Abstract
Keywords
1-Hexadecyl 3-Methylimidazolium Chloride Pharmaceutical Triton X-114 Visible Spectroscopy Vitamin B12
1. Background
Cobalt is an essential element required for the human body in minute amounts as a component of vitamin B12 (1-3). Vitamin B12 (cobalamin) plays a vital role in producing red blood cells and DNA. In addition, the proper functioning of the nervous system depends on this compound. Some investigations have shown a relationship between maternal B12 deficiency and the risk of a defect in the fetal neural tube (4). On the other hand, excessive cobalt uptake can lead to diseases such as asthma, vasodilation, flushing, and cardiomyopathy (5-7). The recommended daily intake of vitamin B12 is about 2.4 μg.
This work proposes a simple surface-active ionic liquid-based preconcentration method for the spectrophotometric determination of cobalt ions in vitamin B12 samples. Ionic Liquids (ILs) are a new class of ionic compounds with low melting points and significant properties such as good chemical and thermal stability, tunable viscosity, miscibility with water and organic solvents, and extremely low vapor pressure (8). These fascinating features introduce them as attractive substitutes for the extracting phase in liquid-liquid microextraction methods.
Commonly, hydrophobic ILs are chosen for this purpose. The advantage of this property (hydrophobicity) becomes apparent in the final stage of the extraction process when the extraction phase must be separated from the aqueous phase. Because of the negligible interaction tendency between the hydrophobic IL phase and aqueous phase, the separation step can be implemented easily. Though these kinds of ILs are favorable in liquid-liquid microextraction procedures, they suffer from the lack of access to the highest attainable extraction efficiency. This malfunction is due to the low contact area between the IL micro-volume phase and the analyte-containing phase. Thus, various methods are imperative to disperse the IL phase homogeneously in the extraction medium (9-20).
Recently we reported a modified extraction procedure based on the surface activity of some ILs. We showed that Surface-active ILs (SAILs) could provide efficient extraction without a dispersing agent (21). Indeed, under optimum conditions, SAILs make a homogeneous, evenly dispersed extracting phase with high contact area so that an efficient extraction would be possible. On the other hand, the SAILs water solubility encounters the analyst with another problem: Separating the analyte-rich extraction phase from the aqueous phase at the final stage of the extraction process. As discussed elsewhere (21), it is possible to overcome this problem by combining the SAIL-based extraction procedure with the cloud point extraction method. Then, we can separate phases easily just by maintaining the temperature of the extraction medium higher than the cloud point temperature (CPT) of the SAIL-based mixed micellar system. Thus, the proposed SAIL-assisted cloud point extraction (CPE) method would enable us to have the benefits of both SAILs and the CPE method as a simple way of overcoming the difficulty of phase separation.
In this study, the mixture of a surface-active ionic liquid (C16MeImCl; CMC: 0.97 × 10-3 mol L-1) and a nonionic surfactant with low CPT (Triton-X114) was used as the extraction phase for implementing the SAIL-assisted CPE method. Our preliminary experiments showed that the CPT of this mixed micellar system is about 29°C at the optimized experimental conditions; thus, the separation of phases would be possible by setting the temperature of the extraction mixture above this value. It is noteworthy that in the absence of Triton X-114, the whole mixture remains clear, and no phase separation would occur even by raising the temperature to around 90°C.
Due to its nutritional and toxicological importance, cobalt is necessary to assay in real samples, such as pharmaceutics, food products, biological fluids, and natural waters (22-24). Several instrumental techniques have been developed to determine the trace amounts of cobalt, such as flame atomic absorption spectrometry (25-30), inductively coupled plasma mass spectrometry (31), inductively coupled plasma optical emission spectrometry (32), high-performance liquid chromatography (33), and graphite furnace atomic absorption spectrometry (34-36). However, these techniques are sophisticated and expensive. Thus, the explained preconcentration procedure combined with visible spectrophotometry is proposed as a simple, sensitive, and economical substitute for determining cobalt ions in real pharmaceutical samples. To show the influence of the SAIL on the extraction efficiency, we compared the analytical features of the proposed method to those obtained in the absence of the SAIL.
