Abstract
Background:
As an environmental contaminant, Arsenic (As) poses many risks to human health. Increased Oxidative Stress (OS) and decreased antioxidant cell defense are the suggested mechanisms of carcinogenicity and toxicity of As. As a powerful antioxidant and water-soluble compound, vitamin C protects cells and tissues against oxidation and has a wide range of healing properties.Objectives:
The current study aimed to formulate a suitable ascorbic acid (vitamin C) niosome and compare it with vitamin C in preventing As-induced toxicity in HEK-293 cells.Methods:
Various formulas of vitamin C niosomes were prepared by C-SPAN mixed with cholesterol. The physicochemical characteristics of niosomal formulations, including load size, zeta-potential, and the drug release profile, were evaluated in HEK-293 cells. Then, OS biomarkers such as total reactive oxygen species (ROS), malondialdehyde (MDA), catalase (CAT), Antioxidant Capacity (TAC), and superoxide dismutase (SOD) activities determined the protective effects of vitamin C niosomes compared with vitamin C against As-induced toxicity.Results:
The particle size and zeta potential of the optimal vitamin C niosome were 163.2 ± 6.1 nm and 23.3 ± 3.5 mV, respectively. Arsenic increased ROS and MDA levels while decreasing CAT, TAC, and SOD activities in the HEK-293 cell line. Finally, the vitamin C niosome decreased OS and increased antioxidant properties more than vitamin C.Significance:
Vitamin C niosome was more effective than vitamin C in treating As-induced toxicity in vitro.Keywords
1. Background
Arsenic (As) and its compounds are known as group-I carcinogens that cause various problems for human health, including cancers (1). The two known forms of arsenic are As (V) and As (III). The first one is abundantly found in surface water, while the second one is predominantly observed in groundwater and is highly toxic to biological systems (2). Although As is used as an antiseptic in insecticides to preserve hides or manufacturing dyes, the primary source of exposure to As is natural waters worldwide (3). The World Health Organization has considered the maximum permissible level of 10 to 50 µgL-1 for As concentrations in drinking water (4).
Arsenic is highly harmful to humans because of its high accumulation in kidney and liver tissues (5). Studies have shown that long-term exposure to As induces liver, skin, bladder, and lung cancers in humans (1, 6, 7). The mechanism of As toxicity is complex. According to evidence (8), although the activity inhibition of various enzymes such as glutathione reeducates by reaction with sulfhydryl groups of proteins has been attributed to As, its cellular toxicity mechanism is via the generation of reactive oxygen species (ROS) and oxidative stress (OS). The enhanced generation of ROS after As exposure via covalent binding with the membrane lipid of cells can induce lipid peroxidation (LPO) and changes the intrinsic antioxidant defenses of cells, including Catalase (CAT) activity, superoxide dismutase (SOD), antioxidant capacity (TAC), and lactate dehydrogenase (LDH) content in various tissues, leading to harmful effects on different tissues, such as cardiovascular disorders, gastrointestinal tract diseases, and diabetes in humans (9, 10).
A wide range of drug therapies has been proposed to combat As toxicity (11, 12), including natural and industrial antioxidants. Ascorbic acid (vitamin C) is a water-soluble antioxidant compound found in fruits and vegetables that can counteract free radicals and prevent oxidative damage to the cell membrane induced by radicals in the aqueous environment (13). It can regulate cholesterol metabolism and inhibit LDL oxidation as a free radical scavenger in the human body (14). Different studies demonstrated that vitamin C alone or in combination with other vitamins could protect various cells from As toxicity. For example, Ramanathan et al. indicated that the combined administration of alpha-tocopherol and vitamin C prevents As-induced toxicity by decreasing LPO in rat liver and kidney microsomes (15). Similarly, Banerjee et al. reported that ascorbic acid has protective effects against As toxicity and OS (16). Another similar study revealed that ascorbic acid and alpha-tocopherol supplementation could improve As-induced molecular alterations (17).
Despite the abundant applications of ascorbic acid supplementation in many diseases, its usefulness is limited due to its hydrophilic nature, poor stability, and easy degradation in oxidation pathways. Therefore, it is impossible to use this vitamin to treat many diseases, or it needs incredibly high doses to achieve therapeutic effects. Different nanoparticles are designed in nanotechnology studies to overcome these problems. Based on a study, ascorbic acid as a nanoparticle (micellar ascorbic acid) increases the therapeutic effects of ascorbic acid in preclinical animal models by increasing stability, hydrophobic potential, tissue distribution, and biological half-life (18). For instance, in one study, nano ascorbic acid was superior to ascorbic acid concerning neuroprotective properties in experimental studies (19). Despite the beneficial effects of nano ascorbic acid in treating diseases, no study has focused on comparing the relative efficacy of ascorbic acid and ascorbic acid nanoparticles against As-induced toxicity.
2. Objectives
The current study addressed this issue by assessing ROS, MDA level, and various antioxidant activities, including CAT, SOD, and TAC, in HEK-293 cells.
3. Methods
Arsenic trioxide (As2O3) (CAS No. 1327-52-1) was purchased from Sigma-Aldrich Co., USA. Ascorbic acid was purchased from Merck Chemical Company, Germany. The nonionic surfactants used as vesicle-forming materials, including polysorbate 60 (Tween 60), sorbitan monostearate 60 (Span 60), and cholesterol (Chol), were purchased from Merck. All organic solvents and other chemicals were of analytical grade and obtained from Sigma-Aldrich.
