Abstract
Keywords
Melissa officinalis Aqueous Extract Ethanolic Extract Antioxidant Anti-inflammatory Paw-edema
1. Background
The search for novel medicinal plant-based pharmacotherapy for various diseases mediated by inflammation and oxidative stress has progressed significantly due to their fewer side effects and better tolerability. Thousands of plants are currently being investigated as potential reservoirs for discovering new drugs. Melissa officinalis L. (lemon balm, MO) is a perennial aromatic herb and a member of the mint family (Lamiaceae), which has gained scientific attention due to its long known usage in traditional medicine and multiple pharmacological effects proven in preclinical and clinical studies (1). Its traditional usage as a sedative, hypnotic, memory enhancer, and antidepressant has reached a whole new level and expanded to a broad spectrum of pathologies, thanks to several investigations confirming its effects in cardio-metabolic pathologies, different cancer types, viral infections, and neurodegenerative diseases (2, 3). Several mechanisms mediate these effects. Nonetheless, the antioxidant potential of its bioactive compounds stands out as the major one. Melissa officinalis L. has been the focus of research in the last decade. Different formulations of MO have been investigated so far, such as essential oil, tea, and several extract types. The leaves are most commonly used as an herbal drug since they are the richest source of bioactive compounds, including volatile compounds such as different monoterpenes, sesquiterpenes, phenolic acids, and flavonoids. The phenolic acids found in MO include rosmarinic acid (RA), caffeic acid, protocatechuic acid, cinnamic acid, chlorogenic acid, gallic acid, ferulic acid, ellagic acid, p- coumaric acid, and salvianolic acid. Rosmarinic acid is considered the main bioactive constituent (3-5). Additionally, different flavonoids were identified such as luteolin, apigenin, hesperidin, hesperetin, naringin, naringenin, catechin, epicatechin, routin, quercetin, myricetin, quercitrin, rhamnocitrin, and isoquercitrin (3, 5). It is noteworthy that the presence of these compounds and their abundance vary depending on the extraction process and conditions, used solvent, region’s climate, plant species, and maturity stage of MO (6). Several studies reported the superior antioxidant capacity of MO essential oil (7) and extracts. Moreover, there is evidence about oxidative stress ameliorating properties of MO extracts (MOEs), mostly aqueous or hydroalcoholic extracts. Besides, their phenolic compounds exert antioxidant activity through free-radical scavenging, lipid peroxidation inhibition, and endogenous antioxidant system protection (8, 9). The anti-inflammatory potential of MOEs is expected since it represents a great mixture of bioactive compounds known to suppress inflammation in different conditions (10). In vivo anti-inflammatory potential of MO essential oil was previously confirmed via significant reduction and inhibition of carrageenan-induced paw edema in rats, with citrals being the components mostly mediating this effect via TNF-α inhibition (11).
2. Objectives
However, up to date, no study has explored and compared the phenolic and flavonoid content, in vitro antioxidant capacity, in vivo anti-inflammatory potential, and redox status of three different MOEs, including aqueous extract ethanolic macerate, and ethanolic extract under reflux. Thus, this study aimed to evaluate and compare the active components content, antioxidant, and anti-inflammatory potential of three different MOEs and their effects on systemic redox status after acute per os administration in vivo.
3. Experimental
3.1. Compliance with Ethical Standards
All experimental procedures used in this study that involved laboratory animals were approved by the Ethics Committee of the Faculty of Medical Sciences, University of Kragujevac (Kragujevac, Serbia) No. 01-10171. Additionally, all the experimental procedures were carried out according to appropriate European regulations.
3.2. Plant Material
Dried leaves of M. officinalis L. (Lamiaceae) were used in the study. The plant material used for the analysis was bought from Bilje Borča, LLC (Belgrade, Serbia). Obtained dried plant material was powdered by a mill (IKA A11, Germany). The powdered plant was then put and kept in well-sealed paper bags at room temperature until extraction.
3.3. Extracts Preparation
Three different extracts were made for this research. Extract 1 (E1) was created using the maceration extraction process that involved extracting the dried plant material for three days with 70% ethanol in a well-sealed container with mixing on a shaker for three days at room temperature. The other two extracts were made under reflux of the solvent. This kind of extraction was done at the boiling temperature of the used solvent. Aqueous (E2) and ethanolic (E3) extracts were prepared by extracting the above ground part of the plant with a solvent (water or 70% ethanol). The extraction process lasted for 2.5 hours, followed by the filtering of the mixture through a gaze. After that, it was left at room temperature so it could spontaneously precipitate ballast substances. The obtained liquid extracts were then filtered through filter papers (Whatman, No.1). Finally, we used a rotary vacuum evaporator (RV05 basic IKA, Germany) at 40°C, 90 rpm, and 250 Mbar vacuum to get all three dry extracts. Dried extracts were then stored in dark glass vials at 4ºC until use for further testing (12).
3.4. Phytochemical Analysis of Melissa officinalis Extracts
3.4.1. Determination of Phenolic and Flavonoid Content
The total phenolic content of the examined extracts was determined based on the spectrophotometric method, which involved the oxidation of polyphenolic compounds in the presence of Folin-Ciocalteu reagent (FC, a mixture of phosphotungstic and phosphomolybdic acid) to phenoxide anions, and the reduction of reagents to tungsten oxide and blue molybdenum oxide. In summary, 100 µL of 0.1 % (m/m) aqueous solution of dry extracts was mixed with 0.5 mL of 0.2 M FC reagent and 0.6 mL solution of Na2CO3 (c = 60 g/L). The intensity of the blue color was determined by a spectrophotometer at λ = 760 nm after 30 min incubation time. The total phenolics were expressed as gallic acid equivalents (GAE) per gram of dry extract (d.e.) based on the standard curve obtained for gallic acid under the same experimental conditions.
The total flavonoid content determination was based on the properties of flavonoids to build complexes with metals. In the reaction of flavonoids with AlCl3, Al3+ binds to total flavonoids and forms a complex of flavonoids with aluminum. Specifically, 500 µL of 10% (m/m) aqueous extract was diluted with extraction solution (10% solution (v/v) of acetic acid in methanol) to 10 mL. Furthermore, the test tube contained 5 mL of the obtained solution and 10 mL of AlCl3 reagent (133 mg AlCl3 × 6H2O and 400 mg of CH3COONa dissolved in 100 mL of distilled water), while the blank tube contained distilled water instead of AlCl3 reagent. The intensity of the colored complex in the test tube was determined spectrophotometrically аt λ = 430 nm against blank. The quantified total flavonoids were expressed as quercetin equivalents (QE) per gram of d.e. based on the calibration curve obtained for quercetin under the same experimental conditions (13).
