Abstract
Keywords
Pharmaceutical Carbon nanostructures Graphene Carbon Nanotube Nanoparticle Voltammetric Modified electrode
Introduction
Pharmaceutical analysis should be performed during all steps of pharmaceutical development (1-3), from the early stage of synthesis, formulation, stability testing and quality control to toxicological and pharmacological investigations in animals and humans in process development including preclinical and clinical trials (4, 5). After administration to patients, analytical measurements are essential for bioavailability testing and also for evaluating their effectiveness and investigation of the needed dosage of drug formulation (6, 7). Quantitative analysis of active ingredient content in drug formulations and also after application in human biological fluids is inevitable. While various analytical techniques have been developed for this aim, electrochemical techniques have been considered as sensitive, easy operation, efficient and cost effective methods which can be rapidly and accurately employed for pharmaceutical assay (7). Compared to other analytical methods, electrochemical determination has demonstrated to be highly sensitive and reliable with less interference from non-electro-active species for the determination of wide range of pharmaceutical agents (8, 9). Over the past two decades, electrochemical behaviour of drugs such as ascorbic acid, phenothiazines, benzodiazepines, paracetamol, tramadol, theophylline, sumatriptan, naltrexone, mercaptopurine, gabapentin, lamotrigine, etc. has been widely investigated and a great trend in pharmaceutical analysis can be seen for developing electrochemical sensors. In addition, intelligent drug delivery systems or implantable sensors for the rapid detection of biomarker and the release of therapeutic agents on demand can be designed based on these sensors (10).
Development of modified electrodes
Electrochemical techniques have been widely employed for both qualitative and quantitative analysis of organic (11-14) and inorganic (15, 16) compounds. In electrochemistry, working electrode surface plays an important role as the selectivity and sensitivity of the measurements is highly depended on its properties (17).
In voltammetry measurements, potential and current of resulted peak (s) in recorded voltammograms show two important characteristic of the target species. Peak potential specify the electro-active spices (18), while peak current is a function of the analyte concentration in the sample solution, effective surface area of the electrode and number of electrons transferred between analyte and electrode during redox reaction process (19). Notably, electrochemical response of most of the species is poor or insensible at low concentrations at ordinary bare electrodes such as carbon paste (20), glassy carbon (21), Pt (22, 23) or Au (24) electrodes. Chemically modified electrodes (CMEs) have attracted much interest in the construction of electro-chemical sensors as they can overcome mentioned intrinsic problems, and facilitate the interfacial electron transfer (25-28). Chemically modified electrodes are constructed by bonding or coating a thin film of mono or multi molecular, ionic or polymeric compounds onto the surface of a conducting or semiconducting substrate. The response of the modified electrodes exhibits the chemical or electrochemical properties of the modifier materials in the faradaic reactions. Here, enhancement of the desirable electrochemical properties and attenuation or elimination of the unwanted properties takes place. For a constant amount of analyte the peak current can be enhanced by increasing the electrode surface area such as surface modification with nanostructured materials (29). Also, surface modification can change the peak potential positively or negatively, depending on the modification process which can increase or decrease the over-potential of the redox reactions. This over-potential change can be related to changing the charge transfer way or electro-catalysis process or variation in mass transfer phenomena (30). A vast range of materials has been employed as electrode modifier toward improvement of sensitivity and selectivity of the electrochemical responses (20-22, 25-28 and 31-33). In recent decades, carbon nanostructured materials have well-played roles in electrochemistry. They are used as electrode modifier in a wide range of electrochemical experiments, from sensors (34-36) and biosensor (34, 37-39) to batteries (40), fuel cells (41), solar cells (42, 43), super capacitors (44, 45), and so on. Carbon nanostructures have attracted significant attention of researchers in the field of development of modified electrodes due to their unique properties such as high electrical conductivity, chemical stability, and very large surface area (46, 47). Many papers have been published on carbon nanostructured based modified electrodes (22, 47-49).
Carbon nanostructured based electrode modification
Various ways have been applied for electrode modification by carbon nanostructures. Generally, electrode modification includes bulk or surface modification. In simplest method, suspension of various types of carbon nanostructures is prepared in a suitable organic or inorganic solvent such as water, dimethyl form amide (DMF), or mixture of them (50, 51). Then, the optimized adequacy of this suspension was drop casted on the electrode surface, on which a thin layer of modifier is formed after solvent evaporation (50-53). Even though the operation of this method is very easy, the dispersion of some materials in applicable solvents is not possible. For example, strong interaction between multiwalled carbon nanotubes (MWCNTs) hinders their dispersion in any solvent. Notably, even after applying long time and intensive sonication for dispersing them, the resulted suspension is not stable and MWCNTs immediately precipitate from the solvent. Therefore, this property negatively affects the reproducibility of the modification process. To overcome this problem, in most works, before suspension preparation step, MWCNTs are functionalized for example with acid treatment, which facilitates their dispersion and stability of the prepared final modifier sample (54-56). Moreover, some polymeric binders such as chitosan (50), nafion (57), poly (vinyl alcohol) (58), polyaniline (41) and electropolymerized polypyrrole (59) have been employed to facilitate the dispersion of the carbon-based materials and to improve the final stability of the modifier thin film. In some cases, modifier is added to the bulk material of the electrode. This way is mostly used for preparation of the carbon paste modified electrodes (60-65). Moreover, covalent bonding with the help of linking agents, chemisorption via self-assembled monolayers, or electrochemically attachment of modifier to the electrode surface are other ways employed for electrode surface modification. Although, graphene oxide (GO) suspension is easily prepared and stays stable for long time, the reduced graphene oxide (RGO) is hardly dispersed and the obtained suspension is not stable. Therefore, modification of the electrode surface with RGO employing chemically synthesized RGO is time consuming and undesirable. However, GO can be electrochemically reduced and attached onto the electrode surface. In addition, composite of various compounds with carbon nanostructures were used for electrode modification, which combined the synergistic properties of different materials in a simple and versatile way while facilitated the control of preparation conditions (60, 62-70).