3.1. Manufacturing Niosomes
In this study, niosomes were prepared according to a previous study using the “surface-active agents film hydration” method (20). Span 60, cholesterol, and Polyethylene Glycol (PEG) were weighed (based on amounts shown in Table 1) and dissolved in 20 mL of ethanol by stirring and hating at 50°C. Then, the organic solvent was removed by a rotary evaporator for approximately 20 minutes at the rotational speed of 90 rpm and temperature of 30 ± 2°C in vacuum conditions. To form a film on the inner wall of the balloon, 12 mL of phosphate buffer solution was added to the formulation and stirred for four hours in an incubator shaker at 110 rpm and 37°C. Then, the dispersion was sonicated for five minutes. Simultaneously with the niosomes of vitamin C, the blank niosomes (Table 1), which included no drug, were prepared by a similar process.
Ingredients in Various Formulations
Formula | Span 60 (mg) | Cholesterol (mg) | Polyethylene Glycol (mg) | Vitamin C (mg) |
---|---|---|---|---|
Blank niosome B1 | 224 | 112 | 40 | 0 |
Vitamin C niosome F1 | 224 | 112 | 40 | 24 |
Blank niosome B2 | 268 | 68 | 40 | 0 |
Vitamin C niosome F2 | 268 | 68 | 40 | 24 |
Blank niosome B3 | 200 | 136 | 40 | 0 |
Vitamin C niosome F3 | 200 | 136 | 40 | 24 |
3.2. Physicochemical Properties of Vesicles
To specify the optimal formula, first, we performed the dynamic light scattering (DLS) method to determine the size and zeta potential of the niosomes using a Zetasizer (Nano ZS3600, Malvern Instruments, UK). Drug loading and encapsulation efficiency were determined as described in previous studies. Briefly, the suspension of noisome was centrifuged using a Beckman Coulter, USA) in condition of (18,000 rpm, 10 min, 4°C). Unloaded vitamin C was separated from the niosomes, and the amount of vitamin C was estimated by an ultraviolet spectroscopy instrument (Shimadzu, Japan) at 214 nm in the supernatant. Finally, drug encapsulation efficiency and loading percent were calculated using the following equations (21):
3.3. Release of Vitamin C from Niosomes
The drug release was evaluated based on the method used in our previous study (20). Briefly, 1 mL of each niosome formula was added into a dialysis bag (Sigma, cut off 12,000 Dalton). Then, the bag was put in a bottle containing 100 mL of PBS (pH 7.4). Next, the bottles were incubated in an incubator shaker at 37°C and 110 rpm. Finally, 1 mL of samples was collected at the fixed time intervals, and the amount of drug released in the medium was calculated using the spectrophotometry method.
3.4. Cell Culture
The study was confirmed by the Ethics Committee of Hamadan University of Medical Sciences (IR.UMSHA.REC.1398.281). HEK-293 cells were purchased from the Iran Pasteur Institute and cultured in DMEM media containing 10% fetal bovine serum (FBS) and 1% penicillin-streptomycin (Pen/Strep). Cells were grown in a 37°C incubator in 5% CO2. The following steps of the experiments were performed after two to three passages. To evaluate whether the niosomes of vitamin C were more effective than vitamin C in improving As-induced toxicity, we divided cells into six groups: Cells poisoned with As, cells treated with vitamin C, cells treated with nano-vitamin C, cells treated with As + vitamin C, cells treated with As + nano-vitamin C, and cells treated with media as the control. The MTT test evaluated the toxicity and the 50% inhibitory concentration (IC50) of As, vitamin C, and nano-vitamin C.
3.5. Toxicity Assessment Using MTT Assay
Briefly, HEK-293 cells were cultured in 96-well plates containing the medium (8,000 - 6,000 cells per well). After 24 to 48 hours, the cell density was about 70% to 75%. Cells were exposed to As (1 picomolar to 1 M), vitamin C, and nano-vitamin C (1 picomolar up to 6,400 µM), and finally As (1 pM to 1 M), simultaneously treated with vitamin C and nano-vitamin C (1 picomolar to 6,400 µM) separately. One group of untreated cells was used as the control group. After the treatment, 20 μL of fresh media was added to each well and incubated for four hours at 37°C. Then, cells were drained, and 100 μL of Dimethyl Sulfoxide (DMSO) reagent was added to each well. The absorption of solutions was recorded with a Plate Reader at 570 nm. The number of viable cells in the control and other groups was calculated using the formula: O.D. treated cells/O.D. control × 100.
3.6. Oxidative Stress Biomarkers Assay
3.6.1. Measurement of MDA
The Yagi method measured lipid peroxidation. Briefly, 50 µL of the sample, 4 mL of distilled water, and 1 mL of reagent were added to the protein deposition. The reaction mixture was placed in a 95°C bath for 60 min. Then, the tubes were cooled with water, and 5 mL of butanol was added and shaken vigorously. The butanol layer was removed after centrifuging at 3,000 rpm. Finally, fluorescence intensity was measured with fluorimetry at a provocative wavelength of 515 nm and a diffusion wavelength of 553 nm (22).
3.6.2. Determination of Reactive Oxygen Species (ROS)
In this step, the treated cells spilled in a 96-well plate containing 10 μmol/L CM-H2DCFDA (Molecular Probes-Life Technology, Brooklyn, NY) were placed in an incubator at 37°C for 45 min. Then, the formation of intracellular ROS was determined using a Fluorescent Plate Reader at excitation/emission wavelengths of 485/530 nm (8).