3.4.2. Chemical Profiling
The tested extracts were chemically characterized, and selected compounds were quantified by a validated high-performance liquid chromatography (HPLC) method. In particular, phenolic compounds were separated using an Agilent Technologies 1100 liquid chromatographer equipped with a diode array detector (Agilent Technologies, Santa Clara, California, USA). For separating the natural plant components, we used a reversed-phase Nucleosil C18 column (250 mm × 4.6 mm, 5 μm particle size; Agilent Technologies) held at 30°C. The first solvent was 1% (v/v) aqueous formic acid, while the second was methanol. The following program was used to deliver the mobile phase in the gradient mode: 0 min 10% methanol, 10 min 25% methanol, 20 min 45% methanol, 35 min 70% methanol, 40 min 100% methanol, and 46 min 10% methanol. The fresh HPLC mobile phase was prepared daily and filtered (nylon filter with pore size 0.45 μm). Then, 10 μL of extract solution was injected into the HPLC column, and the flow rate was changed for 48 min, as follows: 1 mL/min for the first 10 min; 0.8 mL/min for the second 10 min, 0.7 mL/min for the third 10 min, and 1 mL/min for the last 18 min (14). Standard solutions of the following components were used for quantification: Gallic acid (GA), caffeic acid (CA), trans-cinnamic acid (CNA), p-coumaric acid (pQA), chlorogenic acid (CHA), rosmarinic acid (RA), ferulic acid (FA), quercetin (Qe), rutin (R), and quercitrin (Qt). All standards were run under the same experimental conditions, and methanol was used as a solvent. We analyzed GA, CA, and CNA at 280 nm, pQA, CHA, RA, FA, and Qe at 330 nm, and R and Qt at 350 nm. Agilent OpenLAB Chemstation v.A.01.05 (Agilent Technologies) software was used for data processing. The results were expressed as mg/g of dry extract.
3.5. Antioxidant Potential Determination
The potential of the examined lemon balm extracts to neutralize 2,2-diphenyl-1-picrylhydrazyl (DPPH), hydroxyl (OH), and nitroso (NO) radicals were assessed by well-known spectrophotometric methods (13). Also, the ability of the studied extracts to protect the integrity of biological cell membranes containing lipids was estimated via the determination of lipid peroxidation (LP) inhibition potential, where liposomes emulsion was used as a test model (15). In addition, ferric reduction antioxidant potential (FRAP) test was performed to examine the ability of the extracts to reduce ferric ions, according to the previous research (13). Ascorbic acid (AA), propyl gallate (PG), and tert-butylated hydroxytoluene (BHT) served as positive controls for antioxidant tests. Each sample was recorded four times in all test systems. The obtained results were expressed as the IC50 value, representing the concentrations of the extracts and positive controls that caused 50% of neutralization/inhibition, determined by linear regression analysis. The results were presented as AA equivalents (AAE) per gram of dehydrated extract in the case of the FRAP assay.
3.6. In Vivo Evaluation of Anti-inflammatory Effects
3.6.1. Animals
Eighty-eight male dark agouti rats (eight weeks of age; body weight 160 ± 20 g) were included in the research. First, the animals were acclimatized for two weeks. Rats were kept in the vivarium of the Faculty of Medical Sciences, University of Kragujevac, Serbia. We assured that the animals were housed in standard conditions for a vivarium, including a temperature of 22 ± 2°C, a light/dark cycle of 12/12 h, and free access to standard food (9% fat, 20% protein, 53% starch) and water (ad libitum).
All animals (n = 88) were randomly divided into following equal groups (n = 8): CTRL-control group animals treated with saline; IND animals treated with standard NSAID indomethacin (8 mg/kg); E150, E1100, and E1200 animals treated with extract E1 at 50, 100, or 200 mg/kg; E250, E2100, and E2200 animals treated with extract E2 at 50, 100, or 200 mg/kg; and E350, E3100, and E3200 animals treated with extract E3 at 50, 100, or 200 mg/kg.
3.6.2. Carrageenan-Induced Paw Edema Model
The anti-inflammatory potential of the investigated extracts E1, E2, and E3 at the mentioned three doses was assessed via the carrageenan-induced paw edema model (16). Sixty minutes after per os administration of the extract/indomethacin/saline, 0.1 mL of 0.5% carrageenan (prepared as a 1% w/v solution in 0.9% saline) was injected into the right hind paw of rats, while the left hind paw was not treated and it was taken as a Witness or control.
The thickness of each rat’s paw tissue was always measured in the same way, in the middle of the rat paw with a digital caliper. The measurements were performed immediately before inflammation and one, two, three, four, and six hours post-carrageenan injection. The thickness of the rat paws was measured in the middle of the rat's paw with a digital caliper. Differences in tissue thickness between the right and left paws of treated versus untreated rats served as a measure of the anti-inflammatory effect achieved. The inhibition (reduction) of paw edema was calculated as a percentage according to the following formula:
where Δk is the difference in paw tissue thickness in the control (untreated) group, and Δe is the difference in paw tissue thickness in the experimental group. The maximum anti-inflammatory effect could be 100%. All measurements were performed three times by the same person to avoid bias. After the carrageenan test, all animals were sedated with a mixture of ketamine and xylazine at frequently used doses of 10 and 100 mg/kg, respectively, and sacrificed by decapitation to collect blood samples and determine the redox status parameters.
3.6.3. Redox Status Post-carrageenan Application
Collected blood samples were used to determine the parameters of oxidative stress spectrophotometrically according to our previous research. The pro-oxidant parameters determined in plasma samples were hydrogen peroxide (H2O2), nitrites (NO2-), and the index of lipid peroxidation measured as thiobarbituric acid reactive substances (TBARS). The parameters of antioxidant protection were determined from erythrocytes lysate samples, including the activity of catalase (CAT) and superoxide dismutase (SOD) and the level of reduced glutathione (GSH) (17).
The H2O2 determination was based on the oxidation of phenol red by H2O2 in a reaction catalyzed by horseradish peroxidase. The measurement of H2O2 in plasma samples was performed at λ= 610 nm. The NO level was measured indirectly by measuring nitrites (NO-2). Nitrites in plasma samples were measured using Griess’s reagent at λ = 550 nm. The degree of lipid peroxidation in plasma samples was assessed indirectly by measuring the level of TBARS using 1% thiobarbituric acid in 0.05 sodium hydroxide at an absorbance of 530 nm (17).
The CAT buffer, prepared RBC lysate sample, and 10 mM H2O2 were used for CAT activity determination at an absorbance of 360 nm. The SOD activity was determined based on the epinephrine method, which involved mixing a sample of RBC lysates with carbonate buffer and epinephrine and then measuring the absorbance at λ= 470 nm. The GSH level was determined according to GSH oxidation by 5,5-dithiobis-6,2-nitrobenzoic acid. The GSH level was measured at λ = 420 nm (17).
3.7. Material
All chemicals used in the study for preparing reagents, solutions, and standards (indomethacin, carrageenan 5,5-dithiobis-6,2-nitrobenzoic acid (DTNB), L-Glutathione reduced (GSH), epinephrine, sodium hydroxide, thiobarbituric acid (TBA), phenol red (Phenolsulfonphthalein), peroxidase from horseradish, ascorbic acid (AA), propyl gallate (PG), tert-butylated hydroxytoluene (BHT), phosphotungstic acid hydrate, phosphomolybdic acid hydrate, gallic (GA), rosmarinic (RA), trans-cinnamic (CNA), p-coumaric (pQA), chlorogenic (CHA), caffeic (CA), ferulic acid (FA), quercetin (Qe), quercitrin (Qt), and rutin (R)) were obtained from Sigma Aldrich (St. Louis, Missouri, United States).
