Abstract
Keywords
Introduction
Abuses of METH, as a neurostimulator agent, has been increased in recent years (1, 2). The consequences of chronic use of METH and its biochemical and behavioral effects remain unclear (3, 4). METH causes increase in release of dopamine, norepinephrine into synaptic terminals (4, 5). It causes hyper-stimulation of receptors in the acute phases and also down regulation of its receptor in chronic phase (4, 5). METH action, pharmacologically, is similar to cocaine and this similarity causes high potential of abuse and addiction (5). Chronic abuses of METH can induce behavioral changes such as anxiety and depression-like behavior and also cognition (learning and memory) impairment in rodent experimental models (6, 7). Experimental studies have confirmed the potential effect of METH in neurodegeneration of some areas of the brain such as the hippocampus, responsible for cognition and anxiety (7). Previous studies have demonstrated that METH abuse can lead to production of apoptotic proteins like Bax, caspase-3, 8 and 9 and therefore, it causes DNA fragmentation in some brain regions, such as hippocampus and amygdala (8, 9). METH and other neuro-stimulant compounds can cause inflammation , oxidative stress and mitochondrial dysfunction in brain cells, but it’s putative mechanism remains unknown (10, 11). Interestingly, METH-induced neurotoxicity appears to be more pronounced in some brain regions like hippocampus (CA1, CA2, CA3, and DG regions) and amygdala (11-13).
During recent years, using herbal/natural compounds with therapeutic probability have been amazingly increased. Natural flavonoids and their derivatives are being extensively considered as therapeutic agents against neurodegenerative diseases and some Neuro-disorders induced by drug abuse (14, 15). Crocin is a carotenoid chemical compound that is found in the flowers crocus and gardenia (16-18). Crocin is the ingredient which is primarily responsible for the color of saffron (17, 19 and 20). Crocin has been shown to be an antioxidant, and neural protective agent (19, 21 and 22). The antioxidant behavior of crocin is related to the sugar moiety in crocin molecule which has a vital role in its chemical reactivity (19). Also, crocin has possible antidepressant properties in mice and humans (23).
It exerts biological effects through its antioxidant, anti-inflammatory, antiapoptotic, and immunomodulatory activities (23-25). Crocin treatment has shown to counteract oxidative stress by reducing lipid peroxidation and improving the activity of antioxidant enzymes like superoxide dismutase (SOD) and catalase in some neurodegenerative disorder (24, 26). Furthermore, chronic treatment with crocin reduces the alcohol-induced rise in TNF-α and IL-1β, levels (27). All these properties may contribute to therapeutic potential efficacy of crocin in neurodegenerative disorders of drug abusers, but it’s exact mechanism remains unclear (27-29). Cyclic AMP response element binding protein (CREB) is a chief transcription factor which is involved in regulation of genes associated with synaptic and survival of neurones, neuroprotection and neural plasticity such as Brain-derived neurotrophic factor (BDNF) (30, 31). BDNF is an important neurotrophic factor which primarily supports the growth and survival of neurons. It is highly expressed in some brain areas that are known to regulate cognition, emotions, and rewards (17, 32). It is suggested that crocin may protect hippocampal and frontal neurons against stress-induced damage via up- regulation of CREB and BDNF, but this concept was not approved definitely. Thus, we designed this study to assess whether crocin confers neuroprotection against METH-induced hippocampal damage, and to determine the role of P-CREB-BDNF signaling pathway in this protection.
Experimental
Animals
Sixty adult Wistar male rats, weighing between 250–300 g, were purchased from lab house of Iran University of Medical Sciences. They were kept under controlled condition, room temperature (22 ± 0.5 °C) with 12-h light/dark cycle and had free access to food and water. Our experiment protocol was approved by the Committee of Research in Ethics by the Iran University of Medical Science (Research code: 98-1-37-14561).
Effects of various doses of crocin on mitochondrial GSH and GSSG content in METH treated rats
