Abstract
Keywords
Nanoparticles Cytotoxicity Cell line Gd2O3-DEG Magnetoliposomes
Introduction
Liposomes as carriers for controlled delivery of drugs that have been drawing much attention which is because of encapsulation of many substances in aqueous and lipid phases (1). Despite the useful properties of nanoparticles, their side effects, mechanism, and their toxicity should be investigated. The important fact about nanoparticles is their remarkable reactivity due to their size, surface chemistry, and oxidative stress. These characteristics may result in toxic effects (2).
The small sizes of nanoparticles increase their surface area and more chemical molecules which attach this surface and increase its reactivity or toxic effects. In addition, these small size nonmaterials pass through cell membranes especially into the mitochondria (2, 3). Despite beneficial characteristics of nano sized particles for drug delivery and contrast agents, they could result unwanted side effects. Both the surface chemistry and chemical components of nanoparticles are the surfaces assumed as important factors, so that the surface modification of nanoparticles can reduce toxicity. The reduction of the toxicity of drugs and contrast agents such as iron oxide or paramagnetic nanoparticles have been shown by coating them with different coatings (3).
Recently, nonmaterial’s such as Gd2O3, super paramagnetic nanoparticles (SPIO) with/without polymeric coating have received enormous attention for their ability to create new types of contrast agents in magnetic resonance imaging (4). Gadolinium is a paramagnetic substance in its trivalent state and is used for MRI because of its strong effect on decreasing the T1 relaxation times of the tissues. However, gadolinium shows the potential toxicity due to its seven unpaired electrons and therefore could be decreased by chelating and coating. The primary magnetic properties of diethylene glycol (DEG) of this group were reported, as a new surface covering material in combination with Gd2O3 nanoparticles (5). However, the synthesis procedure and effective size and agglomeration of gadolinium nanoparticles coated with DEG materials should be studied more (6-8). For this reason, these two groups of surface coating materials (DEG and liposome) even could be useful for covering nanoparticles in biomedical cellular and molecular imaging applications.
For example, cancer cells are more flexible towards nanoparticle toxicity than normal cells due to an increased rate of proliferation and metabolic activity (9, 10). The differences between toxic effects are even observed for nanoparticles of the same materials. The main objective of this study was to evaluate toxicity on biological structures such as biological cells. Therefore, selection of the appropriate cell type that is based on target introduction methods of nanomaterials is an important factor in cytotoxicity assays. For this purpose, Gd2O3-DEG was prepared in new synthetic controlled method and cytotoxicity assays.
This study would be involved in nanoparticles, which are the composition of Gd2O3, DEG, and liposome. Gd2O3 nanoparticles with Diethylene Glycol Polymer could produce a good MR signal and therefore could be a useful potential contrast medium for cell tracking in molecular magnetic resonance imaging (mMRI). In this study, Gd2O3 nanoparticles were used as a model of nonmaterial in order to evaluation of in-vitro potential toxicity in Hepa 1-6 cell lines.
Experimental
Preparation of Gd2O3-DEG
Gd2O3-DEG was prepared through the polyol method, previously reported by this group (11). Next, as a part of the new method, the colloidal liquid was cooled, centrifuged, filtered at 2000 rpm for 0.5 h by using 0.2 µm filter (polyethersulfone, Viva science Sartorius, Hannover, Germany). Free Gd3+ ions and excess DEG in the solution were eliminated by a 1000 MW membrane (Dialysis tubing, benzoylated, Sigma, USA) for 24 h. The agglomerated nanoparticles were removed by 12000 Da membrane (Dialysis tubing cellulose membrane, Sigma, USA) for 24 h across distilled water.