3.8. Statistical Analyses
Statistical analysis of the obtained data was performed by IBM SPSS 20.0 for Windows. We used Kolmogorov-Smirnov and Shapiro-Wilk tests to check the normality of data distribution. Data were expressed as means ± standard deviation (SD), and the differences between groups were analyzed by one-way analysis of variance (ANOVA), followed by the Bonferroni test. The difference was considered statistically significant when the P-value was lower than 0.05.
4. Results
4.1. Phytochemical Analysis of Melissa officinalis Extracts
Depending on the extraction type and the polarity of the solvent, different extraction yields were obtained and expressed as a percentage in 100 g of the drug. The E2 yield was 5.37 g (26.8%), which was considerably higher than E1 (0.3 g; 1.5%) and E3 (1.66 g; 8.3%) yields. Ethanolic extracts E1 and E3 were slightly richer in total phenolic content than aqueous extract E2. On the other hand, the highest level of total flavonoids was found in E2 aqueous extract among E1 and E3 extracts (P < 0.05), while a similar flavonoid content was found in E1 and E3 (Table 1). The most abundant constituent of all three extracts was RA, with a higher yield in ethanolic extracts E1 and E3 than in aqueous extract E2. Characteristically, ethanolic extracts E1 and E3 contained higher levels of phenolic acids (trans-cinnamic acid, caffeic acid, p-coumaric acid, chlorogenic acid, RA, and ferulic acid) than aqueous extract E2. Gallic acid content was highest in E1 among E2 and E3. On the other hand, the highest content of flavonoid quercetin was found in E2 among E1 and E3 (Table 1, Figure 1).
Sample (mg/g d.e.) | E1 | E2 | E3 | |||
---|---|---|---|---|---|---|
X | U | X | U | X | U | |
Phenolic acids | ||||||
Trans-cinnamic acid | 1.28A | 0.14 | 0.37A,B | 0.04 | 1.34B | 0.15 |
Caffeic acid | 0.44A | 0.02 | 0.19A,B | 0.01 | 0.45B | 0.02 |
P-coumaric acid | 0.30A | 0.03 | 0.12A,B | 0.01 | 0.34B | 0.03 |
Chlorogenic acid | 8.86A | 0.44 | 4.29A,B | 0.21 | 8.78B | 0.44 |
Rosmarinic acid | 107.22A | 6.43 | 42.38A,B | 2.54 | 109.44B | 6.57 |
Ferulic acid | 0.34A | 0.02 | 0.22A,B | 0.01 | 0.36B | 0.02 |
Gallic acid | 0.77A,B | 0.12 | 0.07A,C | 0.01 | 0.11B,C | 0.02 |
Flavonoids | ||||||
Quercetin | 0.69A,B | 0.05 | 1.84A,C | 0.13 | 1.08B,C | 0.08 |
Rutin | < LOD | < LOD | < LOD | |||
Quercitrin | < LOD | < LOD | < LOD | |||
Total phenolic content (mg GAE/g d.e.) | 73.39 ± 6.25 | 69.39 ± 6.79 | 73.19 ± 4.09 | |||
Total flavonoid content (mg QE/g d.e.) | 6.23 ± 0.39 A | 10.95 ± 0.48 A,B | 6.38 ± 0.49 B |
Chromatograms of samples E1 (blue line), E2 (red line), and E3 (green line) with detection at (A) 280 nm, (B) 330 nm, and (C) 350 nm. Identified compounds: 1- trans-cinnamic acid, 2- caffeic acid, 3- p-coumaric acid, 4- chlorogenic acid, 5- rosmarinic acid, 6- ferulic acid, 7- gallic acid, and 8- quercetin.
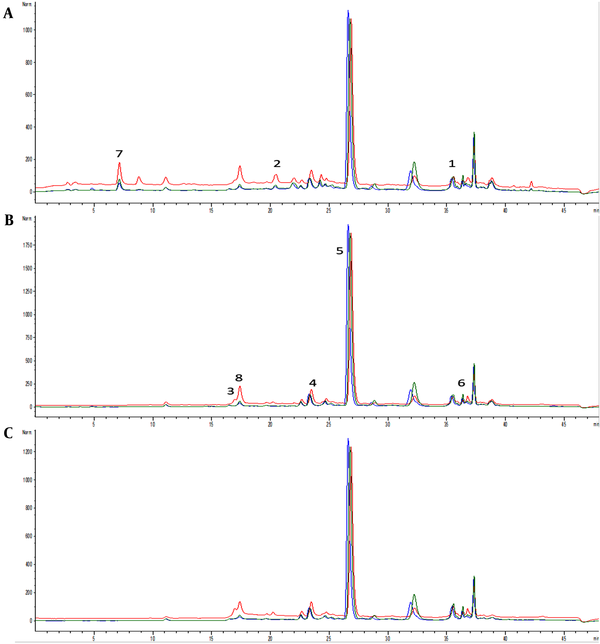
4.2. Antioxidant Potential of Melissa officinalis Extracts
Table 2 depicts the antioxidant potential of the investigated extracts using five different antioxidant tests. The E2 and E3 extracts were shown to have better DPPH-neutralizing activity than E1, while the E1 extract had the best potency to neutralize OH radicals. All three extracts had a similar effect on NO, while the E2 aqueous extract showed the least antioxidant capacity for lipid peroxidation among ethanolic extracts E1 and E3. On the other hand, E1 showed the best antioxidant potential in the FRAP assay (Table 2).
In Vitro Antioxidant Activity of the Investigated Lemon Balm Extracts and Positive Control Substances a, b, c
Samples | Assay | ||||
---|---|---|---|---|---|
DPPH IC50 | OH IC50 | NO IC50 | LP IC50 | FRAP (mg AAE/g d.e.) | |
E1 | 9.95 ± 0.39 A,B | 39.99 ± 3.14 A,B | 36.67 ± 3.75 | 50.54 ± 4.16 A,B | 341.71 ± 42.69 B |
E2 | 4.76 ± 0.48 A | 55.97 ± 4.18 A,C | 35.34 ± 3.11 | 81.10 ± 6.47 A,C | 329.06 ± 23.75 C |
E3 | 4.91 ± 0.49 B | 61.34 ± 4.87 B,C | 32.87 ± 3.01 | 58.53 ± 5.15 B,C | 294.39 ± 11.67 B,C |
AA | / | 2.03 ± 0.39* | / | / | / |
PG | 0.67 ± 0.02* | 8.94 ± 0.45* | 9.12 ± 0.27* | / | / |
BHT | / | 0.04 ± 0.00* | / | 7.08 ± 0.23* | / |
4.3. In Vivo Evaluation of Anti-inflammatory Effects of Melissa officinalis Extracts
Treatment with E1200 significantly reduced edema diameter in the second hour after carrageenan administration compared to the control group (P < 0.05). This effect persisted until the end of the observed period (the sixth hour) and was similar to standard anti-inflammatory drug indomethacin (63.89%), while lower doses of E1 did not achieve an anti-inflammatory effect at any measurement times. On the other hand, aqueous extract E2100 exhibited edema reduction at the fifth hour, also persisting at the sixth hour (P < 0.05), similar to the indomethacin group (69.44%), while the highest dose of this extract E2200 significantly reduced paw edema earlier, in the second hour post-carrageenan; this effect persisted until the end of the observed period, similar to the indomethacin group (61.11%). In contrast, when it comes to E3, only low and medium doses exhibited edema-reducing effects. Besides, E350 induced the fastest edema reduction in the first hour, while medium-dose E350 acted beginning from the third hour post-carrageenan (P < 0.05). In both of these groups, the anti-inflammatory effect persisted until the sixth hour (Table 3).