Various types of carbon based nanostructures were employed in electrode modification such as single and multi-walled carbon nanotubes (71-79), graphene oxide and reduced graphene oxide (80-84), graphene (85-87), fullerenes (88), carbon nanoparticles (89-98), carbon black (99-102), and carbon quantum dots (Scheme 1) (103-107). Most of these modifiers improve effective surface area while facilitate the electron transfer between analytes and electrode surface in the electrode-solution interface. In addition, they more effectively adsorbed the analytes and enhanced the electrochemical responses as a result of pre-accumulation on the electrode surface (106-109). Some works dealing with modified electrodes prepared, using carbon nanostructures for pharmaceutical applications aim were listed in Tables 1 to 3.
The combination of CNSs with other nano-structured materials such as metals, metal oxides, and polymers has provided multifunctional hybrid or composites that can significantly improve the sensing properties of the electrochemical sensors. Nano-materials with various type of morphology such as nano-particles, nano-wiers, nano-ribbons, nano-spheres are integrated with CNSs. The prepared heterogeneous nanostructures show outstanding promising potential in sensing applications.
Nanocomposites of Au nanoparticle coated β-cyclodextrin functionalized RGO were synthesized by Pham et al. (110). They modified the GCE surface by a thin film of synthesized nano composite and employed it for ciprofloxacin determination. They successfully achieved the detection limit of 2.7 nM by employing differential pulse voltammetry. Recently, He et al. constructed an electrochemical sensor based on Cu2O-reduced graphene oxide nano-composite (Cu2O-RGO) employed for dopamine (DA) analysis (111). A wide range of 0.01 to 80 µM with low detection limit of 6.0 nM was resulted for DA determination. Also, about 204 and 144 mV of peak to peak separation were observed between oxidation peaks of ascorbic acid (AA)-DA and DA-uric acid (UA), respectively. Therefore, analysis of DA can be performed without interference of AA or UA. In another attempt, Nafion/carboxylated-MWCNTs nanocomposite was used for modification of the GCE surface (112). This sensor was applied for timolol maleate (TM) determination. Linear range of 1.0 nM to 20 µM coupled with 0.7 nM detection limit was resulted in analysis evaluation. High selectivity of the prepared sensor toward TM provided its accurate determination in eye drop, urine and water samples without interference of other organic or inorganic compounds. Sharma et al. reviewed the surface modified electrodes employed for serotonin detection (113). They reported that various nanocomposite of carbon nanostructures have been used for serotonin sensor construction. For example, a nanocomposite of poly(p-aminobenzene sulfonic acid), (poly-P-ABSA), MWCNTs, and chitosan were prepared and employed for surface modification of the GCE. Here, the electrostatic interactions between negatively charged poly-P-ABSA and positively charged chitosan and special structure of CNTs formed a conductive and stable thin film on the GCE surface. They attributed high conductivity, electrocatalytic activity and adsorption ability properties for carbon nanostructured based composites that effectively improve the sensing ability. The electrochemical behavior of isoxsuprine (ISOX) was evaluated using a GCE modified with nanocomposite of MWCNTs decorated with Ag nanoparticles (114). Two oxidation peaks were observed for ISOX revealing two steps irreversible oxidation. Adsorption of ISOX molecules on the modifier film improved the sensitivity of the electrochemical analysis. High repeatability and reproducibility in response to ISOX beside resulted low detection limit of 12.0 nM revealed applicability of the introduced procedure for ISOX analysis. A flexible screen-printed modified electrode based on electrochemically RGO-CB nano-composite has been employed for detection of dopamine, epinephrine, and paracetamol (115). The hydrophilic surface having high amount of defect sites enhanced the accumulation of target analytes onto the electrode surface, which sensibly improved the current response. This sensor was able to do simultaneous electrocatalytic analysis of three mentioned analytes with very low detection limit. These results confirm the outstanding electrochemical properties of modified electrode based on composite of CNSs.
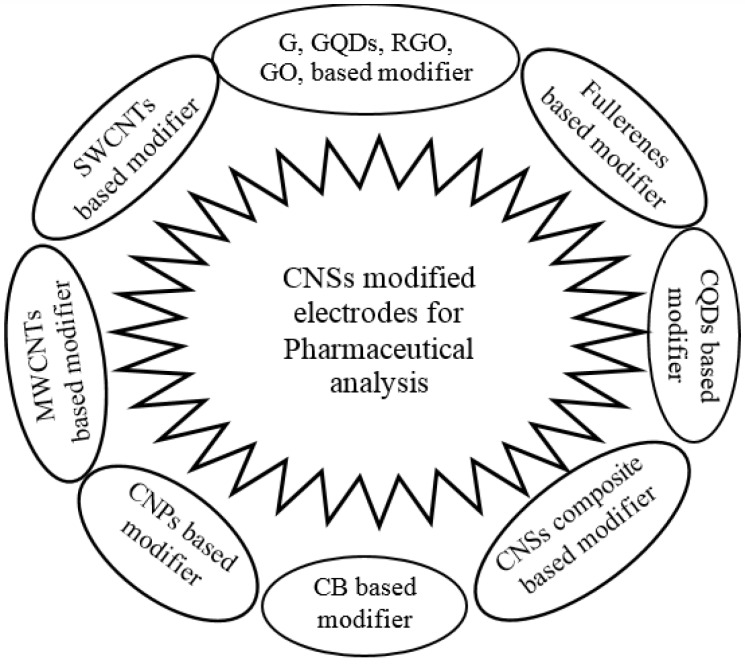
Comparison of figures of merit of MWCNTs modified electrodes used in pharmaceutical analysis
Modified electrode | Analyte | Method | LRa(μM) | DLb(nM) | Reference |
---|---|---|---|---|---|
P-Dopa/ MWCNTs-COOH/GCE | Metronidazole | DPV | 5-5000 | 250 | |
MWCNT/PMB/AuNP/GCE | Nevirapine | DPASVe | 0.1-50 | 53 | |
MWCNTs/GO/pyrogallol/GCE | Omeprazole | DPV | 0.0002-100 | 0.01 | |
Functionalized MWCNTs/GCE | MebeverineHydrochloride | SWAdASVf | 0.0005-0.035 | 0.13 | |
g1-M-3-OITFB/ZnO/CNTs/CPE | Raloxifene | DPV | 0.08-400 | 40 | |
Poly(o-anisidine)/CNTs/GCE | Mebendazole | DPV | 1-35 | 400 | |
3DG-CNTN/GCE | Methotrexate | DPV | 0.7-199 | 70 |
Comparison of figures of merit of GO, RGO and Gr modified electrodes used in pharmaceutical analysis