2. Methods
2.1. Materials
C16MeImCl and ammonium pyrrolidinedithiocarbamate (APDC) were purchased from Acros Organics (Geel, Belgium) and Merck (Darmstadt, Germany), respectively. The nonionic surfactant Triton X-114 (Sigma, St. Loius, MO, USA) was used without further purification. A 1.0% (v/v) Triton X-114 stock solution was prepared by dissolving 1.0 mL of Triton X- 114 in distilled water in a 100 mL volumetric flask. A cobalt stock solution was prepared by dissolving appropriate amounts of cobalt nitrate (Sigma-Aldrich, St. Louis, MO, USA) in double-distilled water. Britton-Robinson buffer solutions were used to adjust the pH of the solutions. Vitamin B12 ampoules (Caspian Pharmaceutical Company) and tablets (Health Aid) were purchased from a local drug store.
2.2. Apparatus
Absorption spectra were obtained using a SPECORD 250 UV-Visible spectrophotometer (Analytikjena). A pH meter model 827 Metrohm (Herisau, Switzerland) was used to measure and adjust the pH of the solutions. A Julabo thermostated water bath (Seelbach, Germany) model GMBH D-77960 was used to control the temperature. A Hettich Universal 320R centrifuge (Tuttlingen, Germany) was used to facilitate the separation of the extraction phase.
2.3. SAIL-Assisted CPE Procedure
Ten milliliters of cobalt solution (sample or standard) was transferred into a conical-bottom Teflon test tube. Then, 0.3 mL of 0.01 M C16MeImCl solution, 0.9 mL Triton- X114 (1%), and 0.055 mL of 2 × 10-2 mol L-1 APDC solution were added. The pH of the mixture was adjusted to 6.0 by adding 1.0 mL of Britton–Robinson buffer solution. After adding 0.75 mL of 10% (w/v) NaCl to the mixture, it was placed in a water bath at 45°C for 10 min. The phase separation was performed by centrifugation at 3,000 rpm for 10 min. After removing the upper aqueous phase with a syringe, the residue was diluted by adding 0.45 mL of ethanol. The absorbance of this solution was measured against blank at 657 nm.
2.4. Assay Procedure of Cobalt Ions in Real Samples
The contents of three vitamin B12 ampoules were mixed thoroughly. The obtained solution was transferred to a 10.0 mL volumetric flask and diluted to the mark with double distilled water. Before the extraction process, it was necessary to digest the real samples by adding proper reagents such as nitric acid and hydrogen peroxide. Therefore, an appropriate amount of the diluted sample was transferred to a Pyrex beaker. After adding 2.0 mL of nitric acid (1: 1), the mixture was heated on a hot plate to near dryness. After cooling to room temperature, 0.4 mL of concentrated hydrogen peroxide (30%) was added. The resulting solution was diluted up to 10.0 mL with distilled water, and then cobalt ions were extracted and determined as described in the previous section. Alternatively, after milling and mixing three tablets, the resulting homogenized powder was transferred to a 10.0 mL volumetric flask and diluted to the mark with double distilled water. Then, the appropriate amount of the obtained solution was transferred to a Pyrex beaker and treated according to the procedure applied for B12 ampoules.
3. Results and Discussion
As discussed earlier, the proposed mixed micellar system based on C16MeImCl and Triton-X114 was used for the cloud point extraction and preconcentration of cobalt ions. To transfer the analyte ions into the micellar phase, we would convert the analyte ions to a complex hydrophobic molecule. Thus, APDC as a suitable ligand was used for the complexation of cobalt ions. Considering the absorption spectrum of the cobalt-APDC complex (Figure 1), it was possible to measure the concentration of this ion at about 650 nm (wavelength of maximum absorption of the complex).
Absorption spectrum of the cobalt-APDC complex after extraction of 10 mL of cobalt solution (2 ppm).