Group | 1 h | 2 h | 3 h | 4 h | 5 h | 6 h |
---|---|---|---|---|---|---|
E150 | ||||||
Paw edema reduction (mm) | 0.52 ± 0.08 | 1.18 ± 0.10 # | 0.97 ± 0.08 # | 0.83 ± 0.08 # | 0.58 ± 0.08 # | 0.38 ± 0.15 # |
Edema inhibition (%) | 13.89 | 16.47 | 20.55 | 36.36 | 18.60 | 36.11 |
E1100 | ||||||
Paw edema reduction (mm) | 0.48 ± 0.08 | 1.30 ± 0.14 # | 1.10 ± 0.16 # | 0.89 ± 0.15 # | 0.66 ± 0.15 # | 0.36 ± 0.09 # |
Edema inhibition (%) | 20.00 | 8.24 | 9.59 | 2.91 | 7.91 | 40.00 |
E1200 | ||||||
Paw edema reduction (mm) | 0.60 ± 0.13 | 1.10 ± 0.24 *# | 0.93 ± 0.29 # | 0.73 ± 0.25 # | 0.50 ± 0.15 # | 0.22 ± 0.20 * |
Edema inhibition (%) | 0.00 | 22.35 | 23.29 | 20.00 | 30.23 | 63.89 |
E250 | ||||||
Paw edema reduction (mm) | 0.59 ± 0.08 | 1.38 ± 0.10 # | 1.17 ± 0.08 # | 0.90 ± 0.10 # | 0.67 ± 0.08 # | 0.48 ± 0.08 # |
Edema inhibition (%) | 1.68 | 2.35 | 4.11 | 2.91 | 2.91 | 19.44 |
E2100 | ||||||
Paw edema reduction (mm) | 0.35 ± 0.29 | 1.18 ± 0.08 # | 1.07 ± 0.2 # | 0.75 ± 0.33 # | 0.33 ± 0.23 * | 0.18 ± 0.17 * |
Edema inhibition (%) | 41.67 | 16.47 | 12.33 | 18.18 | 53.49 | 69.44 |
E2200 | ||||||
Paw edema reduction (mm) | 0.38 ± 0.17 | 0.70 ± 0.26 * | 0.62 ± 0.19 * | 0.48 ± 0.19 * | 0.36 ± 0.21 # | 0.23 ± 0.23 * |
Edema inhibition (%) | 36.11 | 50.59 | 49.32 | 47.27 | 49.77 | 61.11 |
E350 | ||||||
Paw edema reduction (mm) | 0.25 ± 0.16 * | 0.95 ± 0.34 * | 0.85 ± 0.27 * | 0.53 ± 0.36 * | 0.33 ± 0.29 * | 0.27 ± 0.10 * |
Edema inhibition (%) | 58.33 | 32.94 | 30.14 | 41.82 | 53.49 | 55.56 |
E3100 | ||||||
Paw edema reduction (mm) | 0.38 ± 0.22 | 1.02 ± 0.29 | 0.80 ± 0.25 * | 0.63 ± 0.20 * | 0.47 ± 0.23 * | 0.35 ± 0.19 * |
Edema inhibition (%) | 36.11 | 28.24 | 34.25 | 31.27 | 34.88 | 41.67 |
E3200 | ||||||
Paw edema reduction (mm) | 0.40 ± 0.24 | 0.98 ± 0.26 | 0.83 ± 0.25 | 0.63 ± 0.25 | 0.52 ± 0.18 | 0.42 ± 0.19 |
Edema inhibition (%) | 33.33 | 30.82 | 31.51 | 25.82 | 27.91 | 30.56 |
IND | ||||||
Paw edema reduction (mm) | 0.56 ± 0.11 | 0.60 ± 0.12 * | 0.44 ± 0.11 * | 0.36 ± 0.09 * | 0.18 ± 0.13 * | 0.14 ± 0.09 * |
Edema inhibition (%) | 6.67 | 57.65 | 63.84 | 60.73 | 74.88 | 76.67 |
CTRL | ||||||
Paw edema reduction (mm) | 0.60 ± 0.17 | 1.42 ± 0.21 # | 1.22 ± 0.19 # | 0.92 ± 0.17 # | 0.72 ± 0.13 # | 0.60 ± 0.11 # |
4.4. Evaluation of Redox Status Post-carrageenan Application
The level of NO-2 was significantly reduced in groups treated with IND and E2 and E3 extracts at all three doses compared to the control group (P < 0.05). However, the strongest drop was observed in E2 among the other two extracts, without dose dependence. Besides, E1 at all doses was similar to CTRL and significantly decreased NO-2 compared to IND. Also, NO-2 levels were significantly higher at all three doses of E1 than at the same doses of E2 and E3. Additionally, IND and all extracts showed a significant decline in H2O2 production compared to the control group (P < 0.05).
Interestingly, the highest dose of 200 mg/kg exhibited more H2O2 reduction than the medium dose in all three extracts. However, the effects of all three examined extracts did not significantly differ in general. The E1 extract significantly reduced the lipid peroxidation index measured as TBARS compared to control, and this effect was dose-dependent (Table 4). An opposite trend was noticed in the E2 group, and a significant reduction of TBARS was observed at low and medium doses of E250 and E2100. However, in the E3 group, a significant reduction in TBARS was achieved only with lower doses of 50 and 100 mg/kg. Additionally, the IND group significantly reduced TBARS compared to CTRL. The most prominent reduction of TBARS was observed in E1200 among E2200 and E3200 (P < 0.05; Figure 2, Table 4).
Effects of applied extracts on pro-oxidant parameters: (A) hydrogen peroxide (H2O2); (B) nitrites (NO-2); (C) index of lipid peroxidation (TBARS). E150, E1100, and E1200: Rats treated with 50, 100, and 200 mg/kg of Melissa officinalis ethanolic (70%) macerate; E250, E2100, and E2200: Rats treated with 50, 100, and 200 mg/kg of M. officinalis aqueous extract obtained under reflux of solvent; E350, E3100, and E3200: Rats treated with 50, 100, and 200 mg/kg of M. officinalis ethanolic (70%) extract obtained under reflux of solvent; a, Significant differences compared to the control group at the level of P < 0.05; b, Significant differences compared to indomethacin (IND) group at the level of P < 0.05. Data are expressed as means ± standard deviation (SD)
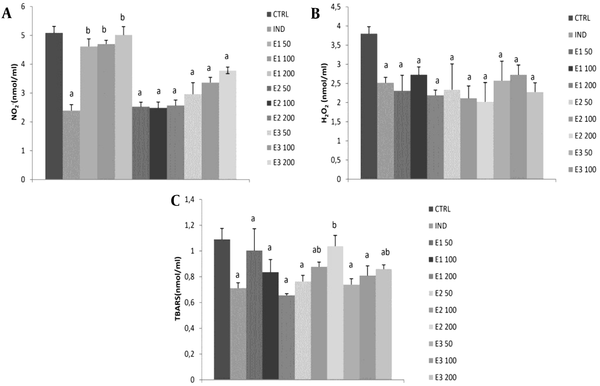
All three doses of E1 ethanolic macerate significantly increased SOD activity compared to the control and other two extracts, E2 and E3 (P < 0.05), with no dose-dependent effect (Figure 3, Table 4). However, these values did not significantly differ from the indomethacin group. The CAT activity was significantly higher only in the indomethacin group than in the control group. All of the three applied extracts, E1, E2, and E3 at all doses, as well as IND, significantly increased GSH levels compared to control. A more GSH increment was observed at medium doses in groups E2100 and E3100 than in group E1100, while the highest dose E3200 exhibited a more increase in GSH than the other two extracts (P < 0.05).