3.6.3. Determination of CAT and SOD Activity
The activities of catalase (CAT) and superoxide dismutase (SOD) were measured using Kiazist kits KSOD91 and KCAT93, respectively, according to previous studies (23, 24). In this test, the CAT activity unit was estimated as the amount of the sample that will catalyze the decomposition of 1 micromole of H2O2 to H2O and O2 in one minute. The specific activity was reported in units/mL. The SOD activity unit was determined as the amount of sample that will catalyze the decomposition of 1 mmol of O2 to H2O2 and O2 in one minute. The activity of SOD was evaluated calorimetrically at a wavelength of 420 nm.
3.6.4. Determination of Total Antioxidant Capacity (TAC)
The TAC level of cells was determined based on the FRAP method. This method evaluated the capacity of the sample to reduce Fe3+ to Fe2+ in the presence of TPTZ (Tripyridyl-s-triazine). The interaction between Fe2+ and TPTZ produces the blue color complex. Maximum optical density was measured at 593 nm based on a calibration curve obtained by various concentrations of FeSO4 (25).
3.7. Statistical Analysis
The Graph Pad Prism software (version 6.0) was used for data analysis. The obtained data were expressed as mean ± SEM. The one-way Analysis of Variance (ANOVA), followed by Tukey’s Post Hoc test, evaluated the mean differences between experimental groups. The P values below 0.05 were considered statistically significant.
4. Results
4.1. Characteristics of Vitamin C Niosomes
Table 2 presents the physicochemical properties of niosomes in different formulations. According to the findings, although there was no significant difference in the particle size between various formulations, the F1 formulation was the optimum formulation because of its lower poly disparity index, zeta potential, and particle size. The microscopic images confirmed the information obtained by the particle size analyzer regarding the F1 formulation. The morphology of the niosome is displayed in Figure 1. Based on the results, the nano-vitamin C release pattern into the phosphate buffer (pH = 7.4) begins with a half an hour lag phase (Figure 2). This is probably due to the excellent loading efficiency of the drug in this formulation and the minimal free drug in the dialysis membrane at the first stages of drug release presses. It takes about half an hour for the drug to diffuse out of the niosome membrane. After that, the drug is released from the particles at a highly constant rate for up to two hours.
Entrapment Efficiency, Drug Loading, and Polydispersity Index of Different Samples
Formula | F1 b | F2 | F3 | B1 c | B2 | B3 |
---|---|---|---|---|---|---|
EE % | 94.76 ± 6.02 | 50.11 ± 5.4 | 33.30 ± 3.5 | NA | NA | NA |
DL % | 1.97 ± 0.02 | 1.24 ± 0.03 | 1.02 ± 0.01 | NA | NA | NA |
Particle size (nm) | 163.2 ± 6.1 | 178.6 ± 8.5 | 174.1 ± 7.1 | 127.7 ± 8.3 | 149.4 ± 5.8 | 154.3 ± 6.8 |
Zeta-potential (mV) | -23.3 ± 3.5 | -17.5 ± 3.2 | -20.3 ± 5.4 | -25.7 ± 2.5 | -24.5 ± 2.3 | -18.5 ± 3.56 |
PDI | 0.23 ± 0.011 | 0.26 ± 0.012 | 0.35 ± 0.014 | 0.32 ± 0.034 | 0.31 ± 0.022 | 0.34 ± 0.026 |
Scanning electron microscopy (SEM) image of vitamin C-loaded niosome with scale bar 1 μm
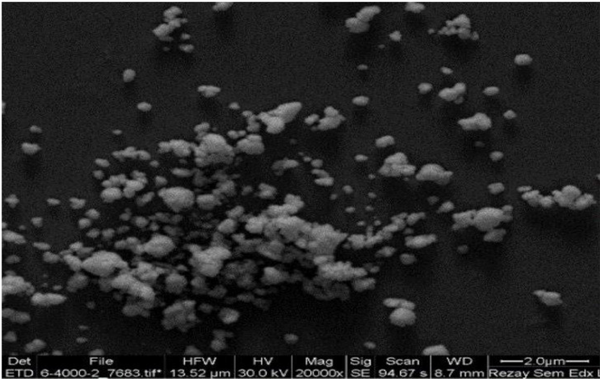
The release profile of the optimum vitamin C-loaded nanoparticles in phosphate buffer, pH = 7.4 (n = 3)
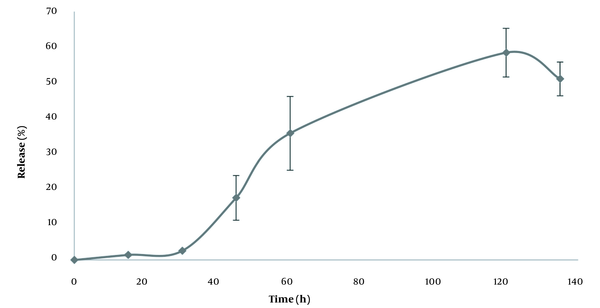
4.2. Cytotoxicity
The MTT assay was used to study the cytotoxicity effect of As, vitamin C, and the niosome of vitamin C on the HEK-293 cell line. The results showed significant reductions in cell viability at different concentrations of As, vitamin C, and nano-vitamin C compared to the control group (P < 0.05), although these changes were dose-dependent. In addition, the IC50 values of As, vitamin C, and nano-vitamin C were 2.0, 650, and 950 μM after 48 hours of cell treatment, respectively (Figures 3 and 4).