Preparation of Magnetoliposomes
Liposomes were prepared by lipid-film hydration method (6). Distearoylphosphatidylcholine: Cholesterol (DSPC:Chol) (DSPC, Lipoid GmbH, Germany) (Chol, Sigma, Germany) with a molar ratio 50:50. A mixture of the appropriate amounts of lipids (typically: 50 mmols of total lipid) was dissolved in chloroform/methanol 2:1 (v/v) and evaporated for 2 h in order to dry by rotary evaporation at 65 °C. Then, appropriate amounts of Gd2O3-DEG in distilled water were added to lipid film and hydrated for 2 h. After preparation of the Magnetoliposomes (ML), sonication for 0.5 h and dialysis for 24 h were performed in order to remove unentrapped Gd2O3-DEG (7). In-vitro dilutions with five different concentration forms 0.3, 0.6, 0.9, 1.2, 1.5 mM of Gd-DTPA, Gd2O3-DEG, and MLNs were prepared.
The Characterization Study
The concentration of the Gd2O3-DEG and ML nanoparticles was determined by inducing coupled plasma-atomic (ICP) (AES-Varian-Liberty 150 AX Turbo-USA), and after paramageto liposomes nanoparticles (PMLNs) sonication, dialysis of the membrane was performed. The measurements of particle morphology and hydrodynamic diameter were performed by transmission of electron microscopy (TEM) (Philips, Model CM 120, Netherland) and dynamic light scattering (DLS), (Malvern Instrument-UK).
Cell Culture
Even though these results may not accurately predict the in-vivo toxicity, it provides a basis for understanding the mechanism of toxicity and nanoparticle uptake at the cellular level (12). The Hepa 1-6 was derived from BW7756 tumor in a C57L mouse (National Cell Bank of Iran, Pasteur Institute of Iran). The cells were cultured in DMEM (Invitrogen, Auckland, New Zealand) with 2 mM glutamine and 2 g/L sodium bicarbonate (Sigma, St Louis, MO) adjusted to contain 4.5 g/L glucose and 10% fetal bovine serum (FBS) (Invitrogen) and 1% penicillin/streptomycin (Invitrogen, Carlsbad, CA) at 37 °C in a 5% CO2 atmosphere.
Microculture tetrazolium test (MTT assay)
The inhibitory effect of Gd-DTPA, Gd2O3–DEG nanoparticles, and PMLNs on the growth and proliferation of Hepa 1-6 cell lines were assessed by up-taking of thiazolyl blue tetrazolium bromide (MTT, Sigma) by viable cells (13). The cells were plated onto 96-well plates (Orange Scientific, Brussels, Belgium) at a density of 1.0 × 104 cell/100 µL/well. After incubation at 37 °C for 24 h, the medium was replaced with either control medium or medium containing specialized concentration Gd2O3 for 24, 48 and 72 h. The concentration of Gd2O3 for cell culture treatment was 0.3, 0.6, 0.9, 1.2 mM, respectively and the concentration of zero means negative control. One-hundred microliter of MTT solution (0.5 mg/mL) was added to each well and then the cells incubated at 37 °C for 3 h. Following solubilize the precipitated formazan with 100 µL DMSO, the optical densitometry was measured at a wave length of 570 nm. The inhibition rate (IR) was evaluated using the following equation: IR (%) = 1 - ODexp/ODcon × 100, where ODexp and ODcon are the optical densitometries of treated and untreated cells, respectively. The viability rate of Gd2O3 was evaluated using the following equation: Viability (%) = 100 - IR (%) (14).
LDH leakage
LDH assay was performed for detecting the cytotoxicity index of Gd2O3-DEG nanoparticles Gd-DTPA and Magnetoliposomse nanoparticles in the cell medium by using a commercially available kit (Roche Applied Science).