Group | Mean ± SEM GSH (nmol/mg protein) | GSSG (nmol/mg protein) | GSH/GSSG |
---|---|---|---|
Control group | 62.7 ± 4.1 | 0.98 ± 0.2 | 76 |
METH (10 mg/kg) | 40.5 ± 4.1a | 5.2 ± 1.1a | 11a |
METH + Crocin (10 mg/kg) | 46.5 ± 3.4 | 4.9 ± 0.04 | 15.3 |
METH + Crocin (20 mg/kg) | 50.4 ± 5 | 4.69 ± 0.14 | 17.04 |
METH + Crocin (40 mg/kg) | 56.3 ± 4.3b | 3.5 ± 0.12b | 36.3b |
METH + Crocin (80 mg/kg) | 58.2 ± 2.8b | 3.5 ± 0.10b | 42.6b |
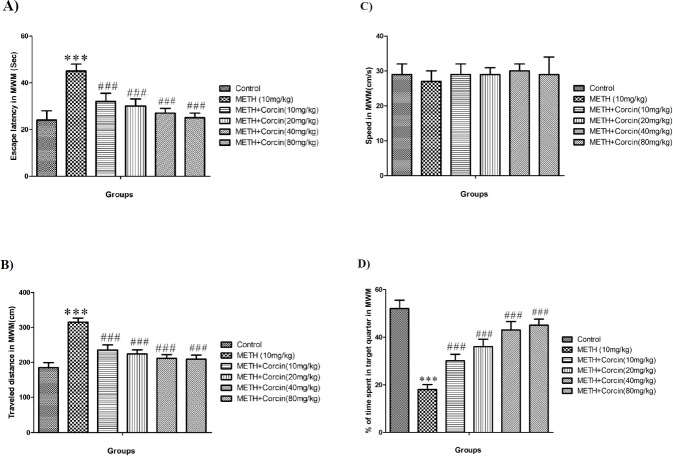
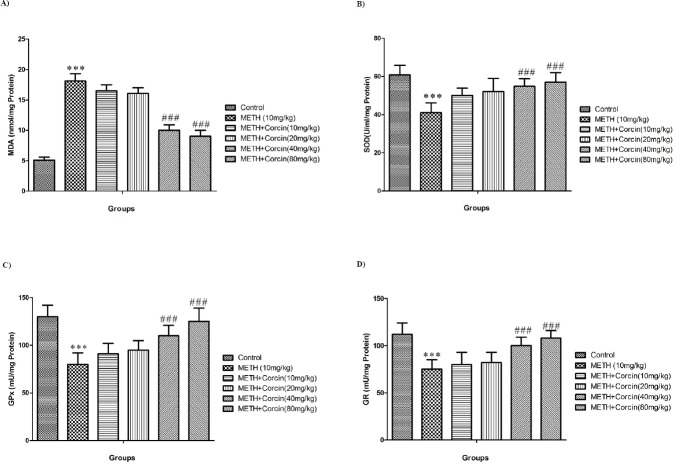
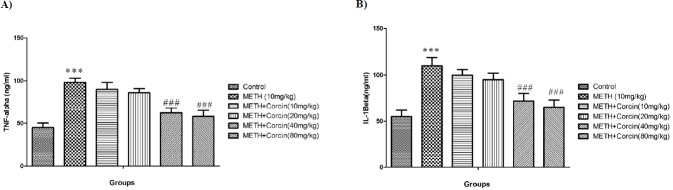
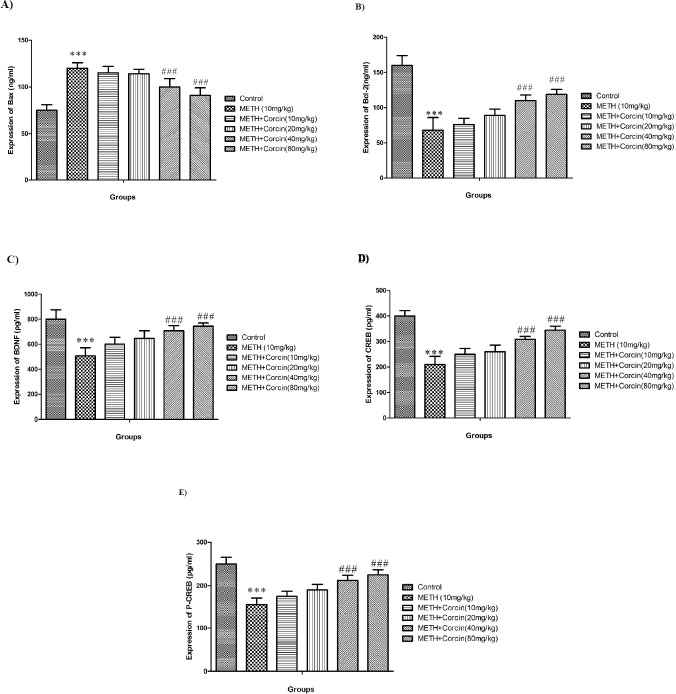
Drug
Crocin and METH were purchased from Sigma-Aldrich (USA) and dissolved freshly in normal saline just before administration.
Experimental design
Group 1 (control group) were administrated with normal saline (0.7 mL/rat, i.p) for 21 days and Group 2 (METH) received METH (10 mg/kg, i.p) for 21 days.
Groups 3, 4, and 5 concurrently were treated by METH (10 mg/kg, i.p) and crocin with a dosage of 10, 20, 40, and 80 mg/kg, i.p., respectively for 21 days. It should be mentioned that in these groups the administration of crocin was done first and after one hour the METH was administrated.
During the 17th and 21st day, morris water maze (MWM) task, a standard behavioral method for evaluation of learning and spatial memory, was performed. After 22nd day, all animals were sacrificed and parameters for oxidative stress, inflammation, and apoptosis were evaluated in hippocampal tissues. Keeping in view the importance of CREB signaling and its product, BDNF, the effect of crocin on METH-induced disturbances in the CREB signaling pathway was studied in hippocampal tissues (33-35).
Behavioral method
Morris water maze task (MWM)
MWM apparatus includes a black colored circular tank, filled with water, 160 cm in diameter and 90 cm in height, which was fixed in the center of the experimental lab. This equipment was divided into four quadrants (North, East, West, and South) and was filled with water to the height of 50 cm. The operator stays in the North-East part of the room. A disk on the platform with 15 cm diameter, which was hidden, was located 1 cm beneath the surface of the water. In the first 4 days of the experiment, called training procedure, mentioned platform was randomly inserted persistently in one of the quarter. An automated infrared tracking system (CCTV B/W camera, SBC-300 (P), Samsung Electronics Co, Ltd, Korea) recorded the position of the experimented animal in the tank. The camera was mounted 2.4 m above the surface of the water (36, 37).
A) Handling
On the first day before the start of the experiment, all rats one by one were positioned on the tank that was filled with 40 ºC water, room temperature (25 ± 2 ºC) and the experimenter guided the rat for swimming and to reach to the quarter where platform was placed. In our experiment, the platform was situated on South-East quarter of a tank (36, 37).
B) Training procedure
Some discriminate landmarks (such as a distinguish picture, window, door, etc.) were placed in the extra maze in the room for spatial cues for learning about the platform’s position for animals. As mentioned above, the position of the platform was set up in the South-East quarter of the MWM tank with 25 cm distance from the edge of the tank, and 1 cm beneath the surface of the water. For evaluation of learning procedure, each rat experimented for 4 trials in a day for 4 days. Each animal was randomly located in from four quarters (North, East, West, and South) respectively. During the learning procedure if the rats found the platform within the 60 sec, the trial was automatically closed by a computer, but if they could not reach and find the platform within 60 sec the trial automat was stopped by computer. In learning experiment, two parameters were evaluated: 1) The time of escape latency characterized by time to find the hidden platform. 2) Traveled distance which was confirmed by the distance each animal spent to reach and find the hidden platform.
In memory assessment procedure, on the fifth day (probe day), the platform was removed and the animals were randomly terrified of the water from one of the above-mentioned directions (almost East) and the percentage of presence of the animals in the target quarter (South-East quarter) was recorded and calculated (36-40).