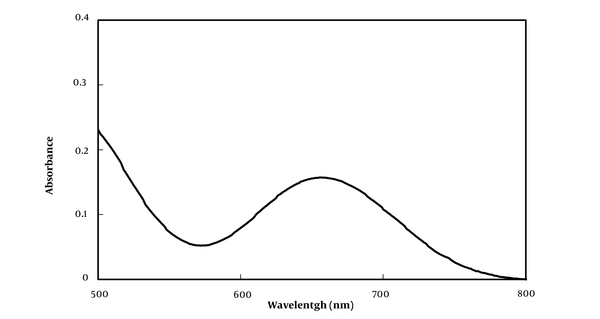
For quantitative extraction of cobalt ions by the mixed micellar system, the influences of various parameters affecting the extraction efficiency were primarily investigated using univariate optimization. In addition, a Box-Behnken design (BBD), which is a common response surface methodology (RSM) for experimental design, was used to investigate the effects of the mentioned variables and the probable interaction between them. The BBD always considers three coded levels (-1, 0, +1) for each variable. The number of experiments (N) required for the development of BBD is defined as N = 2k (k - 1) + C0, where k is the number of variables to be tested, and C0 is the number of repetitions at the central point (37). Thus, five factors, including sample pH, amount of C16MeImCl, the concentration of the chelating agent, amount of salt, and volume of Triton X-114 that could affect the extraction efficiency, were chosen according to the preliminary experiments (Table 1). Other parameters such as temperature and extraction time, centrifuging rate, and time were kept constant. According to the above-mentioned equation, for five factors and three center points, 46 experimental runs were planned. Based on the matrix design (Appendix 1), 46 experiments were performed. The procedure used for the tests was the same as explained in section 2.3, using a standard solution of 1 mg L-1 Co ions. The absorbance of the Co-APDC complex at 657 nm was used as the response. The experimental data were processed using Minitab 17 statistical software.
Factors and Levels for Box-Behnken Design
Factors | Level | ||
---|---|---|---|
-1 | 0 | +1 | |
pH | 2.5 | 6 | 9.5 |
Concentration of SAIL (mM) | 0.1 | 0.3 | 0.5 |
Volume of Triton X-114 (mL) | 0.8 | 0.9 | 1 |
Volume of APDC (mL) | 0.03 | 0.05 | 0.07 |
Concentration of NaCl (%) | 0.55 | 0.75 | 0.95 |
3.1. Univariate Optimization of Factors Affecting Extraction Performance
3.1.1. Effect of Extracting Phase Composition
First, the effect of the Triton X-114 amount on the extraction efficiency was investigated by applying various volumes of this surfactant in the range of 0.3 - 1.5 mL. As shown in Figure 2A, the maximum efficiency was observed in the presence of 0.9 mL of Triton X-114 (1.0% solution). In the second step, the effect of SAIL concentration on the extraction efficiency was investigated by adding different amounts of C16MeImCl to the extraction mixture. As Figure 2B shows, when the concentration of C16MeImCl was equal to 0.3 mM, the extraction efficiency was at its maximum value.
A, Effect of Triton X-114 concentration on extraction efficiency. Experimental conditions: Cobalt concentration, 1 mg L-1; SAIL concentration, 0.3 mM; APDC, 0.06 mL (0.02 M); pH, 6; NaCl, 0.75%; B, Effect of SAIL concentration on extraction efficiency. Experimental conditions: Cobalt concentration, 1 mg L-1; Triton X-114, 0.9 mL (0.1%); APDC, 0.06 mL (0.02 M); pH, 6; NaCl, 0.75%..
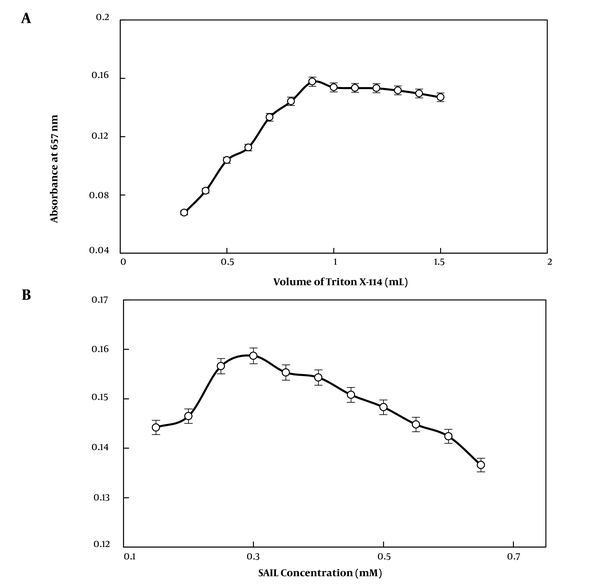
3.1.2. Effect of Sample pH
Considering the effect of the extraction mixture pH on the APDC-cobalt complexation reaction, it is necessary to find the optimized pH value. Thus, the influence of sample pH on the extraction efficiency was investigated in the range of 2 - 10. As shown in Figure 3, the extraction efficiency was increased as the sample pH increased from 3.0 to 6.0 and then decreased when the pH was higher than 6.0. Hence, the solution pH was adjusted to 6.0 for further experiments. The decrease in the extraction of cobalt ions at pH < 6 might be due to the dithiocarbamate ion protonation in acidic solutions and the subsequent decomposition into carbon disulfide and protonated amines (38). The decreased absorbance at higher pHs could be attributed to the hydrolysis of cobalt ions.