Modified electrode | Analyte | Method | LRa(μM) | DLb(nM) | Reference |
---|---|---|---|---|---|
GNS-CNTs/MoS2/GCEc | Dopamine | DPV | 0.1-100 | 50 | |
PP3CA/ERGO/GCEd | Breast Cancer (BRCA) | DPV | 10 fM–0.1 | 3 fM | |
AuNP-rGO-CS/GCEe | Methylparaben | SWV | 0.03-1.3 | 13.77 | |
AuNP-rGO/GCE | Hesperidin | Amperometry | 0.05-8 | 8.2 | |
Graphen/Carbon paste electrode | Promazine | SWV | 0.1-8 | 8 | |
Gr nanoplatelets-carbon nanofibers/GCE | Nepafenac | AdSSWV | 0.25-15 | 63 | |
Graphene/TiO2/V2O5/CPE | Chlorpromazine | DPV | 0.033-85 | 5.3 |
Comparison of figures of merit of CNPs, carbon black and CQDs modified electrodes used in pharmaceutical analysi
Modified electrode | Analyte | Method | LRa(μM) | DLb (nM) | Reference |
---|---|---|---|---|---|
Activated CNPs/CPE | Naproxen | DPV | 0.1-120 | 23.4 | |
CNPs/SDS/CPEc | Dopamine | DPV | 0.1-100 | 120 | |
CNP/GCE | Acetaminophen | DPV | 0.1-100 | 50 | |
Tramadole | 10-1000 | 500 | |||
CNP/GCE | Ractopamine | DPV | 2-30 | 0.2 | |
Nanocellulose/CNP/GCE | Metoclopramide | LSV | 0.06-2 | 6 | |
Carbon nanofiber/CNP/GCE | Folic acid | DPV | 0.1-10 | - | |
Melamine/CNPs/GCE | Raloxifene | DPV | 0.4-2 | 10 | |
TiO2/Nafion/CNP/GCE | Dobutamine | ASDPV | 0.006-1 | 2 | |
CB/Ag/PEDOT,PSS/GCE | Paracetamol | SWV | 0.62-7.1 | 12 | |
Levofloxacin | 0.67-12 | 14 | |||
CB/GCE | Estradiol | Ampreometry | 0.15-3.5 | 92 | |
CB/GCE | L-cysteine | Chronoamperometry | 50-700 | 45.87 | |
CB/GCE | Ethinyl Estradiol | DPV | 0.25-3 | 0.13 | |
CQD/GCE | Dopamine | LSVe | 0.19-11.81 | 2.7 | |
PBG/CQDs/GCEf | Guanine | DPV | 0.5-142 | 16 | |
Adenine | 0.3-130 | 26 | |||
β-CD/CQD/GCEg | Dopamine | DPV | 4-220 | 14 | |
Tryptophan | 5-270 | 16 |
Comparison of figures of merit of modified electrodes used for dopamine analysis
For analysis of some certain drugs and pharmaceutical compounds the modified electrodes based on various types of carbon nano-structures were developed. For example, MWCNTs and SWCNTs, CNPs, GO, RGO and also carbon black or carbon quantum dots were employed for electrode modification, used in Dopamine (DA) analysis (80, 115 and 116-120), Table 4. Notably, due to high surface area and good conductivity of the mentioned carbon nanostructures, the modified electrode only based on one type of carbon nanostructure provided almost same sensitivity and linear range of determination or limit of detection for DA. However, as mentioned in Table 4, employing hybrid or composite materials of carbon nanostructures effectively improved the linear range and lowered the detection limit of DA analysis.
Advantages and limitations in clinical complex samples bioanalysis
Even though, carbon nanostructures based modifiers significantly have improved the analysis sensitivity (28, 47 and 77), as demonstrated, they can respond to the wide range of compounds. Therefore, in most cases they are unable to discriminate between responses of compounds with similar electro-active functional groups in their structures, especially in case of biological fluids which have very complex nature, due to including a wide range of organic and inorganic compounds. For example, as reported, carbon based modified electrodes can effectively improve the response of dopamine, epinephrine, norepinephrine, and other similar compounds. Notably, as the main functional group that undergoes redox reaction in their compounds is quinone - hydroquinone part, discrimination between their responses is not easy and employing carbon nanostructure as electrode modifier alone cannot provide sensible selectivity between them. Therefore, their accurate simultaneous quantification in a mixture solution such as human blood or urine sample is not possible. As reported, addition of other materials such as metal (22, 84), metal oxides (75, 87), organometallic nano-materials (109, 121), and polymeric compounds (71, 73, 76, 104 and 105) and so on to the modification procedure beside the carbon nanostructures can overcome this problem.
Conclusion
Electrochemical methods employing carbon-based nanostructures can be efficiently employed for pharmaceutical and clinical analysis. However, to improve the selectivity and to access to more sensitivity, it is recommended to apply mixture, hybrid, or composite of these nano-materials with other organic or inorganic compounds. Therefore, it is possible to benefit synergetic or enhancement effect of them together. So, we can access to selective determination of active ingredients of drugs in both bulk pharmaceutical formulation or after administration in biological fluid of the patients’ bodies.
References
-
1.
Akay C, Tuncer D, Egim I, Sayal A, Aydin A, Özkan Y, Gül H. Rapid and simultaneous determination of acetylsalicylic acid, paracetamol, and their degradation and toxic impurity products by HPLC in pharmaceutical dosage forms. Turk. J. Med. Sci. 2008;38:167-73.
-
2.
Badyal PN, Sharma C, Kaur N, Shankar R, Pandey A, Rawal RK. Analytical techniques in simultaneous estimation: An overview. Austin J. Anal. Pharm. Chem. 2015;2:1037-50.
-
3.
Bunaciu AA, Aboul-Enein HY, Fleschin S. Application of fourier transform infrared spectrophotometry in pharmaceutical drugs analysis. Appl. Spectrosc. Rev. 2010;45:206-19.