Mitochondrial preparations
The animals were anesthetized using sodium thiopental (50 mg/kg, i.p) and the hippocampus was isolated from each rat. The isolated tissues were homogenized in cold homogenization buffer (25 mM 4-morpholinepropanesulfonic acid, 400 mM sucrose, 4 mM magnesium chloride (MgCl2), 0.05 mM ethylene glycol tetraacetic acid (EGTA), pH 7.3) and the homogenized tissues were centrifuged at 450×g for 10 min. The supernatants obtained were re-centrifuged at 12000×g for 10 min. Finally, the sediments were re-suspended in homogenization buffer and stored at 0 °C. Total mitochondrial proteins in tissues were determined using a Dc protein assay kit (Bio-Rad), (California, USA). Briefly; Bradford reagent (1 part Bradford: 4 parts dH2O) was added to serial dilution series (0.1-1.0 mg/mL) of a known protein sample concentration; e.g., bovine serum albumin (BSA), dissolved in homogenization buffer. These serial dilution series were prepared and used for providing a standard curve. On the other hand, 10, 15, 20, 25, and 30 μL of the protein extract (homogenized cell solutions) were added to multiple wells. Bradford reagent was also added to each well. The density of colors of all wells was read by the plate reader at 630 nm. Finally, by using the standard curve, protein quantity in the extracts was obtained. These homogenized cell solutions, containing mitochondria of hippocampal cells, were analyzed for the measurement of oxidative stress and inflammatory markers (34, 35, 41 and 42).
Measurement of oxidative stress parameters
Determination of lipid peroxidation
For assessment of lipid peroxidation, malondialdehyde (MDA) - a natural by-product was assessed. Briefly, 100 μL of SDS lysis solution was added to wells containing (100 μL) of sample solution or MDA standard. After shaking and incubation of these wells, 250 μL of thiobarbituric acid (TBA) reagent was added to each well and incubated at 95 °C for 45-60 min. Next, the tubes were centrifuged at 1000×g for 15 min and 300 μL of n-Butanol was added to 300 μL of the supernatant. Then, the tubes were centrifuged for 5 min at 10,000×g. Finally, the absorbance was read at 532 nm and the results obtained were expressed as nmol/mg of protein (34, 35 and 41-44).
Determination of GSH (Glutathione) and GSSG (Glutathione disulfide) disulfide
For measuring GSH (Glutathione) and GSSG (Glutathione disulfide) levels, 25 μL of the IX glutathione reductase solution and 25 μL of the IX NADPH solution were added to a 96-well plate containing a standard solution of glutathione or a sample of homogenized solution. Then, 50 μL of the IX Chromogen was added to each well and mixed vigorously. Finally, the absorbance was read at 405 nm for each GSSG/GSH standard and sample. Using the standard curve, the levels of GSSG/GSH were quantified and expressed as nmol/mg of protein (33, 44).
Determination of manganese superoxide dismutase (MnSOD) activity
The previously described method was used to assess SOD activity (31, 33 and 44). SOD activity was measured using the following equation: SOD activity = {[(A blank 1 - A blank 3) – (A sample - A blank 2)] / (A blank 1 - A blank 3)} × 100.
Determination of glutathione peroxidase (GPx) activity
GPx activity was assessed as previously described (31, 33 and 44). It was measured based on a change in absorbance [ΔA340/min] by the following equation:
ΔA340/min = A340nm (Start) – A340nm (Stop) /Reaction time (min), any change in the absorbance is directly proportional to GPx activity.
GPx activity: ΔA340/min × Reaction volume (mL) × Dilution factor of the original sample/Extinction coefficient for NADPH at 340 nm × Volumes of the tested sample. The results were expressed as mU/mg protein (31, 33 and 44).
Determination of glutathione reductase (GR) activity
GR activity was assessed as described previously (31, 33 and 44). It was measured based on a change in absorbance [ΔA340/min] by the following equation:
ΔA340/min = A340nm (Start) – A340nm (Stop)/Reaction time (min), any change in the absorbance is directly proportional to GR activity.
GR activity: ΔA340/min × Reaction volume (mL) × Dilution factor of the original sample/Extinction coefficient for NADPH at 340 nm × Volumes of the tested sample. The results were expressed as mU/mg protein (31, 33 and 44).
Determination of protein expression alteration
Concentrations (expression of protein) of brain-derived neurotrophic factor (BDNF), brain cyclic adenosine monophosphate (cAMP) (CREB) (total and phosphorylated), TNF-α, IL-1β, Bax, and Bcl-2 in cell lysate of hippocampal tissue, were measured by using a commercially available ELISA kit (Genzyme Diagnostics, Cambridge, U.S.A). Briefly, wells containing sheep anti-rat BDNF, CREB (total and phosphorylated), IL-1β, and TNF-α polyclonal antibody (Sigma Chemical Co., Poole, and Dorset, UK) were washed three times with washing buffer (0.5 M of Sodium chloride (NaCl), 2.5 mM sodium dihydrogen phosphate (NaH2PO4), 7.5 mM Na2HPO4, 0.1% Tween 20, pH 7.2). Then, 100 mL of 1% (w/v) ovalbumin (Sigma Chemical Co., Poole, Dorset, UK) solution was added to each well and incubated at 37 °C for 1 h. Following three washes, 100 ml of samples and standards were added to each well and incubated at 48 °C for 20 h. After three washes, 100 mL of the biotinylated sheep anti-rat IL-1β or TNF-α antibody (1:1000 dilutions in washing buffer containing 1% sheep serum, Sigma Chemical Co., Poole, and Dorset, UK) was added to each well. Next, after 1-hour incubation and three washes, 100 mL avidin-HRP (Dako Ltd, UK) (1:5000 dilution in wash buffer) was added to each well and the plate was incubated for 15 min. After washing three times, 100 mL of TMB substrate solution (Dako Ltd., UK) was added to each well and then incubated for 10 min at room temperature. Then, 100 mL of 1M H2SO4 was added and absorbance was read at 450 nm. The results were expressed as ng IL-1β/mL or TNF-α/mL or Bax and Bcl-2 of suspension of hippocampus tissues and about the BDNF and CREB (total and phosphorylated) were reported as pg/mL of suspension of hippocampus tissues (45-48).
Statistical analysis
The data were analyzed by GraphPad PRISM v.6 Software and averaged in every experimental group and expressed as Means ± Standard error of the means (SEM). Then, the differences between control and treatment groups were evaluated by ANOVA. To evaluate the severity of the behaviors, the differences between averages in each group were compared using the Tukey’s post-hoc at a significant level of P < 0.001.
Results
Evaluation of escape latency and traveled distance during training days in the MWM
METH (10 mg/kg) causes significant increase in escape latency (with F (5, 42) = 6.507) and traveled distance (with F (5, 42) = 12.46) during four days training in the MWM when compared to control groups (P < 0.001) (Figures 1A and 1B). While crocin in all doses inhibited METH-induced significant reduction in escape latency (with F (5, 42) = 6.507) and traveled distances (with F (5, 42) = 12.46) as opposed to METH (10 mg/kg) treated group (P < 0.001) (Figures 1A and 1B).