Serum Lactate Dehydrogenase (LDH) Assay—Serum stored at 20 °C was used for this assay, which was performed according to the manufacturer’s protocol (Roche Applied Science) (15). Briefly, after the exposure of Hepa 1-6 cell lines to Gd2O3 -DEG nanoparticles, Gd-DTPA and PMLNS for 2 h, 100 µL of supernatant (cell medium+ Serum+ Nanoparticles) was transferred into an optically clear 96-well flat-bottomed microtiter plate. The concentration of Gd2O3 for cell culture treatment was 0.3, 0.6, 0.9, 1.2, 1.5 mM, respectively and the concentration of zero means negative control. To determine LDH activity, 100 µL of the reaction mixture was added to each well and incubated for 30 min at 15–25 °C. The control group is 0.1% DMSO (Dimethyl sulfoxide). The spectrophotometer was calibrated to zero absorbance using culture medium without cells. The relative LDH leakage (%) related to control wells containing cell culture medium without nanoparticles or PBS as a vehicle was calculated by (A)test/(A)control × 100.
The mark (A)test is the absorbance of the test sample and (A)control is the absorbance of the control sample. The absorbance of the samples was measured at 490 nm using an ELISA reader. Released LDH in culture supernatants causes the conversion of a tetrazolium salt (INT) into a red formazan product. The amount of color formed is proportional to the number of lysed cells. Cytotoxicity is expressed relative to the released basal LDH by untreated control cells (16).
Statistical Analysis
Statistical analysis was done by using repeated measures ANOVA for comparing paired samples (within-subjects) and One-way ANOVA for between subjects (compared means between groups). P-values less than 0.05 were considered statistically significant. The percentages of cell viability were presented graphically in the form of histograms, using Microsoft Excel computer program. Data are expressed as mean ± standard deviation (SD). All experiments were examined in triplicate.
Results
Characterization of the contrast materials
Table 1 shows the size and polydispersity index (PdI) measurements using the DLS, thereby, Gd2O3-DEG and PML nanoparticles had a hydrodynamic diameter distribution of 27.29 ± 6.10 nm with a PdI of 0.357, and the size of 128.86 ± 36.24 nm with the PdI of 0.387, respectively.
The results showed that with increasing the molecular weight, the nanoparticles size increased as well. However, despite their different sizes, PdI’s nanoparticles (as an index of the nanoparticles dispersion) had acceptable ranges of less than 0.5.
Figure 1 shows the morphology of three wrapped around nanoparticles, while specifically, just images of Gd2O3-DEG are sharp and uniformly such that spherical or ellipsoidal shape of Gd nanomagnetic particles could be visualized separately with clear grains in nano dimensions. Whiles the images of magneto liposomes could not be viewed as sharp as Gd2O3-DEG nanoparticles among their surface covers because of large molecular weights were agglomerated such that.
Cell Morphology
The general morphology of the cell incubated with nanoparticles in phase-contrast microscopy is shown in Figure 2. At the end of 24 h, the exposed cells were washed with PBS and the cells were visualized by phase-contrast microscopy.
Figure 2 shows Hepa 1-6 cell lines that were well spread after incubation with Gd2O3-DEG for 24 h, and there was no distinct change in morphology after incubation for 24 h with any concentration of Gd2O3-DEG nanoparticles relatives. Similar results were obtained with Magneto liposomes.
Microculture tetrazolium test (MTT assay)
The Metabolic function of the cells was measured by means of the MTT assay after culturing in the presence of the nanoparticles for 24, 48, and 72 h. As is evident from Figure 2, the Gd2O3 nanoparticles with DEG, Magnetoliposomes had no significant effect. Hepa 1-6 cell lines were exposed to different doses of nanoparticles for 24, 48, and 72 h as indicated in the Materials and Methods. Each point represents a mean value and standard deviation of 3 experiments. Cell Viability in different concentrations is not significantly different (P < 0.05) compared to the control, as is evident from Figure 3. The MTT assay showed that Gd2O3-DEG nanoparticles are more toxic than other nanoparticles.