Effects of the investigated extracts on the parameters of antioxidant defense system: (A) superoxide dismutase (SOD); (B) catalase (CAT); (C) reduced glutathione (GSH). E150, E1100, and E1200: Rats treated with 50, 100, and 200 mg/kg of Melissa officinalis ethanolic (70%) macerate; E250, E2100, and E2200: Rats treated with 50, 100, and 200 mg/kg of M. officinalis aqueous extract obtained under reflux of solvent; E350, E3100, and E3200: Rats treated with 50, 100, and 200 mg/kg of M. officinalis ethanolic (70%) extract obtained under reflux of solvent; a Significant difference compared to the control group; b Significant difference compared to the indomethacin (IND) group. Data are expressed as means ± standard deviation (mean ± SD)
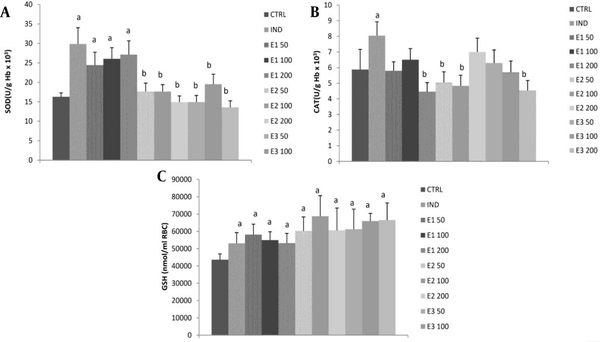
The Effects of Melissa officinalis Extracts on Redox Status After Carrageenan-Induced Paw Edema Test a
Groups | H2O2 (nmol/mL) | NO-2 (nmol/mL) | TBARS (nmol/mL) | SOD (U/g Hb × 103) | CAT (U/g Hb × 103) | GSH (nmol/mL RBC) |
---|---|---|---|---|---|---|
E150 | 2.31 ± 0.41 | 4.62 ± 0.26 # | 1.00 ± 0.17 *# | 24.42 ± 3.32 | 5.79 ± 0.58 | 58079.31 ± 6096.09 |
E1100 | 2.49 ± 0.20 # | 4.70 ± 0.13 | 0.84 ± 0.10 # | 26.05 ± 2.87 | 6.50 ± 0.71 | 54904.18 ± 4885.96 |
E1200 | 2.19 ± 0.14 * | 5.02 ± 0.29 * | 0.66 ± 01 * | 27.13 ± 3.53 | 4.46 ± 0.58 * | 53148.98 ± 5716.80 |
E250 | 2.34 ± 0.67 | 2.53 ± 0.16 A | 0.76 ± 0.05 # A | 17.64 ± 2.15 A | 5.05 ± 0.68 | 60248.66 ± 8089.83 |
E2100 | 2.11 ± 0.32 # | 2.48 ± 0.20 A | 0.88 ± 0.04 # | 17.64 ± 1.77 A | 4.83 ± 0.67 # | 68728.83 ± 11923.66 #A |
E2200 | 2.02 ± 0.50 * | 2.57 ± 0.19 A | 1.04 ± 0.08 * A | 14.92 ± 1.59 A | 7.00 ± 0.89 *A | 60643.09 ± 12874.12 * |
E350 | 2.58 ± 0.51 | 2.96 ± 0.39 #A | 0.74 ± 0.05 A,B | 14.92 ± 1.70 A | 6.29 ± 0.84 # | 61234.73 ± 11628.40 |
E3100 | 2.73 ± 0.25 #B | 3.37 ± 0.18 #A,B | 0.81 ± 0.08 | 19.54 ± 2.54 # | 5.70 ± 0.72 | 65908.68 ± 4515.11 A |
E3200 | 2.28 ± 0.24 * | 3,79 ± 0.11 *A,B | 0.86 ± 0.03 A,B | 13.57 ± 1.70 *A | 4.54 ± 0.64 B | 66559.49 ± 9884.11 A |
5. Discussion
This study aimed to determine the chemical composition and in vitro antioxidant capacity of three different МОЕs. Besides, their in vivo anti-inflammatory potential and effects on carrageenan-induced oxidative stress were examined.
Many factors influence extraction efficacy and yields, including extraction method, temperature, solvent-to-material ratio, extraction time, solvent composition and polarity, and extraction pressure (18). In our study, the highest extraction yield was obtained by extraction with water as a polar solvent under reflux (26.8%), which is in line with other research investigating aqueous MOEs of originating from Germany, France, and Tunisia (26.5 - 31%) (19). Also, 70% ethanol solvent achieved a higher yield under reflux (8.3%) than in the maceration method (1.5%), which can be explained by the fact that extraction under reflux of the solvent at the boiling point is one of the most efficient methods for the isolation of antioxidant components of plants, contrary to maceration at room temperature.
Total phenolic and flavonoid content data reported here are similar to those of other studies for aqueous and ethanolic MOEs (20). However, there are also studies with different observations. For example, the phenolic content of MO ethanolic macerate in our study (73.39 ± 6.25 GAE/g d.e) is superior to that of Safaeian et al., where the same method of extraction was used (28.84 ± 4.04 GAE/g d.e), possibly due to different origin of MO (Iran) (21). In line with other research on MOEs, we detected phenolic acids such as RA, trans-cinammic, chlorogenic, gallic, ferulic, p-coumaric, and caffeic acid together with flavonoid quercetin in the examined extracts. In the present study, RA was the main compound in all three examined MO extracts, which is consistent with earlier studies (4, 5, 22). The highest content of RA was observed in ethanolic E3 extract (109.44 mg/g d.e), while lower content in aqueous extract E2 (42.28 mg/g d.e) may be due to its higher solubility in ethanol than in water. Although the yield was highest in aqueous extract E2, the content of RA was found to be highest in E3 ethanolic extract, indicating that other components besides phenolics were extracted. This may be because water, as a universal polar solvent, extracts more ballast substances such as carbohydrates and proteins than ethanol, which can be attributed to the higher solubility of proteins and carbohydrates in water than in ethanol (23, 24).