Evaluation of swimming speed during training days
The swimming speed was not altered during training trials in any of the animal groups (with F (5, 42) = 0.097) (Figure 1C).
Evaluation of percentage in target quarter in probe trial
METH, 10 mg/kg, causes significant decrease in the percentage of the presence of animals in target quarter (with F (5, 42) = 16.77) in comparison with control group (P < 0.001) (Figure 1D). Also, crocin in all doses used can significantly diminish METH induced decrease in presence of animals in target quarter (with F (5, 42) = 16.77) (Figure 1D).
Effects of various doses of crocin on METH-induced GSH/GSSG alterations
METH (10 mg/kg) treatment markedly reduced the mitochondrial GSH content, with F (5, 42) = 4.200, while increased the GSSG levels, with F (5, 42) = 11.20, in comparison to the control group (P < 0.001) (Table 1). Conversely, high doses of crocin (40 and 80 mg/kg) improved the GSH-content (with F (5, 42) = 4.200) and reduced the GSSG levels [F (5, 42) = 11.20] in METH-treated animals when compared to the positive control (P < 0.001) (Table 1).
Effects of various doses of crocin on METH-induced alteration in oxidative stress parameters
METH administration significantly increased the MDA levels (with F (5, 42) = 30.19) and reduced the SOD, GPx and GR activity with F (5, 42) = 1.80, F (5, 42) = 2.86 and F (5, 42) = 2.207, respectively when compared to the control group (P < 0.001) (Figures 2A-2D). Conversely, high doses of crocin (40 and 80 mg/kg) inhibit the METH-induced increase in MDA level (with F (5, 42) = 30.19) and decreases in SOD, GPx, and GR activity with F (5, 42) = 1.80, F (5, 42) = 2.86 and F (5, 42) = 2.207 respectively (P < 0.001) (Figures 2A-2D).
Effects of various doses of crocin on METH-induced rise in inflammatory biomarkers
METH, 10 mg/kg, causes significant elevation in level of IL-1β, with F (5, 42) = 8.36, and TNF-α, with F (5, 42) = 11.58, as compared to the control group (P < 0.001) (Figures 3A and 3B). Conversely, high doses of crocin (40 and 80 mg/kg) prevented the METH-induced rise in level of IL-1β, with F (5, 42) = 8.36, and TNF-α, with F (5, 42) = 11.58 when compared to METH only treated group (P < 0.001) (Figures 3A and 3B).
Effects of various doses of crocin on METH-induced changes in Bax and Bcl-2 proteins level
METH (10 mg/kg) treatment increased protein expression of Bax with F (5, 42) = 8.60 and reduced protein expression of Bcl-2, with F (5, 42) = 8.60, when compared to the control group (P < 0.001). Conversely, high doses of crocin (40 and 80 mg/kg) improved Bcl-2 expression while reduced Bax protein expression when compared to the positive controls (F (5, 42) = 8.60) (P < 0.001) (Figures 4A and 4B).
Effects of various doses of crocin on METH-induced alteration in protein expression of both forms of CREB and BDNF
METH (10 mg/kg) treatment markedly reduced the protein expression of BDNF and CREB (total and phosphorylated) with F (5, 42) = 3.53, F (5, 42) = 9.62, and F (5, 42) = 6.75, respectively when compared to control group (P < 0.001) (Figures 4C-4E). Conversely, in METH-dependent aniaml administartion of high doses of crocin (40 and 80 mg/kg) significantly improved the protein expression of BDNF and CREB (total and phosphorylated) [with F (5, 42) = 3.53, F (5, 42) = 9.62 and F (5, 42) = 6.75 respectively] when compared to the METH only treated group (P < 0.001) (Figures 4C-4E).
Discussion
The results of current study demonstrated that various doses of crocin can ameliorate METH-induced neuro-apoptosis, oxidative stress, and inflammation in the rat hippocampus. In addition, it indicated that the protective role of crocin is mediated possibly via P-CREB /BDNF signaling pathway.
METH as a psycho-stimulant agent carries a high potential for abuse and addiction (8, 49). According to our study chronic administration of METH at a dose of 10 mg/kg can increase escape latency and traveled distance in MWM, this data suggested that METH administration can decrease learning activity. Also in probe day, METH administration could decrease percentage of presence in target quarter in MWM. These data suggested that chronic administration of METH can decrease spatial memory. These results confirm the results of previous study indicating chronic administration of METH decreased learning and memory in rats (8, 13). METH caused the release of dopamine, serotonin, and adrenaline in the brain and this releasing caused downregulation of the mentioned amine receptors and consequence of this phenomenon is cognition impairment (13, 50). According to our results, crocin at high doses (40 and 80 mg/kg) could alter the METH-induced cognition impairment. Many previous studies indicated that crocin and other similar herbal compound can improve learning and memory (25, 51).
Our data indicated that administration of METH increases hippocampal MDA level, whereas, crocin treatment (10, 20, 40 and 80 mg/kg) attenuates METH-induced rise in lipid peroxidation in the brain. The inhibitory effect of crocin on MDA level was more significant at higher doses (40 and 80 mg/kg) compared to lower doses (10 and 20 mg/kg) used in this study. These results are similar to previous findings, which indicated METH-induced lipid peroxidation in the brain (8, 52). According to these data, it seems that part of the destructive effects of METH is mediated through mitochondrial dysfunction and probably crocin is somehow modulating this process (24, 53). Furthermore, it has been indicated by previous work that crocin exerts neuroprotective effects by inhibiting the formation of free radicals in neurodegenerative diseases such as Alzheimer (25), and the role of crocin as a scavenger for free radicals is well-evident in this type of disorder (24, 53). Our results indicated that METH (10 mg/kg) decreases mitochondrial GSH content while increasing GSSG level in the hippocampal tissues. Activation of glutathione reduced form (GSH) to the toxic oxidized form (GSSG) by METH is a key change that can start and activate neurodegenerative signals in the brain (54-57), and this mechanism causes harmful effect on glutathione cycle and consequently causes neural cell death (55, 57). Moreover, we found that various doses of crocin, especially 40 and 80 mg/kg, increase GSH content, while reducing GSSG level in animals with METH (10 mg/kg) consumption. These findings have been also reported already by previous studies indicating that crocin, by modulation of glutathione circle, can be therapeutically beneficial against neurodegenerative diseases as it promotes GSH formation (58, 59).