HEK-293 cell viability at different concentrations of As using MTT assay. The results were reported as the mean percentage of cell viability compared to the control group (n = 3) (* compared to control group P < 0.5; ** Compared to control group < 0.01)
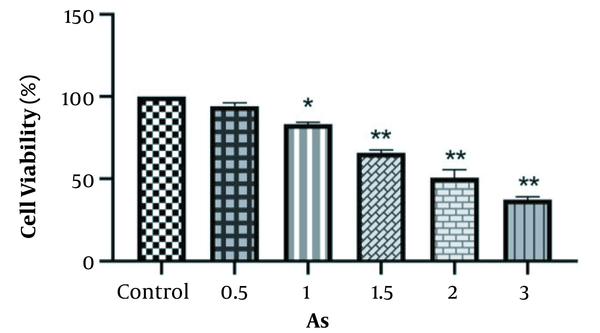
HEK-293 cell viability at different concentrations of vitamin C and nano-vitamin C. The results were reported as the mean percentage of cell viability compared to the control group (n = 3) (* compared to control group P < 0.5; ** compared to control group < 0.01).
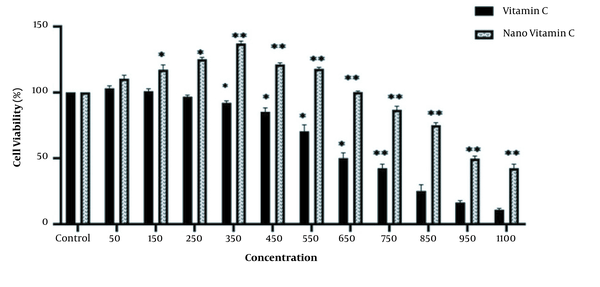
4.3. Effect of Vitamin C and Vitamin C Niosome on Cell Survival
Cell survival was significantly lower in the As-treated group than in the control group (P < 0.001, Figure 5). Treatment with vitamin C improved cell survival. However, cell survival was significantly higher in the group treated with As and vitamin C niosome (P < 0.05).
Cell viability in different groups (C, Control; As, Arsenic; C + Vit C, Control + Vitamin C; C + Nvit C, Control + nan0-vitamin C; As + Vit C, Arsenic + Vitamin C; As + Nvit C, Arsenic + nano-vitamin C). The data were reported based on Mean ± SD. It should be noted that ANOVA and Tukey test were used to compare the data (* Compared to control group P < 0.001, ** Compared to control group < 0.05, # Compared to As group P < 0.05).

4.4. MDA and ROS Levels
Oxidative biomarkers such as MDA and ROS were evaluated to study the effect of vitamin C and vitamin C niosome on As-induced toxicity in HEK-293 cells. Based on the results, As caused a significant increase in MDA and ROS levels in HEK-293 cells compared to the control group (P < 0.01). Conversely, vitamin C and vitamin C niosome treatment reduced MDA and ROS levels compared to the As group. Accordingly, the vitamin C niosome was more effective than vitamin C on the MDA and ROS of cells (Figure 6A and B).
Effect of vitamin C and nano-vitamin C on A, MDA; B, ROS; C, SOD; D, TAC; and E, CAT levels in HEK-293 cell; (C, Control; As, Arsenic; C + Vit C, Control + Vitamin C; C + Nvit C, Control + nanovitamin C; As+Vit C, Arsenic + Vitamin C; As + Nvit C, Arsenic + nano-vitamin C). The data were reported based on mean ± SD. It should be noted that ANOVA and Tukey test were used to compare the data (* Compared to control group P < 0.001; ** Compared to control group < 0.05; # Compared to As group P < 0.05).
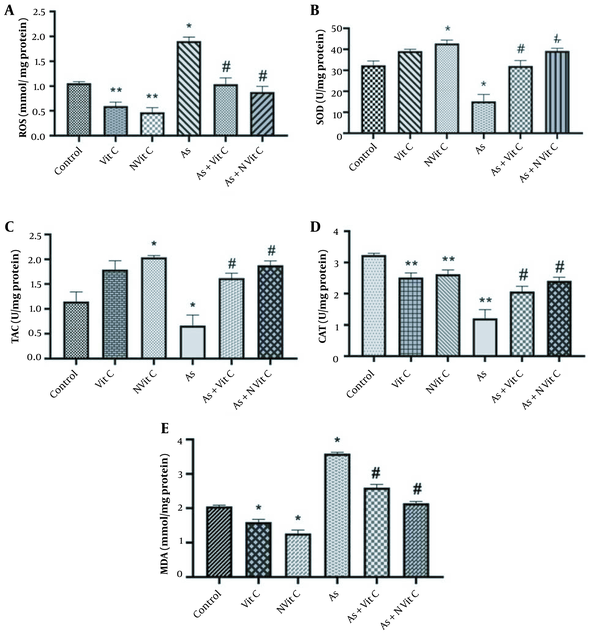
4.5. The activity of SOD, CAT, and TAC
The activities of antioxidant enzymes, SOD, CAT, and TAC, were measured in HEK-293 cells. As shown in Figure 6C - E, SOD, TAC, and CAT activities were significantly lower in the As group than in the control group. However, treatment with vitamin C and vitamin C niosome could improve the alterations of SOD, TAC, and CAT activities in HEK-293 cells. These changes were more significant in the vitamin C niosome group than in the vitamin C group.