-
4.
Barile FA. Published the chapter: Clinical toxicology: Principles and Mechanisms. 1st ed. CRC Press: Washington DC; 2003.
-
5.
Adams IB, Martin BR. Cannabis: pharmacology and toxicology in animals and humans. Addiction. 1996;91:1585-614. [PubMed ID: 8972919].
-
6.
Dustin C. An investigation into formulation and therapeutic effectiveness of nanoparticle drug delivery for select pharmaceutical agents. East Tennessee State Universtiy. 2016:Paper 3024.
-
7.
Siddiqui MR, AlOthman ZA, Rahman N. Analytical techniques in pharmaceutical analysis: A review. Arabian J. Chem. 2013;10:S1409-S1421.
-
8.
Abo El-Maali N. Voltammetric analysis of drugs. Bioelectrochem. 2004;64:99-107.
-
9.
Gupta VK, Jain R, Radhapyari K, Jadon N, Agarwal S. Voltammetric techniques for the assay of pharmaceuticals-A review. Anal. Biochem. 2011;408:179-96. [PubMed ID: 20869940].
-
10.
Ghorbani-Bidkorbeh F. electrochemical sensors and biosensors represent very promising tools in pharmaceutical sciences. Iran. J. Pharm. Res. 2015;14:663-4. [PubMed ID: 26330854].
-
11.
Cavalheiro ETG, Brett CMA, Oliveira-Brett AM, Fatibello-Filho O. Bioelectroanalysis of pharmaceutical compounds. Bioanal. Rev. 2012;4:31-53.
-
12.
Nussbaumer S, Bonnabry P, Veuthey JL, Fleury-Souverain S. Analysis of anticancer drugs: A review. Talanta. 2011;85:2265-89. [PubMed ID: 21962644].
-
13.
Huck CW, Stecher G, Scherz H, Bonn G. Analysis of drugs, natural and bioactive compounds containing phenolic groups by capillary electrophoresis coupled to mass spectrometry. Electrophoresis. 2005;26:1319-33. [PubMed ID: 15776479].
-
14.
Aragón P, Atienza J, Climent MD. Analysis of organic compounds in air: A review. Critical Rev. Anal. Chem. 2010;30:121-51.
-
15.
Santos A, Takeuchi RM, MuÇoz RAA, Angnes L, Stradiotto NR. Electrochemical determination of inorganic contaminants in automotive fuels. Electroanalysis. 2012;24:1681-91.
-
16.
Bhargavi M, Gumpu, Sethuraman S, Krishnan UM, Rayappan JBB. A review on detection of heavy metal ions in water–an electrochemical approach. Sensor Actuat. B-Chem. 2015;213:515-33.
-
17.
Cox JA, Przyjazny A. Electrochemistry of biopolymers. TrAC-Trends Anal. Chem. 1992;11:298-302.
-
18.
Bard AJ, Faulkner LR. Electrochemical Methods: Fundamentals and Applications. 2nd ed. New York: John Wiley and Sons Inc; 2001. 164 p.
-
19.
Brett C, Brett AM. Electrochemistry Principles Methods and Applications. 1st ed. Oxford, England: Oxford Science Publications; 1993.
-
20.
Shahrokhian S, Ghalkhani M. Simultaneous voltammetric detection of ascorbic acid and uric acid at a carbon-paste modified electrode incorporating thionine–nafion ion-pair as an electron mediator. Electrochim. Acta. 2006;51:2599-606.
-
21.
Ghalkhani M, Beheshtian J, Salehi M. Electrochemical and DFT study of an anticancer and active anthelmintic drug at carbon nanostructured modified electrode. Mater. Sci. Eng. C. 2016;69:1345-53.
-
22.
Cesarino I, Galesco HV, Machado ASA. Determination of serotonin on platinum electrode modified with carbon nanotubes/polypyrrole/silver nanoparticles nanohybrid. Mater. Sci. Eng. C. 2014;40:49-54.
-
23.
Dogrukol-Ak D, Zaimoglu V, Tunçel M. Voltammetry of trazodone by platinum electrode and its determination in tablets using DP technique in the rotating condition. Eur. J. Pharm. Sci. 1999;7:215-20. [PubMed ID: 9845808].
-
24.
Bonfil Y, Brand M, Kirowa-Eisner E. Trace determination of mercury by anodic stripping voltammetry at the rotating gold electrode. Anal. Chim. Acta. 2000;424:65-76.
-
25.
Zhao G, Wang H, Liu G. Recent advances in chemically modified electrodes, microfabricated devices and injection systems for the electrochemical detection of heavy metals: A review. Int. J. Electrochem. Sci. 2017;12:8622-41.
-
26.
Radi AE. Recent updates of chemically modified electrodes in pharmaceutical analysis. Comb. Chem. High Throughput Screen. 2010;13:728-52. [PubMed ID: 20426739].
-
27.
Chillawar RR, Tadi KK, Motghare RV. Voltammetric techniques at chemically modified electrodes. J. Anal. Chem. 2015;70:399-418.
-
28.
Sanghavi BJ, Wolfbeis OS, Thomas Hirsch T, Nathan S, Swami NS. Nanomaterial-based electrochemical sensing of neurological drugs and neurotransmitters. Microchim. Acta. 2015;182:1-41.
-
29.
Heli H. Amperometric determination of ascorbic acid in pharmaceutical formulations by a reduced graphene Oxide-cobalt hexacyanoferrate nanocomposite. Iran. J. Pharm. Res. 2015;14:453-63. [PubMed ID: 25901152].
-
30.
Shahrokhian S, Hosseini-Nassab N, Ghalkhani M. Construction of Pt nanoparticle-decorated graphene nanosheets and carbon nanospheres nanocomposite-modified electrodes: application to ultrasensitive electrochemical determination of cefepime. RSC Adv. 2014;4:7786-94.
-
31.
Abi A, Mohammadpour Z, Zuo X, Safavi A. Nucleic acid-based electrochemical nanobiosensors. Biosens. Bioelectron. 2018;102:479-89. [PubMed ID: 29195218].
-
32.