In our study, administration of METH decreased GPx, GR, and SOD activities in isolated hippocampal tissues which confirmed the previous studies report about METH abuse that diminishes antioxidant defenses which may result in neurodegeneration (13, 60). It has been shown that GR is the key enzyme which is responsible for converting the oxidized form of glutathione (GSH) to the reduced form (GSSG) (24). Thus, a METH-induced decrease in GR activity results in elevation of GSSG and reduction of GSH levels as observed in our results. Some novel reports showed that METH consumption causes mitochondrial dysfunction and lead to inhibition of antioxidant enzyme activity in multiple cells, and these properties caused METH-induced degenerative effects on brain cells such as hippocampus (8, 13 and 30). We detected that crocin treatment dose-dependently recovers the activity of antioxidant enzymes. Crocin by activating GR increases the conversion of GSSG to GSH and thus, protects the brain against METH-induced oxidative stress. Previous studies have also incdicated such anti-oxidative properties of crocin in neurodegenerative disorder (59, 61). In addition, our results confirmed the previous findings regarding the decrease in SOD activity following METH abuse (59). Consistent with previous studies, treatment by crocin was found to be effective in reversing the alcohol-induced reduction in SOD activity in the hippocampal tissues (59).
We demonstrated that chronic METH administration significantly increases the level of pro-inflammatory cytokines like IL-β and TNF-α in the hippocampal tissue, whereas, crocin in high doses has a strong potential for suppressing METH-induced neuroinflammation in a dose-dependent manner. Our result is consistent with previous works which have reported the rise of pro-inflammatory cytokines following METH and other psychostimulant agents’ abuse. It has been proposed that METH-induced rise in inflammation is responsible for the neurodegenerative properties of METH (62). On the other hand, crocin has shown to have the therapeutic potential for management of neuroinflammation signaling cascades, thereby protecting the brain against inflammation and its damage (22).
In addition to oxidative stress and inflammation, this study confirms METH-induced apoptosis in the hippocampus. According to the current study, METH administration increased the level of an apoptotic protein, Bax, while decreasing an anti-apoptotic protein, Bcl-2. These data are inconsistent with previous works which have demonstrated that METH abuse can cause brain damage via activation of multiple apoptotic cascades (63, 64). On the other hand, our results demonstrated the anti-apoptotic effect of crocin against METH administration, as indicated by reducing Bax and improved Bcl-2 expressions in the hippocampus. Previous studies demonstrated that crocin treatment attenuates cleaved caspase-3 and production of Bax and nuclear condensation resulting from some neurodegenerative disorder and disease (65).
The anti-inflammatory, anti-apoptotic, and anti-oxidative effects of crocin have been previously reported (22, 65), and were inconsistent with our work, but the involved signaling pathways remain unknown. In this regard, we evaluated the role of the P-CREB-BDNF signaling pathway. Our data demonstrated that METH administration ameliorates CREB (total and phosphorylated) and BDNF protein expression in the hippocampus. In contrast, crocin treatment in high doses enhanced CREB (total and phosphorylated) and BDNF protein expression. Thus, it can be speculated that crocin treatment restores P-CREB-BDNF signaling cascade and protects the brain against METH-induced neurotoxicity. The P-CREB, as a transcription factor, regulates over hundred target genes, especially BDNF, implicated in neuronal regeneration, development, survival, and excitability, addiction, depression and cognition (66). In addition, dysregulation of CREB transcriptional cascade has shown to induce oxidative stress, apoptosis, and neurodegeneration (66, 67). Many previous molecular studies verified that the phosphorylated form of CREB has the main role in many herbal and chemical neuroprotective possessions (67). According to numerous studies, P-CREB (activated form of CREB) causes the creation of BDNF, ligands of TrkB receptor. These works displayed that BDNF by stimulation of its own receptor, TrkB, can inhibits brain cell from degeneration and induces the existence of neurons (68). In the current study, it seems that reduction in P-CREB protein level, by METH, affects the mentioned cascade of BDNF/TrkB signaling pathway and triggers the neurodegeneration, apoptosis, inflammation and oxidative stress. While crocin administration inhibit this property of METH and can trigger cascade of P-CREB/BDNF/ TrkB. Consistent with our results, it has been shown that P-CREB-BDNF signaling pathway has been implicated in modification of several functions in brain such as learning, memory, mood balances, and reward mechanisms (68-71).
Conclusion
Taken together, for the first time, the results of the current study shows that crocin treatment, possibly via stimulation of P-CREB-BDNF signaling pathway, can decline METH-induced apoptosis, oxidative stress and inflammation and might possibly act as a neuroprotective agent against METH induced neurodegeneration. However, further studies regarding human dosage and toxicity are necessary.
References
-
1.
Darke S, Kaye S, McKetin R, Arke S, Kaye S, McKetin R, Duflou J. Major physical and psychological harms of methamphetamine use. Drug Alcohol Rev. 2008;27:253-62. [PubMed ID: 18368606].
-
2.
Barr AM, Panenka WJ, MacEwan GW, Thornton AE, Lang DJ, Honer WG, Lecomte T. The need for speed: an update on methamphetamine addiction. J. Psychiatry Neurosci. 2006;31:301-13. [PubMed ID: 16951733].
-
3.
Krasnova IN, Justinova Z, Ladenheim B, Jayanthi S, McCoy MT, Barnes C, Warner JE, Goldberg SR, Cadet JL. Methamphetamine self-administration is associated with persistent biochemical alterations in striatal and cortical dopaminergic terminals in the rat. PLoS One. 2010;5:e8790. [PubMed ID: 20098750].
-
4.
Volkow ND, Chang L, Wang GJ, Fowler JS, Leonido-Yee M, Franceschi D, Sedler MJ, Gatley SJ, Hitzemann R, Ding YS. Association of dopamine transporter reduction with psychomotor impairment in methamphetamine abusers. Am. J. Psychiatry. 2001;158:377-82. [PubMed ID: 11229977].
-
5.