5. Discussion
This study evaluated whether vitamin C-loaded niosome has protective effects over vitamin C against As-induced toxicity in HEK-293 cells. For this purpose, a stable formulation of niosome vitamin C was prepared, which effectively reduced the As toxicity compared to vitamin C in HEK-293 cells. Vitamin C or L-ascorbic acid is a hydrophilic vitamin that acts as a free radical scavenger, regulates cholesterol metabolism, and also inhibitor of the radical chain of LDL oxidation in cells (26). Although vitamin C is an effective natural antioxidant, it is eliminated from cells shortly after consumption because of poor stability and rapid degradation, especially in oxidative pathways (19). Therefore, to overcome this problem and increase the effectiveness of this antioxidant, a new drug delivery system as niosomes was designed and evaluated to reduce As toxicity. Niosomes can encapsulate lipophilic and hydrophilic drugs within their structures, thus protecting drugs from external agents, increasing drug absorption and effectiveness, and reducing the toxicity of drugs (27, 28). The structure of the niosome does not have enough charges because of non-ionic surfactants; therefore, the stabilization of niosomes can be obtained by their neutral charges at the surface, hydrophilic nature, and the flexibility of polyethylene glycol chains, which can prevent their interactions with biological ingredients (29).
Different niosomes of vitamin C were designed and studied at the beginning of our study (Table 1). Eventually, the niosome of vitamin C based on F1 formulation was used for in vitro study due to properties such as more possibility of cellular uptake, higher drug loading, and narrower particle size distribution than other formulations. To investigate the performance of the select niosome, we studied various parameters and compared them with those of the free drug, including zeta potential, particle size, loading rate, and entrapment efficiency percentage. Many studies have investigated the effect of zeta potential and the size of nanoparticles, indicating that nanoparticles with a smaller size could easily enter the cell and cause better efficiency for removing toxic radicals due to their absorption and accumulation in the target cell (30). On the other hand, the zeta potential of nanoparticles has a vital role in the electrical constancy, along with their effect on cellular uptake, so that nanoparticles with higher electrical stability can prevent the niosomes aggregation and enlargement over time (31). In this study, both mentioned parameters were examined for the select vitamin C niosome. The best particle size and zeta potential of the niosome were 163.2 ± 6.1 nm and 23.3 ± 3.5 mV, respectively. Our findings are in line with previous studies, indicating that the increased absolute value of zeta potential of nanoparticles up-regulates the cellular uptake (32).
Drug loading was another effective parameter evaluated for the vitamin C niosome. The designed niosome, in addition to high stability, needs a high load capacity in the body to maintain its properties and increase the absorption and activity of the drug for removing xenobiotics and toxins in the target tissue (33, 34). In this study, many attempts were made for optimizing the percentage of cholesterol to the surfactant to obtain the highest drug loading so that the percentage of drug loading in the optimal formulation was 1.97. In line with our study, Varshosaz et al. reported that the percentage of loading efficiency was lower for α-tocopherol molecules than for ascorbic acid because of its hydrophobicity (19). After selecting the best formulation for making vitamin C niosome, our study investigated the protective effect of vitamin C niosome compared to vitamin C against As-induced OS in the HEK-293 cell line. According to our results (Figure 6A and B), the MDA and ROS levels were significantly higher in cells treated with As than in the control group.
Meanwhile, As reduced CAT, SOD, and TAC activities in these cells. Arsenic can produce various ROS such as superoxide radicals (O2), hydrogen peroxide (H2O2), nitric oxide, dimethyl As proxy radicals, dimethyl As radicals, and perhydroxyl radicals in cells (35). The free radicals can react with most biomolecules such as DNA, lipids, and proteins and damage them (36). According to previous research (37), As via OS can consume and deplete antioxidant systems (enzymatic and non-enzymatic). Arsenic can attenuate antioxidant systems such as CAT and TAC, which are the most significant antioxidant enzymes against the reduced forms of oxygen, thereby conquering OS. These enzymes convert toxic oxygen species into less injurious or inoffensive products (38, 39). Several research studies approved the role of OS in As toxicity. For example, Ting et al. expressed that ROS has an essential role in mediating cellular damage induced by As through the induction of OS and diminishment of antioxidant systems (37). Consistent with our results, Karasavvas et al. concluded that As can produce high levels of H2O2 and other ROSs in HL60 and U266 cells (40). In another in vivo study, Roy et al. showed that As induced genotoxicity and OS (26). According to some previous studies, As can induce toxic effects by OS-induced mechanisms, and various antioxidants could ameliorate the toxic effects in HEK-293 cells (1, 41).
The current study used vitamin C and vitamin C niosome against As-induced OS. The obtained data confirmed that vitamin C and vitamin C niosome decreased oxidative cell markers (i.e., MDA and TOS) while improving antioxidant systems (i.e., CAT, SOD, and TAC) compared to the As group. It should be noted that these effects were more significant in cells receiving the vitamin C niosome. This effect can be related to its antioxidant properties (42). Based on the evidence, vitamin C neutralizes the generated free radicals inside and outside the cells to combat cell damage. Free radicals tend to connect electrons to regain their stability, and vitamin C is a rich source of electrons. Therefore, it can donate electrons to various free radicals such as superoxide and hydroxyl radicals and suppress their reactivity (43). El-Gendy et al. (11) revealed that vitamin C significantly increased HEK-293 cell survival dose-dependently, in line with our results. The obtained results from another research showed that vitamin C could ameliorate imidacloprid-induced oxidative damage by reducing the MDA level and altering antioxidant defense activity in animals (11). Likewise, Vineetha et al. investigated the therapeutic effects of vitamin C and α-tocopherol on As-induced toxicity in H9c2 cardiomyocyte cells by activating Bcl2 and Nrf2 transcription factors. They found that vitamin C and α-tocopherol alleviated As-induced OS in cardiac cells by increasing cell survival and preventing apoptosis (44).