Lima HRS, da Silva JS, de Oliveira Farias EA, Teixeira PRS, Eiras C, Cunha Nunes eLC. Electrochemical sensors and biosensors for the analysis of antineoplastic drugs. Biosens. Bioelectron. 2018;108:27-37. [PubMed ID: 29494885].
-
33.
Banks CE, Richard G, Compton RG. New electrodes for old: from carbon nanotubes to edge plane pyrolytic graphite. Analyst. 2006;131:15-21. [PubMed ID: 16425467].
-
34.
Ni Y, Xu J, Liu H, Shao S. Fabrication of RGO-NiCo2O4 nanorods composite from deep eutectic solvents for nonenzymatic amperometric sensing of glucose. Talanta. 2018;185:335-43. [PubMed ID: 29759209].
-
35.
Sebastian M, Mathew B. Ion imprinting approach for the fabrication of an electrochemical sensor and sorbent for lead ions in real samples using modified multiwalled carbon nanotubes. J. Mater. Sci. 2018;53:3557-72.
-
36.
Bijad M, Karimi-Maleh H, Farsi M, Shahidi SA. An electrochemical-amplified-platform based on the nanostructure voltammetric sensor for the determination of carmoisine in the presence of tartrazine in dried fruit and soft drink samples. J. Food Meas. Charact. 2018;12:634-40.
-
37.
Wu JF, Miao-QingXu MQ, Zhao GC. Graphene-based modified electrode for the direct electron transfer of Cytochrome c and biosensing. Electrochem. Commun. 2010;12:175-7.
-
38.
Chand R, Neethirajan S. Microfluidic platform integrated with graphene-gold nano-composite aptasensor for one-step detection of norovirus. Biosens. Bioelectron. 2017;98:47-53. [PubMed ID: 28649024].
-
39.
Aghili Z, Nasirizadeh N, Divsalar A, Shoeibi S, Yaghmaei P. A nanobiosensor composed of Exfoliated Graphene Oxide and Gold Nano-Urchins, for detection of GMO products. Biosens. Bioelectron. 2017;95:72-80. [PubMed ID: 28414950].
-
40.
Suh DH, Park SK, Nakhanivej P, Kim Y, Hwang SM, Park HS. Hierarchically structured graphene-carbon nanotube-cobalt hybrid electrocatalyst for seawater battery. J. Power Sources. 2017;372:31-7.
-
41.
Kang Z, Jiao K, Cheng J, Peng R, Jiao S, Hu Z. A novel three-dimensional carbonized PANI1600@CNTs network for enhanced enzymatic biofuel cell. Biosens. Bioelectron. 2018;101:60-5. [PubMed ID: 29040915].
-
42.
Zheng D, Yanga G, Zheng Y, Fan P, Zhang W, Yu J. Carbon nano-onions as a functional dopant to modify hole transporting layers for improving stability and performance of planar perovskite solar cells. Electrochim. Acta. 2017;247:548-57.
-
43.
Inoue I, Yamauchi H, Okamoto N, Toyoda K, Horita M, Ishikawa Y, Yasueda H, Uraoka Y, Yamashita I. Thermo-stable carbon nanotube-TiO2 nanocompsite as electron highways in dye-sensitized solar cell produced by bio-nano-process. Nanotechnology. 2015;26:285601. [PubMed ID: 26112188].
-
44.
Chen SH, Wu CH, Fang A, Lin CK. Effects of adding different morphological carbon nanomaterials on supercapacitive performance of sol-gel manganese oxide films. Ceram. Int. 2016;42:4797-805.
-
45.
Atchudan R, Edison TNJI, Perumal S, Lee YR. Green synthesis of nitrogen-doped graphitic carbon sheets with use of Prunus persica for supercapacitor applications. Appl. Surf. Sci. 2017;393:276-86.
-
46.
Amreen K, Nisha S, Senthil Kumar A. Undiluted human whole blood uric acid detection using a graphitized mesoporous carbon modified electrode: A potential tool for clinical point-of-care uric acid diagnosis. Analyst. 2018;143:1560-67.
-
47.
Power AC, Gorey B, Chandra S, Chapman J. Carbon nanomaterials and their application to electrochemical sensors: A review. Nanotechnol. Rev. 2018;7:19-41.
-
48.
Walcarius A. Recent trends on electrochemical sensors based on ordered mesoporous carbon. Sensors (Basel). 2017;17:1863-905.
-
49.
Maduraiveeran G, Jin W. Nanomaterials based electrochemical sensor and biosensor platforms for environmental applications. Trends Environ. Anal. Chem. 2017;13:10-23.
-
50.
Ghalkhani M, Shahrokhian S. Application of carbon nanoparticle/chitosan modified electrode for the square-wave adsorptive anodic striping voltammetric determination of Niclosamide. Electrochem. Commun. 2010;12:66-9.
-
51.
Shahrokhian S, Jokar E, Ghalkhani M. Electrochemical determination of piroxicam on the surface of pyrolytic graphite electrode modified with a film of carbon nanoparticle-chitosan. Microchim. Acta. 2010;170:141-6.
-
52.
Sharma VV, Gualandi I, Vlamidis Y, Tonelli D. Electrochemical behavior of reduced graphene oxide and multi-walled carbon nanotubes composites for catechol and dopamine oxidation. Electrochim. Acta. 2017;246:415-23.
-
53.
Mazloum-Ardakani M, Rajabzadeh N, Dehghani-Firouzabadi A, Benvidi A, Mirjalili BBF, Zamani L. Development of an electrode modified on the basis of carbon nanoparticles and reduced graphene oxide for simultaneous determination of isoproterenol, uric acid and tryptophan in real samples. J. Electroanal. Chem. 2016;760:151-7.
-
54.
Gholivand MB, Akbari A. A novel and high sensitive MWCNTs-nickel carbide/hollow fiber-pencil graphite modified electrode for in situ ultra-trace analysis of bisphenol A. J. Electroanal. Chem. 2018;817:9-17.
-
55.
Mekassa B, Tessema M, Chandravanshi BS. Simultaneous determination of caffeine and theophylline using square wave voltammetry at poly(L-aspartic acid)/functionalized multi-walled carbon nanotubes composite modified electrode. Sens. Biosensing Res. 2017;16:46.
