS, Li TT, Li YS, Li XJ, Tang HB, Cong RH, Li Ma F, Wan CJ. Nutmeg oil alleviates chronic inflammatory pain through inhibition of COX-2 expression and substance P release in vivo. Food Nutr. Res. 2016;60:30849. [PubMed ID: 27121041].
-
16.
Lee JY, Park W. Anti-inflammatory effect of myristicin on RAW 2647 macrophages stimulated with polyinosinic-polycytidylic acid. Molecules. 2011;16:7132-42. [PubMed ID: 21991618].
-
17.
Dhir A. Pentylenetetrazol (PTZ) kindling model of epilepsy. Curr. Protoc. Neurosci. 2012;9:1-9.
-
18.
Naeimi R, Ghasemi-Kasman M, Kazemi S, Ashrafpour M, Moghadamnia AA, Pourabdolhossein F. Zingiber officinale extract pre-treatment ameliorates astrocytes activation and enhances neuroprotection in pentylenetetrazol-induced kindling model of epilepsy in mice. Physiol. Pharmacol. 2018;22:92-102.
-
19.
Hashemian M, Anissian D, Ghasemi-Kasman M, Akbari A, Khalili-Fomeshi M, Ghasemi S, Ahmadi F, Moghadamnia AA, Ebrahimpour A. Curcumin-loaded chitosan-alginate-STPP nanoparticles ameliorate memory deficits and reduce glial activation in pentylenetetrazol-induced kindling model of epilepsy. Prog. Neuropsychopharmacol. Biol. Psychiatry. 2017;79:462-71. [PubMed ID: 28778407].
-
20.
Anissian D, Ghasemi-Kasman M, Khalili-Fomeshi M, Akbari A, Hashemian M, Kazemi S, Moghadamnia AA. Piperine-loaded chitosan-STPP nanoparticles reduce neuronal loss and astrocytes activation in chemical kindling model of epilepsy. Int. J. Biol. Macromol. 2018;107:973-83. [PubMed ID: 28939512].
-
21.
Gol M, Ghorbanian D, Hassanzadeh S, Javan M, Mirnajafi-Zadeh J, Ghasemi-Kasman M. Fingolimod enhances myelin repair of hippocampus in pentylenetetrazol-induced kindling model. Eur. J. Pharm. Sci. 2016;96:72-83. [PubMed ID: 27634580].
-
22.
Saha L, Bhandari S, Bhatia A, Banerjee D, Chakrabarti A. Anti-kindling effect of bezafibrate, a peroxisome proliferator-activated receptors alpha agonist, in pentylenetetrazole induced kindling seizure model. J. Epilepsy Res. 2014;4:45-54. [PubMed ID: 25625088].
-
23.
Sonavane G, Palekar R, Kasture V, Kasture S. Anticonvulsant and behavioural actions of Myristica fragrans seeds. Indian. J. Pharmacol. 2002;34:332-8.
-
24.
Wahab A, Haq RU, Ahmed A, Khan RA, Raza M. Anticonvulsant activities of nutmeg oil of Myristica fragrans. Phytother. Res. 2009;23:153-8. [PubMed ID: 19067329].
-
25.
Naseer M, Ullah N, Ullah I, Koh P, Lee H, Park M, Kim MO. Vitamin C protects against ethanol and PTZ‐induced apoptotic neurodegeneration in prenatal rat hippocampal neurons. Synapse. 2011;65:562-71. [PubMed ID: 20963815].
-
26.
Zhu X, Dong J, Shen K, Bai Y, Zhang Y, Lv X, Chao J. HNMDA receptor NR2B subunits contribute to PTZ-kindling-induced hippocampal astrocytosis and oxidative stress. Brain. Res. Bull. 2015;114:70-8. [PubMed ID: 25896886].
-
27.
Ben Haim L, Carrillo-de Sauvage MA, Ceyzeriat K, Escartin C. Elusive roles for reactive astrocytes in neurodegenerative diseases. Front. Cell Neurosci. 2015;9:278.
-
28.
Liu B, Hong JS. Role of microglia in inflammation-mediated neurodegenerative diseases: mechanisms and strategies for therapeutic intervention. J. Pharmacol. Exp. Ther. 2003;304:1-7. [PubMed ID: 12490568].
-
29.
Maragakis NJ, Rothstein JD. Mechanisms of Disease: astrocytes in neurodegenerative disease. Nat. Clin. Pract. Neurol. 2006;2:679-89. [PubMed ID: 17117171].
-
30.
Yang XW, Huang X, Ahmat M. New neolignan from seed of Myristica fragrans. Zhongguo Zhong Yao Za Zhi. 2008;33:397-402. [PubMed ID: 18533495].
-
31.
Sharma N, Samarakoon KW, Gyawali R, Park Y-H, Lee S-J, Oh SJ, Jeong DK. Evaluation of the antioxidant, anti-inflammatory, and anticancer activities of Euphorbia hirta ethanolic extract. Molecules. 2014;19:14567-81. [PubMed ID: 25225720].
-
32.
Gopi K, Renu K, Jayaraman G. Inhibition of Naja naja venom enzymes by the methanolic extract of Leucas aspera and its chemical profile by GC–MS. Toxicol. Rep. 2014;1:667-73. [PubMed ID: 28962280].
-
33.
Lee JY, Park W. Anti-inflammatory effect of myristicin on RAW 2647 macrophages stimulated with polyinosinic-polycytidylic acid. Molecules. 2011;16:7132-42. [PubMed ID: 21991618].
-
34.
Lim HJ, Woo KW, Lee KR, Lee SK, Kim HP. Inhibition of Proinflammatory Cytokine Generation in Lung Inflammation by the Leaves of Perilla frutescens and Its Constituents. Biomol. Ther. 2014;22:62-7.
-
35.
Min BS, Cuong TD, Hung TM, Min BK, Shin BS, Woo MH. Inhibitory Effect of Lignans from Myristica fragrans on LPS-induced NO Production in RAW2647 Cells B. Korean. Chem. Soc. 2011;32:4059-62.
-
36.
Huang X, Liu Y, Lu Y, Ma C. Anti-inflammatory effects of eugenol on lipopolysaccharide-induced inflammatory reaction in acute lung injury via regulating inflammation and redox status. Int. J. Immunopharmacol. 2015;26:265-71.