Similarly, Majumdar et al. reported that the co-administration of As with folic acid or vitamin B12 could reduce oxidative damage such as MDA and OH levels and thus restore SOD and CAT activities (45). Based on the findings of another study, vitamin E could reduce the risk of As-related urothelial carcinoma (46). Chung et al. also indicated that vitamin C could improve mitochondrial functions in the cells of As-exposed rats (46). Based on biochemical tests, the vitamin C niosome had a better performance in improving redox status than vitamin C, and also it was obtain that changes between the forms of niosome drug and the free form of niosome drug were significant. Consistent with our study, other studies demonstrated that drug formulation in a novel form increases absorption and stability and doubles the effectiveness (20, 47). Therefore, our proposed formulation improved AS-induced OS by increasing SOD, CAT, and TAC levels and reducing ROS, and could be considered a supportive treatment for As toxicity.
5.1. Conclusion
The vitamin C niosome was more effective than vitamin C in treating As-induced toxicity. It should be mentioned that, extensive studies along with more techniques, can be beneficial to increase our knowledge regarding nanoparticles and their use in the treatment of high toxicity induced by different toxicants.
Acknowledgements
References
-
1.
Chang SI, Jin B, Youn P, Park C, Park JD, Ryu DY. Arsenic-induced toxicity and the protective role of ascorbic acid in mouse testis. Toxicol Appl Pharmacol. 2007;218(2):196-203. [PubMed ID: 17188728]. https://doi.org/10.1016/j.taap.2006.11.009.
-
2.
Zeng L. A method for preparing silica-containing iron(III) oxide adsorbents for arsenic removal. Water Res. 2003;37(18):4351-8. [PubMed ID: 14511705]. https://doi.org/10.1016/s0043-1354(03)00402-0.
-
3.
Valko M, Leibfritz D, Moncol J, Cronin MT, Mazur M, Telser J. Free radicals and antioxidants in normal physiological functions and human disease. Int J Biochem Cell Biol. 2007;39(1):44-84. [PubMed ID: 16978905]. https://doi.org/10.1016/j.biocel.2006.07.001.
-
4.
Zhang S, Niu H, Cai Y, Zhao X, Shi Y. Arsenite and arsenate adsorption on coprecipitated bimetal oxide magnetic nanomaterials: MnFe2O4 and CoFe2O4. Chem Eng J. 2010;158(3):599-607. https://doi.org/10.1016/j.cej.2010.02.013.
-
5.
Jarup L. Hazards of heavy metal contamination. Br Med Bull. 2003;68:167-82. [PubMed ID: 14757716]. https://doi.org/10.1093/bmb/ldg032.
-
6.
Chiou HY, Chiou ST, Hsu YH, Chou YL, Tseng CH, Wei ML, et al. Incidence of transitional cell carcinoma and arsenic in drinking water: a follow-up study of 8,102 residents in an arseniasis-endemic area in northeastern Taiwan. Am J Epidemiol. 2001;153(5):411-8. [PubMed ID: 11226969]. https://doi.org/10.1093/aje/153.5.411.
-
7.
Steinmaus C, Moore L, Hopenhayn-Rich C, Biggs ML, Smith AH. Arsenic in drinking water and bladder cancer. Cancer Invest. 2000;18(2):174-82. [PubMed ID: 10705880]. https://doi.org/10.3109/07357900009038249.
-
8.
D'Apolito M, Du X, Pisanelli D, Pettoello-Mantovani M, Campanozzi A, Giacco F, et al. Urea-induced ROS cause endothelial dysfunction in chronic renal failure. Atherosclerosis. 2015;239(2):393-400. [PubMed ID: 25682038]. [PubMed Central ID: PMC4361277]. https://doi.org/10.1016/j.atherosclerosis.2015.01.034.
-
9.
Jomova K, Jenisova Z, Feszterova M, Baros S, Liska J, Hudecova D, et al. Arsenic: toxicity, oxidative stress and human disease. J Appl Toxicol. 2011;31(2):95-107. [PubMed ID: 21321970]. https://doi.org/10.1002/jat.1649.
-
10.
Zargari F, Tabaghchi Saeedy H. Effects of Vitamin C on Paraoxonase1 Arylesterase Activity in Rats Exposed to Arsenic. Iran J Toxicol. 2017;11(3):47-50. https://doi.org/10.29252/arakmu.11.3.47.
-
11.
El-Gendy KS, Aly NM, Mahmoud FH, Kenawy A, El-Sebae AK. The role of vitamin C as antioxidant in protection of oxidative stress induced by imidacloprid. Food Chem Toxicol. 2010;48(1):215-21. [PubMed ID: 19833166]. https://doi.org/10.1016/j.fct.2009.10.003.
-
12.
Rietjens IM, Boersma MG, Haan L, Spenkelink B, Awad HM, Cnubben NH, et al. The pro-oxidant chemistry of the natural antioxidants vitamin C, vitamin E, carotenoids and flavonoids. Environ Toxicol Pharmacol. 2002;11(3-4):321-33. [PubMed ID: 21782615]. https://doi.org/10.1016/s1382-6689(02)00003-0.
-
13.