LDH leakage
The cellular toxicity of gadolinium oxide nanoparticles in-vitro was determined by measuring the releasing of the enzyme lactate dehydrogenize (LDH) into the cell culture supernatants with 24, 48, and 72 h incubation period and the gadolinium concentration of all particles. In order to control completeness of LDH releasing, one sample was lysed and its LDH value was taken as 100% toxicity. The effect was comparable to the exposure to 0.1% (v/v) DMSO.
Concentration-dependent effects of mM on cytotoxicity of Hepa 1-6 cell line. The cells were treated with designated concentrations of mM and 0.1% (v/v) of DMSO as a vehicle control.
After 72 h, LDH leakage was assayed in supernatants and cytotoxicity was expressed as a LDH% release. Statistically, different values of P = 0.005 (0.6 mM), P = 0.013 (0.9 mM), P = 0.023 (1.2 mM), and P = 0.003 (1.5 mM) were determined for 72 h after plating, compared to the control.
Since the LDH assay detects that lysis and cells arenot viable, the cells need to be destroyed for these investigations to optimize test conditions. Therefore, the positive control Triton X-100 was used, at the concentration of 2% to complete lysis of cells.
The results of the LDH assay showed Gd-DTPA and two nanoparticles did not produce cytotoxicity in different concentrations. There were no significant differences between two nanoparticles and Gd-DTPA is used as commercially contrast agents in MRI department (Figure 4).
There was a slight increase in LDH leakage at different doses of Gd2O3-DEG compared to other nanoparticles but Gd-DTPA was not statistically significant (P < 0.05). However, the results were similar for MTT and LDH assay (Figures 3 and 4).
Discussion
Many harmful effects of nanoparticles could be imagined at the cellular level by interacting with vital cell components such as membrane and mitochondria. The physicochemical properties of nanoparticles can affect their pharmacokinetics such as distribution, metabolism, and absorption.
Contrast agents can modify the signal intensity in different tissues by enhancing their contrast and also improving the low sensitivity of magnetic resonance imaging. Recently, studies have shown high efficiency and sensitivity of contrast agents when they have been used in the form of nanoparticles. For having higher relaxivity, reducing toxicity, increasing biocompatibility and half-life, besides preventing the nanoparticles aggregations, contrast agents should be coated with various materials in MRI.
The development of suitable molecular magnetic resonance imaging (mMRI) by using contrast agents, is very important (17). Many methodical reports have been manifested in the last decade, which highlighted this issue, with the goal of understanding the interactions between different types of nanoparticles and cells as functions of size, shape, and surface chemistry of the nanomaterials (18). In-vitro studies using different cell systems demonstrated varying grades of prion inflammatory and oxidative-stress–related cellular answers after doing with laboratory-generated or filter collected environment ultrafine particles (19, 20). Gadolinium with seven unpaired electrons and gadolinium oxide are used as contrast agents for magnetic resonance imaging (MRI). Despite good relaxivity, Gd has high toxicity and it might be chelated or conjugated with other materials such as polymers or liposomes. Some primary magnetic properties of diethylene glycol (DEG) in combination with Gd oxide has been reported previously as a new surface covering material. On the other hand, because of its considerable physicochemical properties, liposome has drawn notable interest incovering the nanoparticles surfaces (21-25). For these reasons, these two groups of surface conjugated materials (DEG and Liposomes) could be useful for covering nanoparticles in biomedical cellular and molecular imaging applications. For this purpose, Gd2O3-DEG was prepared in new synthetic controlled method. However, little is known about how these coatings influence the toxicity of these particles. Therefore, we compared toxicity of contrast agent nanoparticles with Gd2O3 core and two different coatings with conventional contrast agent Gd-DTPA. As these contrast agents might be used for detection of Liver cancer, Hepa 1-6 cell lines were selected as a convenient in-vitro model to assess toxicity. Selection of a suitable cytotoxicity assay method is also an important consideration, since some may interfere with the actual toxic effect produced by the nanoparticle (26-29). For toxicity evaluations, cellular morphology, mitochondrial function (MTT assay), membrane leakage of lactate dehydrogenase (LDH assay) were assessed under control and exposed conditions (24 h, 48 h, 72 h of exposure).