Thrash B, Karuppagounder SS, Uthayathas S, Suppiramaniam V, Dhanasekaran M. Neurotoxic effects of methamphetamine. Neurochem. Res. 2010;35:171-9. [PubMed ID: 19697126].
-
6.
Brecht ML, O’Brien A, Von Mayrhauser C, Anglin MD. Methamphetamine use behaviors and gender differences. Addict. Behav. 2004;29:89-106. [PubMed ID: 14667423].
-
7.
Narita M, Aoki K, Takagi M, Yajima Y, Suzuki T. Implication of brain-derived neurotrophic factor in the release of dopamine and dopamine-related behaviors induced by methamphetamine. Neuroscience. 2003;119:767-75. [PubMed ID: 12809697].
-
8.
Cadet JL, Jayanthi S, Deng X. Methamphetamine-induced neuronal apoptosis involves the activation of multiple death pathways. Review. Neurotox. Res. 2005;8:199-206. [PubMed ID: 16371314].
-
9.
Wu CW, Ping YH, Yen JC, Chang CY, Wang SF, Yeh CL, Chi CW, Lee HC. Enhanced oxidative stress and aberrant mitochondrial biogenesis in human neuroblastoma SH-SY5Y cells during methamphetamine induced apoptosis. Toxicol. Appl. Pharmacol. 2007;220:243-51. [PubMed ID: 17350664].
-
10.
Zhang X, Banerjee A, Banks WA, Ercal N. N-Acetylcysteine amide protects against methamphetamine-induced oxidative stress and neurotoxicity in immortalized human brain endothelial cells. Brain Res. 2009;1275:87-95. [PubMed ID: 19374890].
-
11.
Jumnongprakhon P, Govitrapong P, Tocharus C, Tocharus J. Melatonin promotes blood-brain barrier integrity in methamphetamine-induced inflammation in primary rat brain microvascular endothelial cells. Brain Res. 2016;1646:182-92. [PubMed ID: 27268413].
-
12.
Yamamoto BK, Raudensky J. The role of oxidative stress, metabolic compromise, and inflammation in neuronal injury produced by amphetamine-related drugs of abuse. J. Neuroimmune Pharmacol. 2008;3:203-17. [PubMed ID: 18709468].
-
13.
Gonçalves J, Baptista S, Martins T, Milhazes N, Borges F, Ribeiro CF, Malva JO, Silva AP. Methamphetamine‐induced neuroinflammation and neuronal dysfunction in the mice hippocampus: preventive effect of indomethacin. Eur. J. Neurosci. 2010;31:315-26. [PubMed ID: 20074221].
-
14.
Kim H. Neuroprotective herbs for stroke therapy in traditional eastern medicine. Neurol. Res. 2005;27:287-301. [PubMed ID: 15845212].
-
15.
Kumar GP, Khanum F. Neuroprotective potential of phytochemicals. Pharmacogn. Rev. 2012;6:81-91. [PubMed ID: 23055633].
-
16.
Hosseinzadeh H, Talebzadeh F. Anticonvulsant evaluation of safranal and crocin from Crocus sativus in mice. Fitoterapia. 2005;76:722-4. [PubMed ID: 16253437].
-
17.
Lee IA, Lee JH, Baek NI, Kim DH. Antihyperlipidemic effect of crocin isolated from the fructus of Gardenia jasminoides and its metabolite crocetin. Biol. Pharm. Bull. 2005;28:2106-10. [PubMed ID: 16272698].
-
18.
Yousefsani BS, Mehri S, Pourahmad J, Hosseinzadeh H. Crocin prevents sub-cellular organelle damage, proteolysis and apoptosis in rat hepatocytes: a justification for its hepatoprotection. Iran. J. Pharm. Res. 2018;17:553-562. [PubMed ID: 29881413].
-
19.
Chen Y, Zhang H, Tian X, Zhao C, Cai L, Liu Y, Jia L, Yin HX, Chen C. Antioxidant potential of crocins and ethanol extracts of Gardenia jasminoides ELLIS and Crocus sativus L: A relationship investigation between antioxidant activity and crocin contents. Food Chem. 2008;109:484-92.
-
20.
Khalili M, Roghani M, Ekhlasi M. The effect of aqueous Crocus sativus L extract on intracerebroventricular streptozotocin-induced cognitive deficits in rat: a behavioral analysis. Iran. J. Pharm. Res. 2010;8:185-91.
-
21.
Mard SA, Azad SM, Ahangarpoor A. Protective effect of crocin on gastric mucosal lesions induced by ischemia-reperfusion injury in rats. Iran. J. Pharm. Res. 2016;15:93-99. [PubMed ID: 28228808].
-
22.
Nam KN, Park YM, Jung HJ, Lee JY, Min BD, Park SU, Ung WS, Cho KH, Park JH, Kang I. Anti-inflammatory effects of crocin and crocetin in rat brain microglial cells. Eur. J. Pharmacol. 2010;648:110-6. [PubMed ID: 20854811].
-
23.
Hosseinzadeh H, Shamsaie F, Mehri S. Antioxidant activity of aqueous and ethanolic extracts of Crocus sativus L stigma and its bioactive constituents, crocin and safranal. Pharmacogn. Mag. 2009;5:419-20.
-
24.
Hosseinzadeh H, Sadeghnia HR, Ziaee T, Danaee A. Protective effect of aqueous saffron extract (Crocus sativus L) and crocin, its active constituent, on renal ischemia-reperfusion-induced oxidative damage in rats. J. Pharm. Pharm. Sci. 2005;8:387-93. [PubMed ID: 16401388].
-
25.
Khalili M, Hamzeh F. Effects of active constituents of Crocus sativus L, crocin on streptozocin-induced model of sporadic Alzheimer›s disease in male rats. Iran. Biomed. J. 2010;14:59-65. [PubMed ID: 20683499].
-
26.
El-Beshbishy HA, Hassan MH, Aly HA, Doghish AS, Alghaithy AA. Crocin “saffron” protects against beryllium chloride toxicity in rats through diminution of oxidative stress and enhancing gene expression of antioxidant enzymes. Ecotoxicol. Environ. Saf. 2012;83:47-54. [PubMed ID: 22766413].
-
27.
El-Maraghy SA, Rizk SM, Shahin NN. Gastroprotective effect of crocin in ethanol-induced gastric injury in rats. Chem. Biol. Interact. 2015;229:26-35. [PubMed ID: 25637687].
-
28.