Interestingly, we found the highest flavonoid content in aqueous extract, while the most abundant flavonoid component was quercetin, with the highest content in E2 among E1 and E3 (1.84 mg/g d.e). However, other authors that investigated aqueous MOE did not detect quercetin. However, they detected other flavonoids such as hesperetin and rutin, which were not present in the examined MOEs E1 E2, and E3 in our study (25). Finally, unlike others (5), we did not detect flavonoid glycosides rutin and quercitrin.
Melissa officinalis is a plant well known in the literature for its antioxidant action, which is described and investigated in many studies both in vitro and in vivo in different oxidative stress-related pathologies (2, 3). That is why we evaluated the ROS scavenging capacity of the investigated MOEs in vitro and conducted a post-carrageenan paw edema test in vivo. The ability of the investigated MO extracts to scavenge NO could be crucial for human health since it has been suggested that NO has a crucial role in the pathophysiology and progression of many oxidative stress-related diseases and pathologies such as cardiovascular disease (atherosclerosis and ischemia-reperfusion injury), metabolic disease (diabetes), neurodegenerative disorders, aging, and cancer. Aqueous E2 and ethanolic E3 MOEs showed NO scavenging capacity in vitro and in vivo in the carrageenan paw edema test, in the terms of NO-2 reduction, compared to control, while E1 MO macerate did not affect NO in vivo post-carrageenan. The possible reason may be related to the composition of the extracts since E1 had the lowest content of quercetin, which is one of the most studied flavonoids proved to exhibit health-promoting effects through extracellular NO scavenging and increase NO intracellular biological activity (26).
Additionally, the inhibition of lipid peroxidation in vivo was observed in ethanolic E1 and E2 extracts, while E2 aqueous extract reduced TBARS only at low and medium doses, possibly because flavonoids such as quercetin, the most abundant in E2, can also act pro-oxidant and toxic at higher doses (27). In vitro results of the inhibition of lipid peroxidation also correlate with these findings. Other authors also highlighted the amelioration of oxidative stress in the doxorubicin-induced cardiotoxicity model through the reduction of malondialdehyde (MDA) induced by ethanolic (70%) MO macerate (9). Aqueous MOE applied at four two-fold increasing doses starting from 50 to 400 mg/kg was proved to decrease the MDA level in the carrageenan-induced paw edema model, while we found a significant reduction in TBARS only at lower doses E250 and E2100 but not at E2200 mg/kg (28). Additionally, all three MO extracts decreased H2O2 formation in vivo, correlating with the previous research that revealed MO protective effect in H2O2-induced toxicity on cell lines (21).
Moreover, MO aqueous and ethanolic extracts are proven to increase antioxidant enzyme activity in different animal models (29, 30). In line with our results is the finding of the study where aqueous MOE at doses of 100, 200, and 400 mg/kg was shown to increase reduced GSH post-carrageenan injection (29). Unlike others, we reported no effect of MO extracts on antioxidant enzyme CAT, while only ethanolic macerate of MO (E1) increased SOD activity. The increased SOD activity in the E1 group may be connected with the highest content of gallic acid (31).
To thoroughly investigate the therapeutic potential of MO extracts, another objective of this study was to determine their in vivo anti-inflammatory potential. Nonetheless, limited data are available regarding the influence of aqueous and ethanolic MO extracts on inflammation. For this purpose, we used the carrageenan-induced paw edema model, which is one of the most frequently used models to determine the anti-inflammatory properties of natural products. Paw edema formation after carrageenan injection is described as a biphasic inflammation event (32). Namely, the initial early phase occurs 0 - 2.5 h post-carrageenan injection that is associated with the release of mediators such as histamine, serotonin, and leukotrienes and increased vascular permeability (11, 33). Leukocytes, endothelial cells, and sensory nerve cells form another mediator, nitric oxide (NO), at the site of inflammation (34). The second phase happens 2.5 - 6 h post-carrageenan injection that is attributed to prostaglandins, proinflammatory (TNF-α, IL-1β, and IL-6), and anti-inflammatory cytokines (IL-10) release, leading to edema formation and bradykinin and neutrophil infiltration. Notably, the peak of COX-2 expression occurs one hour after the topical application of carrageenan (34). This correlates with our results showing that the peak edema formation occurs at the second hour in all groups, confirming the development of local and acute inflammatory reactions (35).
Additionally, in our study, ethanolic macerate at a dose of 200 mg/kg, aqueous extract doses of 100 mg/kg and 200 mg/kg, and ethanolic reflux at doses of 50 and 100 mg/kg showed the most pronounced edema reduction in the sixth hour post-carrageenan. Also, the observed effects of the extracts were time-dependent but not dose-dependent, indicating that the least inhibition of paw edema was in the first hours so that after the expiration of the sixth hour, the most pronounced reduction of edema was manifested. However, only the highest doses of E1200 and E2100 induced a similar degree of inhibition of paw edema achieved by the reference drug indomethacin.
Birdane et al. examined in vivo anti-inflammatory effect of aqueous MOE using the same model and implicated that aqueous MOE at all doses of 50, 100, 200, and 400 mg/kg significantly decreased the inflammatory response at 5 h post-carrageenan injection (28). However, we found that only doses of 100 and 200 mg/kg of E2 decreased the inflammatory response and this was even more pronounced at 6 h post-carrageenan, similar to the effect of indomethacin. A previous study highlighted the substantial anti-inflammatory activity of MO essential oil tested at two doses, 200 and 400 mg/kg (61.76% and 70.58% at the sixth hour), and the reduction and inhibition of carrageenan-induced paw edema in both early and late phases of inflammation, most likely induced by terpenoid component of citrals (neral and geranial) via the inhibition of TNF-alpha. However, these effects were not detected in the present study, since the examined extracts did not contain the oxygenated monoterpenes which were present in the essential oil of lemon balm (11).
Given that no data are available regarding the mechanisms of MOE anti-inflammatory activity, the observed effect in our study may be considered a consequence of the synergistic action of phenols and flavonoids. Also, RA is an important and the most abundant compound of MOE, known to possess pleiotropic anti-inflammatory properties such as decreased production of TNFα, NF-κB, and ROS that could have contributed to the achieved effects of investigated extracts, not solely antioxidant effect (2, 35). Additionally, RA per se was tested and proved to reduce carrageenan-induced paw edema by over 60% at the sixth hour (36). Another component that might have contributed to the reduction of paw edema, especially after aqueous MO extract administration, is quercetin via the inhibition of cyclooxygenase (COX) and lipoxygenase (LOX) (37). Chlorogenic acid, also found in the examined extract, may be responsible for the anti-inflammatory effect probably due to the net effect of different mechanisms such as strong COX-2 inhibition, along with increased antioxidant effect and strong activation of NF-κB (38).
Moreover, gallic acid, also detected in the examined extracts, possesses anti-inflammatory action via several mechanisms involving MAPK and NF-κB signaling pathways and reduction of inflammatory cytokines, chemokines, the release of adhesion molecules, and reduction of cell infiltration (39). As far as we know, this study is the first one comparing the properties of aqueous and ethanolic MOEs, giving new information on how different polar solvents and extraction types influence the biological activities of MOEs. Insufficient data are available regarding the anti-inflammatory properties of MOEs; thus, this study may help elucidate possible mechanisms interfering with antioxidant action. However, additional research is required to elucidate the exact cellular mechanism of this action.