Figure 2 shows the morphology of Gd2O3-DEG nanoparticle-exposed cells. Despite the relatively higher toxicity (LDH and MTT assay) in comparison to other nanoparticles and Gd-DTPA, no relevant differences were observed in for the different concentration of Gd2O3-DEG.
The results demonstrated that exposure to Gd based nanoparticles for 24 h resulted in a concentration-dependent increase in LDH leakage and exhibited is significant (P < 0.05) in cytotoxicity of different concentrations. Cell viability in different concentrations is not significantly different (P < 0.05) in comparison to the control. The MTT assay showed that Gd2O3-DEG nanoparticles are more toxic than other nanoparticles.
The MTT and LDH assay showed that Gd2O3-DEG nanoparticles are more toxic than Gd-DTPA and other nanoparticles. Also, Magnetoliposomes is more biocompatible than other nanoparticles. Magneto liposomes and Gd2O3-DEG nanoparticles, the two new synthesized and induced contrast agents are completely similar, except for their molecular weight. It appears that higher molecular weight can lead to more coverage and more biocompatibility.
Interestingly, they found no cellular damage evidence for 15-nm gold nanospheres bearing the same surface group. This result highlights the possible size-dependent toxicity of gold nanoparticles (30). In particular, gold nanoparticles less than 2 nm in diameter show chemical reactivity evidence that does not occur at larger sizes (31).