Tamaddonfard E, Hamzeh‐Gooshchi N. Effect of crocin on the morphine‐induced antinociception in the formalin test in rats. Phytother. Res. 2010;24:410-3. [PubMed ID: 19653196].
-
29.
Firouzi M, Moshayedi P, Sabouni F, Parsa K, Keshavarz M. The effect of crocin (a derivative of Crocus sativus L) on neural development and regeneration of rat: in-vivoand in-vitro study. Iran. J. Pharm. Res. 2010;135:70-9.
-
30.
Motaghinejad M, Motevalian M, Abdollahi M, Heidari M, Madjd Z. Topiramate confers neuroprotection against methylphenidate-induced neurodegeneration in dentate gyrus and CA1 regions of hippocampus via CREB/BDNF pathway in rats. Neurotox. Res. 2017;31:373-99. [PubMed ID: 28078543].
-
31.
Motaghinejad M, Motevalian M, Babalouei F, Abdollahi M, Heidari M, Madjd Z. Possible involvement of CREB/BDNF signaling pathway in neuroprotective effects of topiramate against methylphenidate induced apoptosis, oxidative stress and inflammation in isolated hippocampus of rats: molecular, biochemical and histological evidences. Brain Res. Bull. 2017;132:82-98. [PubMed ID: 28552672].
-
32.
Hattiangady B, Rao MS, Shetty GA, Shetty AK. Brain-derived neurotrophic factor, phosphorylated cyclic AMP response element binding protein and neuropeptide Y decline as early as middle age in the dentate gyrus and CA1 and CA3 subfields of the hippocampus. Exp. Neurol. 2005;195:353-71. [PubMed ID: 16002067].
-
33.
Motaghinejad M, Motevalian M, Falak R, Heidari M, Sharzad M, Kalantari E. Neuroprotective effects of various doses of topiramate against methylphenidate-induced oxidative stress and inflammation in isolated rat amygdala: the possible role of CREB/BDNF signaling pathway. J. Neural Transm (Vienna). 2016;123:1463-77. [PubMed ID: 27665547].
-
34.
Motaghinejad M, Seyedjavadein Z, Motevalian M, Asadi M. The neuroprotective effect of lithium against high dose methylphenidate: possible role of BDNF. Neurotoxicology. 2016;56:40-54. [PubMed ID: 27343358].
-
35.
Motaghinejad M, Karimian SM, Motaghinejad O, Shabab B, Asadighaleni M, Fatima S. The effect of various morphine weaning regimens on the sequelae of opioid tolerance involving physical dependency, anxiety and hippocampus cell neurodegeneration in rats. Fundam. Clin. Pharmacol. 2015;29:299-309. [PubMed ID: 25846801].
-
36.
D’Hooge R, De Deyn PP. Applications of the Morris water maze in the study of learning and memory. Brain Res. Rev. 2001;36:60-90. [PubMed ID: 11516773].
-
37.
Motaghinejad M, Motevalian M, Larijani SF, Khajehamedi Z. Protective effects of forced exercise against methylphenidate-induced anxiety, depression and cognition impairment in rat. Adv. Biomed. Res. 2015;27:134-40.
-
38.
Motaghinejad M, Fatima S, Karimian M, Ganji S. Protective effects of forced exercise against nicotine-induced anxiety, depression and cognition impairment in rat. J. Basic Clin. Physiol. Pharmacol. 2015;27:19-27.
-
39.
Vorhees CV, Williams MT. Morris water maze: procedures for assessing spatial and related forms of learning and memory. Nat. Protoc. 2006;1:848-58. [PubMed ID: 17406317].
-
40.
Bromley-Brits K, Deng Y, Song W. Morris water maze test for learning and memory deficits in Alzheimer›s disease model mice. J. Vis. Exp. 2011;53:2920.
-
41.
Motaghinejad M, Motevalian M, Fatima S, Hashemi H, Gholami M. Curcumin confers neuroprotection against alcohol-induced hippocampal neurodegeneration via CREB-BDNF pathway in rats. Biomed. Pharmacother. 2017;87:721-40. [PubMed ID: 28095363].
-
42.
Motaghinejad M, Motevalian M, Fatima S, Faraji F, Mozaffari S. The neuroprotective effect of curcumin against nicotine-induced neurotoxicity is mediated by CREB–BDNF signaling pathway. Neurochem. Res. 2017;42:2921-32. [PubMed ID: 28608236].
-
43.
Motaghinejad M, Motevalian M, Fatima S. Mediatory role of NMDA, AMPA/kainate, GABAA and Alpha2 receptors in topiramate neuroprotective effects against methylphenidate induced neurotoxicity in rat. Life Sci. 2017;179:37-53. [PubMed ID: 28082019].
-
44.
Motaghinejad M, Motevalian M, Fatima S, Beiranvand T, Mozaffari S. Topiramate via NMDA, AMPA/kainate, GABAA and Alpha2 receptors and by modulation of CREB/BDNF and Akt/GSK3 signaling pathway exerts neuroprotective effects against methylphenidate-induced neurotoxicity in rats. J. Neural Transm (Vienna). 2017;124:1369-87. [PubMed ID: 28795276].
-
45.
Arican O, Aral M, Sasmaz S, Ciragil P. Serum levels of TNF-α, IFN-γ, IL-6, IL-8, IL-12, IL-17, and IL-18 in patients with active psoriasis and correlation with disease severity. Mediators Inflamm. 2005;2005:273-9. [PubMed ID: 16258194].
-
46.
Demircan N, Safran B, Soylu M, Ozcan A, Sizmaz S. Determination of vitreous interleukin-1 (IL-1) and tumour necrosis factor (TNF) levels in proliferative diabetic retinopathy. Eye (Lond). 2006;20:1366-9. [PubMed ID: 16284605].
-
47.
Shi YQ, Huang TW, Chen LM, Pan XD, Zhang J, Zhu YG, Chen XC. Ginsenoside Rg1 attenuates amyloid-β content, regulates PKA/CREB activity, and improves cognitive performance in SAMP8 mice. J. Alzheimers Dis. 2010;19:977-89. [PubMed ID: 20157253].
-
48.
Lee BH, Kim H, Park SH, Kim YK. Decreased plasma BDNF level in depressive patients. J. Affect. Disord. 2007;101:239-44. [PubMed ID: 17173978].
-
49.