A possible limitation of this study is that we investigated only polar solvents (water and ethanol) for MO extraction. Additional limitation is that we did not follow the effects of examined MOEs longer after carrageenan application, for example 24, 48, 72 hours or more.
5.1. Conclusions
The study indicated that lemon balm polar solvent extracts could scavenge a wide range of free radicals in vivo and in vitro. This is of great interest as it indicates that these extracts may prevent oxidative damage in vivo by neutralizing or chelating the reactive species. We found that all three MOEs could reduce the production of pro-oxidants, such as H2O2 and TBARS, and provide antioxidant protection via GSH increment, probably due to the synergistic action of the components found in the examined extracts. Another important finding is that ethanolic macerate and aqueous MOEs were proven as anti-inflammatory agents in the carrageenan paw edema model, which could be attributed to their phytochemical content. The high content of gallic acid and RA provided the most prominent anti-inflammatory response in E1, while the high content of quercetin might be responsible for this action in E2. These data imply the possible future usage of MOEs to prevent inflammatory and oxidative stress-related diseases.
References
-
1.
Zarei A, Changizi-Ashtiyani S, Taheri S, Hosseini N. A Brief Overview of the Effects of Melissa officinalis L. Extract on the Function of Various Body Organs. Zahedan J Res Med Sci. 2015;15:29-34. https://doi.org/10.17795/zjrms1007.
-
2.
Draginic N, Jakovljevic V, Andjic M, Jeremic J, Srejovic I, Rankovic M, et al. Melissa officinalis L. as a Nutritional Strategy for Cardioprotection. Front Physiol. 2021;12:661778. [PubMed ID: 33967832]. [PubMed Central ID: PMC8100328]. https://doi.org/10.3389/fphys.2021.661778.
-
3.
Shakeri A, Sahebkar A, Javadi B. Melissa officinalis L. - A review of its traditional uses, phytochemistry and pharmacology. J Ethnopharmacol. 2016;188:204-28. [PubMed ID: 27167460]. https://doi.org/10.1016/j.jep.2016.05.010.
-
4.
Ibragić S, Salihović M, Tahirović I, Toromanović J. Quantification of some phenolic acids in the leaves of Melissa officinalis L. from Turkey and Bosnia. Bull Chem Tech Bosnia Herzegovina. 2014;42:47-50.
-
5.
Arceusz A, Wesolowski M, Ulewicz-Magulska B. Flavonoids and Phenolic Acids in Methanolic Extracts, Infusions and Tinctures from Commercial Samples of Lemon Balm. Natural Prod Commun. 2015;10(6):977-81. https://doi.org/10.1177/1934578x1501000645.
-
6.
Aharizad S, Rahimi MH, Moghadam M, Mohebalipour N. Study of genetic diversity in lemon balm (Melissa officinalis l.) populations based on morphological traits and essential oils content. Ann Biol Res. 2012;3(12):5748-53.
-
7.
Mimica-Dukic N, Bozin B, Sokovic M, Simin N. Antimicrobial and antioxidant activities of Melissa officinalis L. (Lamiaceae) essential oil. J Agric Food Chem. 2004;52(9):2485-9. [PubMed ID: 15113145]. https://doi.org/10.1021/jf030698a.
-
8.
Sedighi M, Faghihi M, Rafieian-Kopaei M, Rasoulian B, Nazari A. Cardioprotective effect of ethanolic leaf extract of melissa officinalis L against regional ischemia-induced arrhythmia and heart injury after five days of reperfusion in rats. Iran J Pharm Res. 2019;18(3):1530-42.
-
9.
Hamza AA, Ahmed MM, Elwey HM, Amin A. Melissa officinalis Protects against Doxorubicin-Induced Cardiotoxicity in Rats and Potentiates Its Anticancer Activity on MCF-7 Cells. PLoS One. 2016;11(11). e0167049. [PubMed ID: 27880817]. [PubMed Central ID: PMC5120835]. https://doi.org/10.1371/journal.pone.0167049.
-
10.
Drozd JANINA, Anuszewska E. The effect of the Melissa officinalis extract on immune response in mice. Acta Pol Pharm. 2003;60(6):467-70.
-
11.
Bounihi A, Hajjaj G, Alnamer R, Cherrah Y, Zellou A. In Vivo Potential Anti-Inflammatory Activity of Melissa officinalis L. Essential Oil. Adv Pharmacol Sci. 2013;2013:101759. [PubMed ID: 24381585]. [PubMed Central ID: PMC3870089]. https://doi.org/10.1155/2013/101759.
-
12.
Hijazi A, Al Masri DS, Farhan H, Nasser M, Rammal H, Annan H. Effect of different ethanol concentrations, using different extraction techniques, on the antioxidant capacity of Lebanese Eryngium creticum. Int J Pharm Chem Biol Sci. 2015;3(2):262-71.
-
13.
Bozin B, Kladar N, Grujic N, Anackov G, Samojlik I, Gavaric N, et al. Impact of origin and biological source on chemical composition, anticholinesterase and antioxidant properties of some St. John's wort species (Hypericum spp., Hypericaceae) from the Central Balkans. Molecules. 2013;18(10):11733-50. [PubMed ID: 24071982]. [PubMed Central ID: PMC6270400]. https://doi.org/10.3390/molecules181011733.
-
14.
Salaj N, Kladar N, Čonić BS, Jeremić K, Barjaktarović J, Hitl M, et al. Stabilization of sunflower and olive oils with savory (Satureja kitaibelii, Lamiaceae). J Food Nutr Res. 2020;59(3):259-71.
-
15.
Bozin B, Mimica-Dukic N, Simin N, Anackov G. Characterization of the volatile composition of essential oils of some lamiaceae spices and the antimicrobial and antioxidant activities of the entire oils. J Agric Food Chem. 2006;54(5):1822-8. [PubMed ID: 16506839]. https://doi.org/10.1021/jf051922u.
-
16.
Morris CJ. Carrageenan-induced paw edema in the rat and mouse. Methods Mol Biol. 2003;225:115-21. [PubMed ID: 12769480]. https://doi.org/10.1385/1-59259-374-7:115.
-
17.
Bradic J, Zivkovic V, Srejovic I, Jakovljevic V, Petkovic A, Turnic TN, et al. Protective Effects of Galium verum L. Extract against Cardiac Ischemia/Reperfusion Injury in Spontaneously Hypertensive Rats. Oxid Med Cell Longev. 2019;2019:4235405. [PubMed ID: 30863479]. [PubMed Central ID: PMC6378796]. https://doi.org/10.1155/2019/4235405.
-
18.
Yoo G, Lee IK, Park S, Kim N, Park JH, Kim SH. Optimization of Extraction Conditions for Phenolic Acids from the Leaves of Melissa officinalis L. Using Response Surface Methodology. Pharmacogn Mag. 2018;14(54):155-61. [PubMed ID: 29720824]. [PubMed Central ID: PMC5909308]. https://doi.org/10.4103/pm.pm_70_17.
-
19.