The conflicting results could arise from the variability of the used toxicity assays, cell lines, and nanoparticles chemical/physical properties. For example, cytotoxicity results can vary by using cell line. Citrate-capped gold nanoparticles (13 nm in diameter) were found to be toxic to a human carcinoma lung cell line, but not to human liver carcinoma cell line at the same dosage (32). Furthermore, varying the dose range parameters and the exposure time of gold nanoparticles to the cells in these studies, make it difficult to compare.
DLS size and PdI measurement for two nanoparticle contrast agents. The results show a direct relationship between size and molecular weight
Nanoparticles Hydrodynamic Diameter (nm) | PdI | |
---|---|---|
Gd2O3-DEG 27.29 ± 6.10 | 0.357 | |
PMLNs 128.86 ± 36.24 | 0.387 |
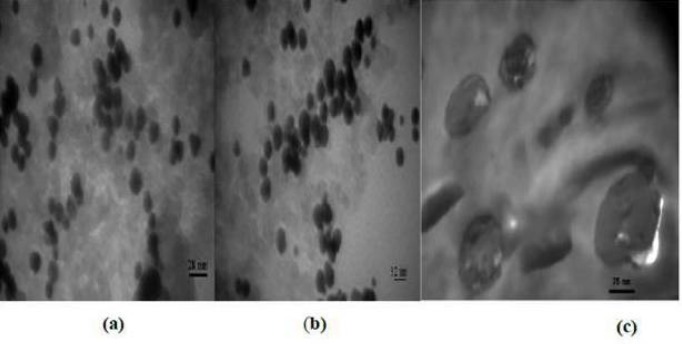
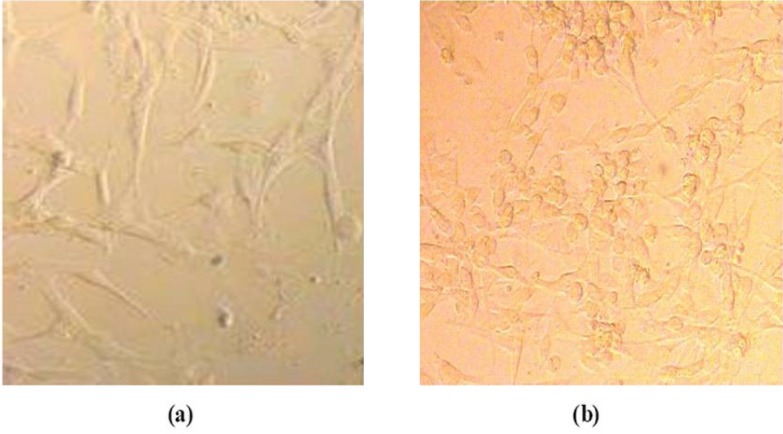
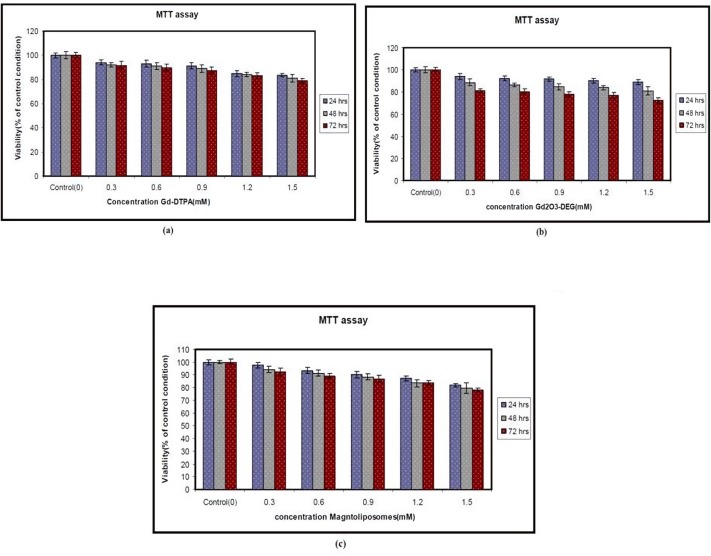
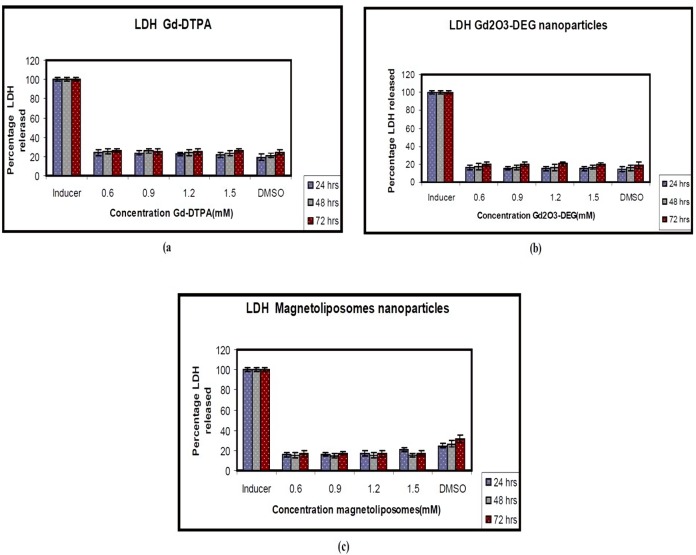
We introduced two new nanoparticles with similar core and different coatings and assayed their toxicity in comparison with Gd-DTPA. In this study, Gd2O3 based contrast agents coated with two non-toxic different coatings. Therefore, among different groups’ coating materials, DEG, and liposomes due to their considerable properties containing different sizes and molecular weights, noticeable relaxivity, magnetic property, and high signal intensity, those are proper for cellular and molecular MRI applications that would be remained for future in-vivo studies.
References
-
1.
Takano S, Aramaki Y, Tsuchiya S. Physicochemical properties of liposomes affecting apoptosis induced by cationic liposomes in macrophages. Pharm. Res. 2003;20:962-8. [PubMed ID: 12880280].
-
2.
Foley S, Crowley C, Smaihi M, Bonfils C, Erlanger BF, Seta P, Larroque C. Cellular localisation of a water-soluble fullerene derivative. Biochem. Biophys. Res. Commun. 2002;294:116-9. [PubMed ID: 12054749].