-
56.
Kong D, Jiang L, Liu Y, Wang Z, Han L, Lv R, Lin J, Lu CH, Chi Y, Chen G. Electrochemical investigation and determination of procaterol hydrochloride on poly(glutamic acid)/carboxyl functionalized multiwalled carbon nanotubes/polyvinyl alcohol modified glassy carbon electrode. Talanta. 2017;174:436-43. [PubMed ID: 28738604].
-
57.
Shahrokhian S, Ghalkhani M. Electrochemical study of Azathioprine at thin carbon nanoparticle composite film electrode. Electrochem. Commun. 2009;11:1425-8.
-
58.
Ndamanisha JC, Guo L, Wang G. Mesoporous carbon functionalized with ferrocenecarboxylic acid and its electrocatalytic properties. Microporous Mesoporous Mater. 2008;113:114-21.
-
59.
Walcarius A. Electrocatalysis, sensors and biosensors in analytical chemistry based on ordered mesoporous and macroporous carbon-modified electrodes. Trends Anal. Chem. 2012;38:79-97.
-
60.
Gautam V, Singh KP, Yadav VL. Polyaniline/MWCNTs/starch modified carbon paste electrode for non-enzymatic detection of cholesterol: application to real sample (cow milk). Anal. Bioanal. Chem. 2018;410:2173-81. [PubMed ID: 29387950].
-
61.
Tamaddon A, Asghari A. Potentiometric determination of salbutamol using carbon paste electrode assisted with multi-walled carbon nanotubes. Anal. Bioanal. Electrochem. 2018;10:230-8.
-
62.
Erady V, Mascarenhas RJ, Satpati AK, Detriche S, Mekhalif Z, Delhalle J, Dhason A. A novel and sensitive hexadecyltrimethylammoniumbromide functionalized Fe decorated MWCNTs modified carbon paste electrode for the selective determination of Quercetin. Mater. Sci. Eng. C. 2017;76:114-22.
-
63.
Akbari Chermini S, Krimi H, Keyvanfard M, Alizad K. Voltammmetric determination of captopril using multiwall carbon nanotubes paste electrode in the presence of isoproterenol as a mediator. Iran. J. Pharm. Res. 2016;15:107-17. [PubMed ID: 27610151].
-
64.
Safari F, Keyvanfard M, Karimi-Maleh H, Alizad K. voltammetric determination of penicillamine using a carbon paste electrode modified with multiwall carbon nanotubes in the presence of methyldopa as a mediator. Iran. J. Pharm. Res. 2017;16:1019-29. [PubMed ID: 29201090].
-
65.
Shahrokhian S, Ghorbani-Bidkorbeh F, Mohammadi A, Dinarvan R. Electrochemical determinations of 6-mercaptopurine on the surface of a carbon nanotube-paste electrode modified with a cobalt salophen complex. J. Solid State Electrochem. 2012;16:1643-50.
-
66.
Li S, Lei S, Yu Q, Zou L, Ye B. A novel electrochemical sensor for detecting hyperin with a nanocomposite of ZrO2-SDS-SWCNTs as decoration. Talanta. 2018;185:453-60. [PubMed ID: 29759227].
-
67.
Gautam V, Singh KP, Yadav VL. Preparation and characterization of green-nano-composite material based on polyaniline, multiwalled carbon nanotubes and carboxymethyl cellulose: for electrochemical sensor applications. Carbohyd. Polym. 2018;189:218-28.
-
68.
Ebrahimi M, Nikoofard H, Faridbod F, Shiralizadeh Dezfuli A, Beigizadeh H, Parviz Norouzi P. A ceria NPs decorated graphene nano-composite sensor for sulfadiazine determination in pharmaceutical formulation. J. Mater. Sci. Mater. Electron. 2017;28:16704-12.
-
69.
Abdel-Haleem FM, Rizk MS, Badr IHA. potentiometric determination of ciprofloxacin in physiological fluids using carbon paste and nano-composite carbon paste electrodes. Electroanalysis. 2017;29:1172-9.
-
70.
Ensafi AA, Allafchian AR, Rezaei B, Mohammadzadeh R. Characterization of carbon nanotubes decorated with NiFe2O4 magnetic nanoparticles as a novel electrochemical sensor: Application for highly selective determination of sotalol using voltammetry. Mater. Sci. Eng. C. 2013;33:202-8.
-
71.
Tursynbolat S, Bakytkarim Y, Huang J, Wang L. Ultrasensitive electrochemical determination of metronidazole based on polydopamine/carboxylic multi-walled carbon nanotubes nanocomposites modified GCE. J. Pharm. Anal. 2018;8:124-30. [PubMed ID: 29736299].
-
72.
Gholivand MB, Ahmadi E, Haseli M. A novel voltammetric sensor for nevirapine, based on modified graphite electrode by MWCNs/poly(methylene blue)/gold nanoparticle. Anal. Biochem. 2017;527:4-12. [PubMed ID: 28366640].
-
73.
Mohamed MA, Yehia AM, Banks CE, Allam NK. Novel MWCNTs/graphene oxide/pyrogallol composite with enhanced sensitivity for biosensing applications. Biosens. Bioelectron. 2017;89:1034-41. [PubMed ID: 27818046].
-
74.
El-Desoky HS, Ghoneim MM, El-Badawy FM. Carbon nanotubes modified electrode for enhanced voltammetric sensing of mebeverine hydrochloride in formulations and human serum samples. J. Electrochem. Soc. 2017;164:B212-B222.
-
75.
Cheraghi S, Taher MA, Karimi-Maleh H. A sensitive amplified sensor based on improved carbon paste electrode with 1-methyl-3-octylimidazolium tetrafluoroborate and ZnO/CNTs nanocomposite for differential pulse voltammetric analysis of raloxifene. Appl. Surf. Sci. 2017;420:882-5.
-
76.
Sangamithirai D, Munusamy S, Narayanan V, Stephen A. Tunable poly(o-anisidine)/carbon nanotubes nanocomposites as an electrochemical sensor for the detection of an anthelmintic drug mebendazole. Polym. Bull. 2018;75:3127-47.