Souihi M, Ben Ayed R, Trabelsi I, Khammassi M, Ben Brahim N, Annabi M. Plant Extract Valorization of Melissa officinalis L. for Agroindustrial Purposes through Their Biochemical Properties and Biological Activities. J Chem. 2020;2020:1-12. https://doi.org/10.1155/2020/9728093.
-
20.
Garcia-Risco MR, Mouhid L, Salas-Perez L, Lopez-Padilla A, Santoyo S, Jaime L, et al. Biological Activities of Asteraceae (Achillea millefolium and Calendula officinalis) and Lamiaceae (Melissa officinalis and Origanum majorana) Plant Extracts. Plant Foods Hum Nutr. 2017;72(1):96-102. [PubMed ID: 28101823]. https://doi.org/10.1007/s11130-016-0596-8.
-
21.
Safaeian L, Sajjadi SE, Javanmard SH, Montazeri H, Samani F. Protective effect of Melissa officinalis extract against H2O2-induced oxidative stress in human vascular endothelial cells. Res Pharm Sci. 2016;11(5):383-9. [PubMed ID: 27920820]. [PubMed Central ID: PMC5122827]. https://doi.org/10.4103/1735-5362.192488.
-
22.
Lin J, Chen Y, Lee Y, Rolis Hou C, Chen F, Yang D. Antioxidant, anti-proliferative and cyclooxygenase-2 inhibitory activities of ethanolic extracts from lemon balm (Melissa officinalis L.) leaves. LWT. 2012;49(1):1-7. https://doi.org/10.1016/j.lwt.2012.04.009.
-
23.
Jahani R, Behzad S, Saffariha M, Toufan Tabrizi N, Faizi M. Sedative-hypnotic, anxiolytic and possible side effects of Salvia limbata C. A. Mey. Extracts and the effects of phenological stage and altitude on the rosmarinic acid content. J Ethnopharmacol. 2022;282:114630. [PubMed ID: 34517061]. https://doi.org/10.1016/j.jep.2021.114630.
-
24.
Do QD, Angkawijaya AE, Tran-Nguyen PL, Huynh LH, Soetaredjo FE, Ismadji S, et al. Effect of extraction solvent on total phenol content, total flavonoid content, and antioxidant activity of Limnophila aromatica. J Food Drug Anal. 2014;22(3):296-302. [PubMed ID: 28911418]. https://doi.org/10.1016/j.jfda.2013.11.001.
-
25.
Ordaz JJ, Hernández JM, Ramírez-Godínez J, Castañeda-Ovando A, González-Olivares LG, Contreras-López E. Bioactive compounds in aqueous extracts of lemon balm (Melissa officinalis) cultivated in Mexico. Arch Latinoam Nutr. 2018;68(3):79-90.
-
26.
Lopez-Lopez G, Moreno L, Cogolludo A, Galisteo M, Ibarra M, Duarte J, et al. Nitric oxide (NO) scavenging and NO protecting effects of quercetin and their biological significance in vascular smooth muscle. Mol Pharmacol. 2004;65(4):851-9. [PubMed ID: 15044614]. https://doi.org/10.1124/mol.65.4.851.
-
27.
Skibola CF, Smith MT. Potential health impacts of excessive flavonoid intake. Free Radic Biol Med. 2000;29(3-4):375-83. https://doi.org/10.1016/s0891-5849(00)00304-x.
-
28.
Birdane YO, Buyukokuroglu ME, Birdane FM, Cemek M, Yavuz H. Anti-inflammatory and antinociceptive effects of Melissa officinalis L. in rodents. Rev Med Vet. 2007;158(2):75-81.
-
29.
Saberi A, Abbasloo E, Sepehri G, Yazdanpanah M, Mirkamandari E, Sheibani V, et al. The Effects of Methanolic Extract of Melissa officinalis on Experimental Gastric Ulcers in Rats. Iran Red Crescent Med J. 2016;18(7). https://doi.org/10.5812/ircmj.24271.
-
30.
Martins EN, Pessano NT, Leal L, Roos DH, Folmer V, Puntel GO, et al. Protective effect of Melissa officinalis aqueous extract against Mn-induced oxidative stress in chronically exposed mice. Brain Res Bull. 2012;87(1):74-9. [PubMed ID: 22020131]. https://doi.org/10.1016/j.brainresbull.2011.10.003.
-
31.
Sohrabi F, Dianat M, Badavi M, Radan M, Mard SA. Does gallic acid improve cardiac function by attenuation of oxidative stress and inflammation in an elastase-induced lung injury? Iran J Basic Med Sci. 2020;23(9):1130-8.
-
32.
Cong HH, Khaziakhmetova VN, Zigashina LE. Rat paw oedema modeling and NSAIDs: Timing of effects. Int J Risk Saf Med. 2015;27 Suppl 1:S76-7. [PubMed ID: 26639722]. https://doi.org/10.3233/JRS-150697.
-
33.
Ding S, Jiang H, Fang J. Regulation of Immune Function by Polyphenols. J Immunol Res. 2018;2018:1264074. [PubMed ID: 29850614]. [PubMed Central ID: PMC5925142]. https://doi.org/10.1155/2018/1264074.
-
34.
Posadas I, Bucci M, Roviezzo F, Rossi A, Parente L, Sautebin L, et al. Carrageenan-induced mouse paw oedema is biphasic, age-weight dependent and displays differential nitric oxide cyclooxygenase-2 expression. Br J Pharmacol. 2004;142(2):331-8. [PubMed ID: 15155540]. [PubMed Central ID: PMC1574940]. https://doi.org/10.1038/sj.bjp.0705650.
-
35.
Saini AK, Goyal R, Gauttam VK, Kalia AN. Evaluation of anti-inflammatory potential of Ricinus communis Linn leaves extracts and its flavonoids content in Wistar rats. J Chem Pharm Res. 2010;2(5):690-5.
-
36.
Rocha J, Eduardo-Figueira M, Barateiro A, Fernandes A, Brites D, Bronze R, et al. Anti-inflammatory effect of rosmarinic acid and an extract of Rosmarinus officinalis in rat models of local and systemic inflammation. Basic Clin Pharmacol Toxicol. 2015;116(5):398-413. [PubMed ID: 25287116]. https://doi.org/10.1111/bcpt.12335.
-
37.
Morikawa K, Nonaka M, Narahara M, Torii I, Kawaguchi K, Yoshikawa T, et al. Inhibitory effect of quercetin on carrageenan-induced inflammation in rats. Life Sci. 2003;74(6):709-21. [PubMed ID: 14654164]. https://doi.org/10.1016/j.lfs.2003.06.036.
-
38.
Mitrea DR, Malkey R, Florian TL, Filip A, Clichici S, Bidian C, et al. Daily oral administration of chlorogenic acid prevents the experimental carrageenan-induced oxidative stress. J Physiol Pharmacol. 2020;71(1). https://doi.org/10.26402/jpp.2020.1.04.
-
39.
Bai J, Zhang Y, Tang C, Hou Y, Ai X, Chen X, et al. Gallic acid: Pharmacological activities and molecular mechanisms involved in inflammation-related diseases. Biomed Pharmacother. 2021;133:110985. [PubMed ID: 33212373]. https://doi.org/10.1016/j.biopha.2020.110985.