-
3.
Oberdörster G, Sharp Z, Atudorei V, Elder A, Gelein R, Kreyling W, Cox C. Translocation of inhaled ultrafine particles to the brain. Inhal. Toxicol. 2004;16:437-45. [PubMed ID: 15204759].
-
4.
Engström M, Klasson A, Pedersen H, Vahlberg C, Käll PO, Uvdal K. High proton relaxivity for gadolinium oxide nanoparticles. Magn. Reson. Mater. Phy. 2006;19:180-6.
-
5.
Riyahi-Alam N, Behrouzkia Z, Seifalian A, Jahromi SH. Properties evaluation of a new MRI contrast agent based on Gd-loaded nanoparticles. Biol. Trace Elem. Res. 2010;137:324-34. [PubMed ID: 20049554].
-
6.
Oussoren C, Eling W, Crommelin D, Storm G, Zuidema J. The influence of the route of administration and liposome composition on the potential of liposomes to protect tissue against local toxicity of two antitumor drugs. Biochim. Biophys. Acta. 1998;1369:159-72. [PubMed ID: 9528684].
-
7.
Roberts D, Zhu WL, Frommen CM, Rosenzweig Z. Synthesis of gadolinium oxide magnetoliposomes for magnetic resonance imaging. J. Appl. Phys. 2000;87:6208-10.
-
8.
Kuriashkin IV, Losonsky JM. Contrast enhancement in magnetic resonance imaging using intravenous paramagnetic contrast media: a review. Vet. Radiol. Ultrasound. 2000;41:4-7. [PubMed ID: 10695873].
-
9.
Nan A, Bai X, Son SJ, Lee SB, Ghandehari H. Cellular uptake and cytotoxicity of silica nanotubes. Nano Lett. 2008;8:2150-4. [PubMed ID: 18624386].
-
10.
Chang JS, Chang KLB, Hwang DF, Kong ZL. In-vitro cytotoxicitiy of silica nanoparticles at high concentrations strongly depends on the metabolic activity type of the cell line. Environ. Sci. Technol. 2007;41:2064-8. [PubMed ID: 17410806].
-
11.
Riyahi-Alam N, Behrouzkia J, Haghgoo S, Seifalian A, Aghdam R, Azizian G. Gd2O3 nanoparticles as a positive MRI contrast agent for cell uptake. 17thIranian Conference of Biomedical Engineering (ICBME): IEEE. 2010.
-
12.
Griffith LG, Swartz MA. Capturing complex 3D tissue physiology in-vitro. Nat. Rev. Mol. Cell Biol. 2006;7:211-24. [PubMed ID: 16496023].
-
13.
Mosmann T. Rapid colorimetric assay for cellular growth and survival: application to proliferation and cytotoxicity assays. J. Immunol. Methods. 1983;65:55-63. [PubMed ID: 6606682].
-
14.
Derfus AM, Chan WC, Bhatia SN. Probing the cytotoxicity of semiconductor quantum dots. Nano Lett. 2004;4:11-8. [PubMed ID: 28890669].
-
15.
Braydich-Stolle L, Hussain S, Schlager JJ, Hofmann MC. In-vitro cytotoxicity of nanoparticles in mammalian germline stem cells. Toxicol. Sci. 2005;88:412-9. [PubMed ID: 16014736].
-
16.
Hengerer A, Grimm J. Molecular magnetic resonance imaging. Biomed. Imaging Interv. J. 2006;2:1-7.
-
17.
Lewinski N, Colvin V, Drezek R. Cytotoxicity of nanoparticles. Small. 2008;4:26-49. [PubMed ID: 18165959].
-
18.
Wörle-Knirsch J, Pulskamp K, Krug H. Oops they did it again! Carbon nanotubes hoax scientists in viability assays. Nano Lett. 2006;6:1261-8. [PubMed ID: 16771591].