Pal S, Chatterjee AK. Prospective protective role of melatonin against arsenic-induced metabolic toxicity in Wistar rats. Toxicology. 2005;208(1):25-33. [PubMed ID: 15664430]. https://doi.org/10.1016/j.tox.2004.11.005.
-
14.
Martinez VD, Vucic EA, Becker-Santos DD, Gil L, Lam WL. Arsenic exposure and the induction of human cancers. J Toxicol. 2011;2011:431287. [PubMed ID: 22174709]. [PubMed Central ID: PMC3235889]. https://doi.org/10.1155/2011/431287.
-
15.
Ramanathan K, Shila S, Kumaran S, Panneerselvam C. Protective role of ascorbic acid and alpha-tocopherol on arsenic-induced microsomal dysfunctions. Hum Exp Toxicol. 2003;22(3):129-36. [PubMed ID: 12723893]. https://doi.org/10.1191/0960327103ht329oa.
-
16.
Banerjee P, Bhattacharyya SS, Bhattacharjee N, Pathak S, Boujedaini N, Belon P, et al. Ascorbic acid combats arsenic-induced oxidative stress in mice liver. Ecotoxicol Environ Saf. 2009;72(2):639-49. [PubMed ID: 18715643]. https://doi.org/10.1016/j.ecoenv.2008.07.005.
-
17.
Kadirvel R, Sundaram K, Mani S, Samuel S, Elango N, Panneerselvam C. Supplementation of ascorbic acid and alpha-tocopherol prevents arsenic-induced protein oxidation and DNA damage induced by arsenic in rats. Hum Exp Toxicol. 2007;26(12):939-46. [PubMed ID: 18375637]. https://doi.org/10.1177/0960327107087909.
-
18.
Apridamayanti P, Listiyana N, Desnita R. Formulation vitamin C using niosomes system span 80 in gel for increase stability and penetration in vitro. Int J PharmTech Res. 2016;9(2):81-8.
-
19.
Varshosaz J, Taymouri S, Pardakhty A, Asadi-Shekaari M, Babaee A. Niosomes of ascorbic acid and alpha-tocopherol in the cerebral ischemia-reperfusion model in male rats. Biomed Res Int. 2014;2014:816103. [PubMed ID: 25309927]. [PubMed Central ID: PMC4163366]. https://doi.org/10.1155/2014/816103.
-
20.
Firouzian F, Pourshoja P, Nili-Ahmadabadi A, Ranjbar A. Hepatoprotective effect of N-acetylcystein loaded niosomes on liver function in paraquat-induced acute poisoning. Pestic Biochem Physiol. 2019;160:146-53. [PubMed ID: 31519249]. https://doi.org/10.1016/j.pestbp.2019.08.001.
-
21.
Mitsopoulos P, Omri A, Alipour M, Vermeulen N, Smith MG, Suntres ZE. Effectiveness of liposomal-N-acetylcysteine against LPS-induced lung injuries in rodents. Int J Pharm. 2008;363(1-2):106-11. [PubMed ID: 18694812]. https://doi.org/10.1016/j.ijpharm.2008.07.015.
-
22.
Yagi K. Simple assay for the level of total lipid peroxides in serum or plasma. Methods Mol Biol. 1998;108:101-6. [PubMed ID: 9921519]. https://doi.org/10.1385/0-89603-472-0:101.
-
23.
Karkhanei B, Talebi Ghane E, Mehri F. Evaluation of oxidative stress level: total antioxidant capacity, total oxidant status and glutathione activity in patients with COVID-19. New Microbes New Infect. 2021;42:100897. [PubMed ID: 34026228]. [PubMed Central ID: PMC8127525]. https://doi.org/10.1016/j.nmni.2021.100897.
-
24.
Mehri F, Rahbar AH, Ghane ET, Souri B, Esfahani M. Changes in oxidative markers in COVID-19 patients. Arch Med Res. 2021;52(8):843-9. [PubMed ID: 34154831]. [PubMed Central ID: PMC8180845]. https://doi.org/10.1016/j.arcmed.2021.06.004.
-
25.
Benzie IF, Strain JJ. Ferric reducing/antioxidant power assay: direct measure of total antioxidant activity of biological fluids and modified version for simultaneous measurement of total antioxidant power and ascorbic acid concentration. Methods Enzymol. 1999;299:15-27. [PubMed ID: 9916193]. https://doi.org/10.1016/s0076-6879(99)99005-5.
-
26.
Roy P, Mukherjee A, Giri S. Positive effects of Vitamin C in arsenic trioxide and sodium fluoride induced genotoxicity and oxidative stress in mice in vivo. Pollution. 2015;1(4):451-60.
-
27.
Pardakhty A, Moazeni E. Nano-niosomes in drug, vaccine and gene delivery: a rapid overview. Nanomed J. 2013;1(1):1-12.
-
28.
Sunilkumar M. Niosomes As novel drug delivery system. Int Res J Pharm Appl Sci. 2015;5:1-7.
-
29.
Jain NK, Nahar M. PEGylated nanocarriers for systemic delivery. Methods Mol Biol. 2010;624:221-34. [PubMed ID: 20217599]. https://doi.org/10.1007/978-1-60761-609-2_15.
-
30.
Hofto LR. Molecular Modeling of the Metabolism of Acetaminophen and [thesis]. Tennessee, USA: Rhodes College; 2010.
-
31.