-
77.
Asadian E, Shahrokhian S, Iraji Zad A, Ghorbani-Bidkorbeh F. Glassy carbon electrode modified with 3D graphene–carbon nanotube network for sensitive electrochemical determination of methotrexate. Sens. Actuat. B-Chem. 2017;239:617-27.
-
78.
Khodadadian M, Jalili R, Bahrami MT, Bahrami G. Adsorptive behavior and voltammetric determination of hydralazine hydrochloride at a glassy carbon electrode modified with multiwalled carbon nanotubes. Iran. J. Pharm. Res. 2017;16:1312-9. [PubMed ID: 29552043].
-
79.
Karim-Nezhad G, Sarkary A, Khorablou Z, Dorraji PS. Synergistic effect of ZnO nanoparticles and carbon nanotube and polymeric film on electrochemical oxidation of acyclovir. Iran. J. Pharm. Res. 2018;17:52-2. [PubMed ID: 29755538].
-
80.
Mani V, Govindasamy M, Chen SM, Karthik R, Huang ST. Determination of dopamine using a glassy carbon electrode modified with a graphene and carbon nanotube hybrid decorated with molybdenum disulfide flowers. Microchim. Acta. 2016;183:2267-75.
-
81.
Shahrokhian S, Salimian R. Ultrasensitive detection of cancer biomarkers using conducting polymer/electrochemically reduced graphene oxide-based biosensor: Application toward BRCA1 sensing. Sens. Actuat. B-Chem. 2018;266:160-9.
-
82.
Piovesan JV, Santana ER, Spinelli A. Reduced graphene oxide/gold nanoparticles nanocomposite-modified glassy carbon electrode for determination of endocrine disruptor methylparaben. J. Electroanal. Chem. 2018;813:163-70.
-
83.
Vasilescu A, Ye R, Boulahneche S, Lamraoui S, Jijie R, Medjram MS, Gáspár S, Singh SK, Kurungot S, Melinte S, Boukherroub R, Szunerits S. Porous reduced graphene oxide modified electrodes for the analysis of protein aggregation Part 2: Application to the analysis of calcitonin containing pharmaceutical formulation. Electrochim. Acta. 2018;266:364-72.
-
84.
Gao Y, Wu X, Wang H, Lu W, Guo M. Highly sensitive detection of hesperidin using AuNPs/rGO modified glassy carbon electrode. Analyst. 2018;143:297-303.
-
85.
AlAqad KM, Suleiman R, Al Hamouz OCS, Saleh TA. Novel graphene modified carbon-paste electrode for promazine detection by square wave voltammetry. J. Mol. Liq. 2018;252:75-82.
-
86.
Nigović B, Jurić S, Mornar A. Electrochemical determination of nepafenac topically applied nonsteroidal anti-inflammatory drug using graphene nanoplatelets-carbon nanofibers modified glassy carbon electrode. J. Electroanal. Chem. 2018;817:30-5.
-
87.
Tavakkoli N, Soltani N, Salavati H, Talakoub M. New carbon paste electrode modified with graphene/TiO2/V2O5 for electrochemical measurement of chlorpromazine hydrochloride. J. Taiwan Inst. Chem. Eng. 2018;83:50-8.
-
88.
Uygun ZO, Şahin C, Yılmaz M, Akçay Y, Akdemir A, Sağın F. Fullerene-PAMAM(G5) composite modified impedimetric biosensor to detect Fetuin-A in real blood samples. Anal. Biochem. 2018;542:11-5. [PubMed ID: 29158128].
-
89.
Soltani N, Tavakkoli N, Mosavimanesh ZS, Davar F. Electrochemical determination of naproxen in the presence of acetaminophen using a carbon paste electrode modified with activated carbon nanoparticles. C. R. Chim. 2018;21:54-60.
-
90.
Sathisha TV, Kumara Swamy BE, Schell M, Eswarappa B. Synthesis and characterization of carbon nanoparticles and their modified carbon paste electrode for the determination of dopamine. J. Electroanal. Chem. 2014;720-721:1-8.
-
91.
Ghorbani-Bidkorbeh F, Shahrokhian S, Mohammadi Ali, Dinarvand R. Simultaneous voltammetric determination of tramadol and acetaminophen using carbon nanoparticles modified glassy carbon electrode. Electrochim. Acta. 2010;55:2752-9.
-
92.
Sudhakara Prasad K, Chuang MC, Annie Ho JA. Synthesis, characterization, and electrochemical applications of carbon nanoparticles derived from castor oil soot. Talanta. 2012;88:445-9. [PubMed ID: 22265524].
-
93.
Yao S, Hua Y, Li G, Zhang Y. Adsorption behavior of ractopamine on carbon nanoparticle modified electrode and its analytical application. Electrochim. Acta. 2012;77:83-8.
-
94.
Szot K, Joensson-Niedziolka M, Rozniecka E, Marken F, Opallo M. Direct electrochemistry of adsorbed proteins and bioelectrocatalysis at film electrode prepared from oppositely charged carbon nanoparticles. Electrochim. Acta. 2013;89:132-8.
-
95.
Shahrokhian S, Naderi L, Ghalkhani M. Nanocellulose/carbon nanoparticles nanocomposite film modified electrode for durable and sensitive electrochemical determination of metoclopramide. Electroanalysis. 2015;27:2637-44.
-
96.
Ghalkhani M, Shahrokhian S. Development of a Nanocellulose composite based voltammetric sensor for vitamin b9 analysis. Curr. Nanosci. 2016;12:493-9.
-
97.
Shahrokhian S, Ghalkhani M, Balotf H, Salimian R. Application of glassy carbon electrode modified with a carbon nanoparticle/melamine thin film for voltammetric determination of raloxifene. J. Electroanal. Chem. 2016;780:126-33.
-
98.
Shahrokhian S, Ghalkhani M, Kohansal R, Mohammadi R. Biomimetic sensor for dobutamine employing Nano-TiO2/Nafion/Carbon nanoparticles modified electrode. Electroanalysis. 2015;28:970-8.
-
99.