-
19.
Brown DM, Wilson MR, MacNee W, Stone V, Donaldson K. Size-dependent proinflammatory effects of ultrafine polystyrene particles: a role for surface area and oxidative stress in the enhanced activity of ultrafines. Toxicol. Appl. Pharmacol. 2001;175:191-9. [PubMed ID: 11559017].
-
20.
Li N, Sioutas C, Cho A, Schmitz D, Misra C, Sempf J, Wang M, Oberley T, Froines J, Nel A. Ultrafine particulate pollutants induce oxidative stress and mitochondrial damage. Environ. Health Perspect. 2003;111:455.
-
21.
Kuo PH. Gadolinium-containing MRI contrast agents: important variations on a theme for NSF. J. Am. Coll. Radiol. 2008;5:29-35. [PubMed ID: 18180006].
-
22.
Casey A, Herzog E, Davoren M, Lyng F, Byrne H, Chambers G. Spectroscopic analysis confirms the interactions between single walled carbon nanotubes and various dyes commonly used to assess cytotoxicity. Carbon. 2007;45:1425-32.
-
23.
Ciofani G, Danti S, D’Alessandro D, Moscato S, Menciassi A. Assessing cytotoxicity of boron nitride nanotubes: interference with the MTT assay. Biochem. Biophys. Res. Commun. 2010;394:405-11. [PubMed ID: 20226164].
-
24.
Park MV, Annema W, Salvati A, Lesniak A, Elsaesser A, Barnes C, McKerr G, Howard CV, Lynch I, Dawson KA. In-vitro developmental toxicity test detects inhibition of stem cell differentiation by silica nanoparticles. Toxicol. Appl. Pharmacol. 2009;240:108-16. [PubMed ID: 19631676].
-
25.
Pedersen H, Söderlind F, Petoral Jr RM, Uvdal K, Käll PO, Ojamäe L. Surface interactions between Y2O3 nanocrystals and organic molecules an experimental and quantum-chemical study. Surf. Sci. 2005;592:124-40.
-
26.
Kozlowska D, Foran P, MacMahon P, Shelly MJ, Eustace S, O′Kennedy R. Molecular and magnetic resonance imaging: The value of immunoliposomes. Adv.Drug Deliv. Rev. 2009;61:1402-11. [PubMed ID: 19796661].
-
27.
Lauffer RB. Paramagnetic metal complexes as water proton relaxation agents for NMR imaging: theory and design. Chem. Rev. 1987;87:901-27.
-
28.
Fortin MA, Petoral Jr RM, Söderlind F, Klasson A, Engström M, Veres T, Käll PO, Uvdal K. Polyethylene glycol-covered ultra-small Gd2O3 nanoparticles for positive contrast at 15 T magnetic resonance clinical scanning. Nanotechnology. 2007;18:1-9.
-
29.
Pan Y, Leifert A, Ruau D, Neuss S, Bornemann J, Schmid G, Brandau W, Simon U, Jahnen‐Dechent W. Gold nanoparticles of diameter 14 nm trigger necrosis by oxidative stress and mitochondrial damage. Small. 2009;5:2067-76. [PubMed ID: 19642089].
-
30.
Turner M, Golovko VB, Vaughan OP, Abdulkin P, Berenguer-Murcia A, Tikhov MS, Johnson BF, Lambert RM. Selective oxidation with dioxygen by gold nanoparticle catalysts derived from 55-atom clusters. Nature. 2008;454:981-3. [PubMed ID: 18719586].
-
31.
Patra HK, Banerjee S, Chaudhuri U, Lahiri P, Dasgupta AK. Cell selective response to gold nanoparticles. Nanomed-Nanotechnol. 2007;3:111-9.
-
32.
Nel A, Xia T, Mädler L, Li N. Toxic potential of materials at the nanolevel. Science. 2006;311:622-7. [PubMed ID: 16456071].