Ag Seleci D, Seleci M, Walter J, Stahl F, Scheper T. Niosomes as Nanoparticular Drug Carriers: Fundamentals and Recent Applications. J Nanomater. 2016;2016:1-13. https://doi.org/10.1155/2016/7372306.
-
32.
Honary S, Zahir F. Effect of Zeta Potential on the Properties of Nano-Drug Delivery Systems - A Review (Part 2). Trop J Pharm Res. 2013;12(2). https://doi.org/10.4314/tjpr.v12i2.20.
-
33.
Manconi M, Sinico C, Valenti D, Loy G, Fadda AM. Niosomes as carriers for tretinoin. I. Preparation and properties. Int J Pharm. 2002;234(1-2):237-48. [PubMed ID: 11839454]. https://doi.org/10.1016/s0378-5173(01)00971-1.
-
34.
Zhao MX, Zhu BJ. The Research and Applications of Quantum Dots as Nano-Carriers for Targeted Drug Delivery and Cancer Therapy. Nanoscale Res Lett. 2016;11(1):207. [PubMed ID: 27090658]. [PubMed Central ID: PMC4835414]. https://doi.org/10.1186/s11671-016-1394-9.
-
35.
Valko M, Rhodes CJ, Moncol J, Izakovic M, Mazur M. Free radicals, metals and antioxidants in oxidative stress-induced cancer. Chem Biol Interact. 2006;160(1):1-40. [PubMed ID: 16430879]. https://doi.org/10.1016/j.cbi.2005.12.009.
-
36.
Reuter S, Gupta SC, Chaturvedi MM, Aggarwal BB. Oxidative stress, inflammation, and cancer: how are they linked? Free Radic Biol Med. 2010;49(11):1603-16. [PubMed ID: 20840865]. [PubMed Central ID: PMC2990475]. https://doi.org/10.1016/j.freeradbiomed.2010.09.006.
-
37.
Ting CM, Lee YM, Wong CK, Wong AS, Lung HL, Lung ML, et al. 2-Methoxyestradiol induces endoreduplication through the induction of mitochondrial oxidative stress and the activation of MAPK signaling pathways. Biochem Pharmacol. 2010;79(6):825-41. [PubMed ID: 19883629]. https://doi.org/10.1016/j.bcp.2009.10.018.
-
38.
Yu H, Liu S, Li M, Wu B. Influence of diet, vitamin, tea, trace elements and exogenous antioxidants on arsenic metabolism and toxicity. Environ Geochem Health. 2016;38(2):339-51. [PubMed ID: 26169729]. https://doi.org/10.1007/s10653-015-9742-8.
-
39.
Rana SV; Sohini. Protective effect of ascorbic acid against oxidative stress induced by inorganic arsenic in liver and kidney of rat. Indian J Exp Biol. 2007;45(4):371-5. [PubMed ID: 17477310].
-
40.
Karasavvas N, Carcamo JM, Stratis G, Golde DW. Vitamin C protects HL60 and U266 cells from arsenic toxicity. Blood. 2005;105(10):4004-12. [PubMed ID: 15677571]. [PubMed Central ID: PMC1895087]. https://doi.org/10.1182/blood-2003-03-0772.
-
41.
Singh AP, Goel RK, Kaur T. Mechanisms pertaining to arsenic toxicity. Toxicol Int. 2011;18(2):87-93. [PubMed ID: 21976811]. [PubMed Central ID: PMC3183630]. https://doi.org/10.4103/0971-6580.84258.
-
42.
Lutsenko EA, Carcamo JM, Golde DW. Vitamin C prevents DNA mutation induced by oxidative stress. J Biol Chem. 2002;277(19):16895-9. [PubMed ID: 11884413]. https://doi.org/10.1074/jbc.M201151200.
-
43.
Bindhumol V, Chitra KC, Mathur PP. Bisphenol A induces reactive oxygen species generation in the liver of male rats. Toxicology. 2003;188(2-3):117-24. [PubMed ID: 12767684]. https://doi.org/10.1016/s0300-483x(03)00056-8.
-
44.
Vineetha RC, Binu P, Arathi P, Nair RH. L-ascorbic acid and alpha-tocopherol attenuate arsenic trioxide-induced toxicity in H9c2 cardiomyocytes by the activation of Nrf2 and Bcl2 transcription factors. Toxicol Mech Methods. 2018;28(5):353-60. [PubMed ID: 29297235]. https://doi.org/10.1080/15376516.2017.1422578.
-
45.
Majumdar S, Maiti A, Karmakar S, Das AS, Mukherjee S, Das D, et al. Antiapoptotic efficacy of folic acid and vitamin B(1)(2) against arsenic-induced toxicity. Environ Toxicol. 2012;27(6):351-63. [PubMed ID: 22566245]. https://doi.org/10.1002/tox.20648.
-
46.
Chung CJ, Pu YS, Chen YT, Su CT, Wu CC, Shiue HS, et al. Protective effects of plasma alpha-tocopherols on the risk of inorganic arsenic-related urothelial carcinoma. Sci Total Environ. 2011;409(6):1039-45. [PubMed ID: 21227482]. https://doi.org/10.1016/j.scitotenv.2010.11.037.
-
47.
Firozian F, Karami S, Ranjbar A, Azandaryani MT, Nili-Ahmadabadi A. Improvement of therapeutic potential N-acetylcysteine in acetaminophen hepatotoxicity by encapsulation in PEGylated nano-niosomes. Life Sci. 2020;255:117832. [PubMed ID: 32450164]. https://doi.org/10.1016/j.lfs.2020.117832.