Wong A, Santos AM, Fatibello-Filho O. Simultaneous determination of paracetamol and levofloxacin using a glassy carbon electrode modified with carbon black, silver nanoparticles and PEDOT:PSS film. Sens. Actuat. B-Chem. 2018;255:2264-73.
-
100.
Smajdor J, Piech R, Ławrywianiec M, Paczosa-Bator B. Glassy carbon electrode modified with carbon black for sensitive estradiol determination by means of voltammetry and flow injection analysis with amperometric detection. Anal. Biochem. 2018;544:7-12. [PubMed ID: 29258828].
-
101.
Sornambikai S, Abdul Kadir MR, Kumar AS, Ponpandian N, Viswanathan C. Selective and low potential electrocatalytic oxidation and sensing of l-cysteine using metal impurity containing carbon black modified electrode. Anal. Methods. 2017;9:6791-800.
-
102.
Smajdor J, Piech R, Piek M, Paczosa-Bator B. Sensitive voltammetric determination of ethinyl estradiol on carbon black modified electrode. J. Electrochem. Soc. 2017;164:H885-H889.
-
103.
Algarra M, González-Calabuig A, Radotić K, Mutavdzic D, Ania CO, Lázaro-Martíneze JM, Jiménez-Jiménez J, Rodríguez-Castellón E, del Valle M. Enhanced electrochemical response of carbon quantum dot modified electrodes. Talanta. 2018;178:679-85. [PubMed ID: 29136880].
-
104.
He S, He P, Zhang X, Zhang X, Li C, Dong F, Lei H, Liu D. Poly(bromocresol green)/carbon quantum dots modified electrode for the simultaneous electrochemical determination of guanine and adenine. J. Electroanal. Chem. 2017;806:158-65.
-
105.
Chen J, He P, Bai H, He S, Zhang T, Zhang X, Dong F. Poly(Β-cyclodextrin)/carbon quantum dots modified glassy carbon electrode: Preparation, characterization and simultaneous electrochemical determination of dopamine, uric acid and tryptophan. Sens. Actuat. B-Chem. 2017;252:9-16.
-
106.
Shahrokhian S, Kohansal R, Ghalkhani M, Amini MK. Electrodeposition of copper oxide nanoparticles on pre-casted carbon nanoparticles film for electrochemical investigation of anti-HIV drug, nevirapine. Electroanalysis. 2015;27:1989-97.
-
107.
Ghalkhani M, Salehi M. Electrochemical sensor based on multi-walled carbon nanotubes–boehmite nanoparticle composite modified electrode. J. Mater. Sci. 2017;52:12390-400.
-
108.
Shahrokhian S, Ghalkhani M. Glassy carbon electrodes modified with a film of nanodiamond-graphite/chitosan: application to the highly sensitive electrochemical determination of azathioprine. Electrochim. Acta. 2010;55:3621-27.
-
109.
Yin H, Zhu J, Chen J, Gong J, Nie Q. MOF-derived in situ growth of carbon nanotubes entangled Ni/NiO porous polyhedrons for high performance glucose sensor. Mater. Lett. 2018;221:267-70.
-
110.
Pham TSH, Mahon PJ, Lai G, Yu A. Reduced graphene oxide nanocomposite modified electrodes for sensitive detection of ciprofloxacin. Electroanalysis. 2018;30:2185-94.
-
111.
He Q, Liu J, Liu X, Li G P, Liang J. Preparation of Cu2O-reduced graphene nanocomposite modified electrodes towards ultrasensitive dopamine detection. Sensors. 2018;18:199-211.
-
112.
Mohammed GI, Khraibah NH, Bashammakh AS, El-Shahawi MS. Electrochemical sensor for trace determination of timolol maleate drug in real samples and drug residues using Nafion/carboxylated-MWCNTs nanocomposite modified glassy carbon electrode. Microchem. J. 2018;143:474-83.
-
113.
Sharma S, Singh N, Tomar V, Chandra R. A review on electrochemical detection of serotonin based on surface modified electrodes. Biosens. Bioelectron. 2018;107:76-93. [PubMed ID: 29448224].
-
114.
Shahrokhian S, Hafezi-Kahnamouei M. Glassy carbon electrode modified with a nanocomposite of multi-walled carbon nanotube decorated with Ag nanoparticles for electrochemical investigation of Isoxsuprine. J. Electroanal. Chem. 2018;825:30-9.
-
115.
Ibáñez-Redín G, Wilson D, Gonçalves D, Oliveira Jr ON. Low-cost screen-printed electrodes based on electrochemically reduced graphene oxide-carbon black nanocomposites for dopamine, epinephrine and paracetamol detection. J. Colloid Interf. Sci. 2018;515:101-8.
-
116.
Han D, Han T, Shan C, Ivaska A, Niu L. Simultaneous determination of ascorbic acid, dopamine and uric acid with chitosan-graphene modified electrode. Electroanalysis. 2010;22:2001-8.
-
117.
Kim YR, Bong S, Kang YJ, Yang Y, Mahajan RK, Kim JS, Kim H. Electrochemical detection of dopamine in the presence of ascorbic acid using graphene modified electrodes. Biosens. Bioelectron. 2010;25:2366-9. [PubMed ID: 20307965].
-
118.
Sun CL, Chang CT, Lee HH, Zhou J, Wang J, Sham TK, Pong WF. Microwave-assisted synthesis of a Core–Shell MWCNT/GONR heterostructure for the electrochemical detection of ascorbic acid, dopamine, and uric acid. ACS Nano. 2011;5:7788-95. [PubMed ID: 21910421].
-
119.
Beitollahi H, Dourandish A, Ganjali MR, Shakeri S. Voltammetric determination of dopamine in the presence of tyrosine using graphite screen-printed electrode modified with grapheme quantum dots. Ionics. 2018;24:4023-31.
-
120.
Oh JW, Jihye Heo J, Kim TH. An electrochemically modulated single-walled carbon nanotube network for the development of a transparent flexible sensor for dopamine. Sens. Actuat. B. 2018;267:438-47.
-
121.
Asadian E, Shahrokhian S, Iraji Zad A. Highly sensitive nonenzymetic glucose sensing platform based on MOF-derived NiCo LDH nanosheets/graphene nanoribbons composite. J. Electroanal. Chem. 2018;808:114-23.