Bruin JE, Gerstein HC, Holloway AC. Long-term consequences of fetal and neonatal nicotine exposure: a critical review. Toxicol. Sci. 2010;116:364-74. [PubMed ID: 20363831].
-
50.
Johnson BA, Roache JD, Ait-Daoud N, Wallace C, Wells LT, Wang Y. Effects of isradipine on methamphetamine-induced changes in attentional and perceptual-motor skills of cognition. Psychopharmacology (Berl). 2005;178:296-302. [PubMed ID: 15452681].
-
51.
Hosseinzadeh H, Sadeghnia HR, Ghaeni FA, Motamedshariaty VS, Mohajeri SA. Effects of saffron (Crocus sativus L) and its active constituent, crocin, on recognition and spatial memory after chronic cerebral hypoperfusion in rats. Phytother. Res. 2012;26:381-6. [PubMed ID: 21774008].
-
52.
Brown JM, Quinton MS, Yamamoto BK. Methamphetamine‐induced inhibition of mitochondrial complex II: roles of glutamate and peroxynitrite. J. Neurochem. 2005;95:429-36. [PubMed ID: 16086684].
-
53.
Naghizadeh B, Boroushaki MT, Vahdati Mashhadian N, Mansouri SMT. Protective effects of crocin against cisplatin-induced acute renal failure and oxidative stress in rats. Iran. Biomed. J. 2008;12:93-100. [PubMed ID: 18506215].
-
54.
Zitka O, Skalickova S, Gumulec J, Masarik M, Adam V, Hubalek J, Trnkova L, Kruseova J, Eckschlager T, Kizek R. Redox status expressed as GSH: GSSG ratio as a marker for oxidative stress in paediatric tumour patients. Oncol. Lett. 2012;4:1247-53. [PubMed ID: 23205122].
-
55.
Griffith OW, Meister A. Origin and turnover of mitochondrial glutathione. Proc. Natl. Acad. Sci. U.S.A. 1985;82:4668. [PubMed ID: 3860816].
-
56.
Harold C, Wallace T, Friedman R, Gudelsky G, Yamamoto B. Methamphetamine selectively alters brain glutathione. Eur. J. Pharmacol. 2000;400:99-102. [PubMed ID: 10913590].
-
57.
Moszczynska A, Turenne S, Kish SJ. Rat striatal levels of the antioxidant glutathione are decreased following binge administration of methamphetamine. Neurosci. Lett. 1998;255:49-52. [PubMed ID: 9839724].
-
58.
Ochiai T, Soeda S, Ohno S, Tanaka H, Shoyama Y, Shimeno H. Crocin prevents the death of PC-12 cells through sphingomyelinase-ceramide signaling by increasing glutathione synthesis. Neurochem. Int. 2004;44:321-30. [PubMed ID: 14643749].
-
59.
Ochiai T, Ohno S, Soeda S, Tanaka H, Shoyama Y, Shimeno H. Crocin prevents the death of rat pheochromyctoma (PC-12) cells by its antioxidant effects stronger than those of α-tocopherol. Neurosci. Lett. 2004;362:61-4. [PubMed ID: 15147781].
-
60.
Imam SZ, Ali SF. Selenium, an antioxidant, attenuates methamphetamine-induced dopaminergic toxicity and peroxynitrite generation. Brain Res. 2000;855:186-91. [PubMed ID: 10650149].
-
61.
Bors W, Michel C, Saran M. Inhibition of the bleaching of the carotenoid crocin a rapid test for quantifying antioxidant activity. Biochim. Biophys. Acta. 1984;796:312-9.
-
62.
Chang L, Alicata D, Ernst T, Volkow N. Structural and metabolic brain changes in the striatum associated with methamphetamine abuse. Addiction. 2007;102:16-32. [PubMed ID: 17493050].
-
63.
Cadet JL, Ordonez SV, Ordonez JV. Methamphetamine induces apoptosis in immortalized neural cells: Protection by the proto‐oncogene, bcl‐2. Synapse. 1997;25:176-84. [PubMed ID: 9021898].
-
64.
Deng X, Cai NS, McCoy MT, Chen W, Trush MA, Cadet JL. Methamphetamine induces apoptosis in an immortalized rat striatal cell line by activating the mitochondrial cell death pathway. Neuropharmacology. 2002;42:837-45. [PubMed ID: 12015210].
-
65.
Oruc S, Gönül Y, Tunay K, Oruc OA, Bozkurt MF, Karavelioğlu E, Bağcıoğlu E, Coşkun KS, Celik S. The antioxidant and antiapoptotic effects of crocin pretreatment on global cerebral ischemia reperfusion injury induced by four vessels occlusion in rats. Life Sci. 2016;154:79-86. [PubMed ID: 27117584].
-
66.
Carlezon WA, Duman RS, Nestler EJ. The many faces of CREB. Trends Neurosci. 2005;28:436-45. [PubMed ID: 15982754].
-
67.
Mayr B, Montminy M. Transcriptional regulation by the phosphorylation-dependent factor CREB. Nat. Rev. Mol. Cell Biol. 2001;2:599-609. [PubMed ID: 11483993].
-
68.
Martinowich K, Hattori D, Wu H, Fouse S, He F, Hu Y, Fan G, Sun YE. DNA methylation-related chromatin remodeling in activity-dependent BDNF gene regulation. Science. 2003;302:890-3. [PubMed ID: 14593184].
-
69.
Yoshii A, Constantine‐Paton M. Postsynaptic BDNF‐TrkB signaling in synapse maturation, plasticity, and disease. Dev. Neurobiol. 2010;70:304-22. [PubMed ID: 20186705].
-
70.
Cao G, Zhu J, Zhong Q, Shi C, Dang Y, Han W, Liu X, Xu M, Chen T. Distinct roles of methamphetamine in modulating spatial memory consolidation, retrieval, reconsolidation and the accompanying changes of ERK and CREB activation in hippocampus and prefrontal cortex. Neuropharmacology. 2013;67:144-54. [PubMed ID: 23159329].
-
71.
Razavi BM, Sadeghi M, Abnous K, Vahdati F, Hosseinzadeh H. Study of the role of CREB, BDNF, and VGF neuropeptide in long term antidepressant activity of crocin in the rat cerebellum. Iran. J. Pharm. Res. 2017;16:1452-62. [PubMed ID: 29552054].