Effect of pH on extraction efficiency. Experimental conditions: Cobalt concentration, 1 mg L-1; SAIL concentration, 0.3 mM; Triton X-114, 0.9 mL (0.1%); APDC, 0.06 mL (0.02 M); NaCl, 0.75%.
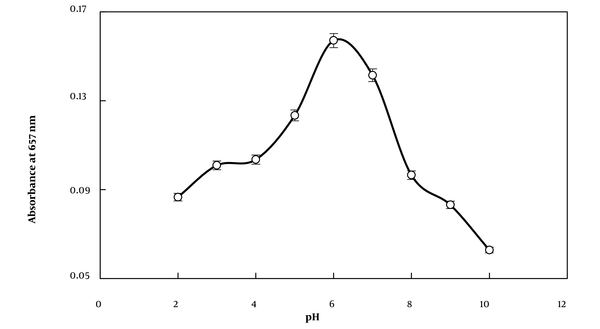
3.1.3. Effect of APDC Concentration
The hydrophobicity of the analyte ions has a vital role in their complete transfer into the extracting phase; thus, a proper chelating agent should be used to offer this property to the analyte ions. In this work, APDC was used as the chelating agent, and its effect was studied on the extraction efficiency of cobalt ions. Thus, various volumes (0.03 - 0.08 mL) of 2 × 10-2 mol L-1 APDC solution were examined. As Figure 4 shows, the maximum absorbance was observed in the presence of 0.055 mL of the APDC solution. A nearly constant variation in the absorbance is evident at higher ligand concentrations. To compensate for the consumption of APDC as a consequence of reaction with other probably available interfering ions in real samples, we used 0.06 mL of 0.02 M APDC solution in the subsequent experiments.
Effect of ligand amount on the extraction efficiency. Experimental conditions: Cobalt concentration, 1 mg L-1; SAIL concentration, 0.3 mM; Triton X-114, 0.9 mL (0.1%); pH, 6; NaCl, 0.75%.
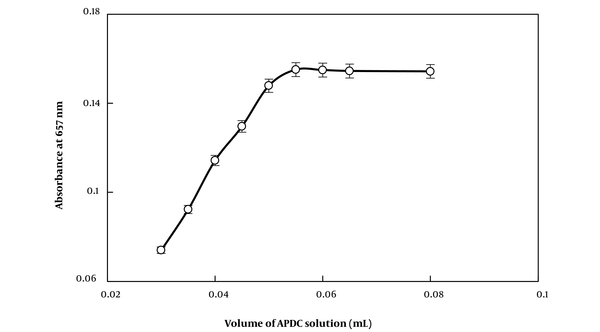
3.1.4. Effects of Equilibration Temperature and Time
Two important variables that affect the extraction efficiency are equilibration temperature and time. Therefore, the effects of these factors were studied in the range of 20 - 90°C and 5 - 30 min, respectively. According to the obtained results, the maximum extraction efficiency was achieved at 10 minutes of equilibration of the extraction mixture at 45°C.
3.1.5. Centrifuging Rate and Time
Centrifugation rate and time are two critical factors that assist the separation of the extraction phase from the aqueous phase, so their effects were studied on the extraction efficiency. The obtained results showed that the highest extraction efficiency would be achieved at 10 minutes of centrifugation at 3,000 rpm.
3.1.6. Effect of Salt Concentration
To investigate the dependence of the mixed micellar system extraction performance on the ionic strength, we examined the extraction efficiency of the system in the presence of various amounts of NaCl (0.45 - 1.0%). As shown in Figure 5, the absorbance was increased with the increase in the salt concentration from 0.45% to 0.75% and then decreased at higher NaCl concentrations. As a consequence of the salting-out effect at salt concentrations up to 0.75%, a decrease in the solubility of the organic IL phase occurred that facilitated the phase separation step. On the other hand, the IL phase started to dissolve at higher salt concentrations. Thus, complete phase separation was impossible, and a decrease in the extraction efficiency was inevitable. Hence, 0.75% was chosen as the optimum concentration of NaCl.
Effect of salt concentration (m/v) on the extraction efficiency. Experimental conditions: Cobalt concentration, 1 mg L-1; SAIL concentration, 0.3 mM; Triton X-114, 0.9 mL (0.1%); APDC, 0.06 mL (0.02 M); pH 6.
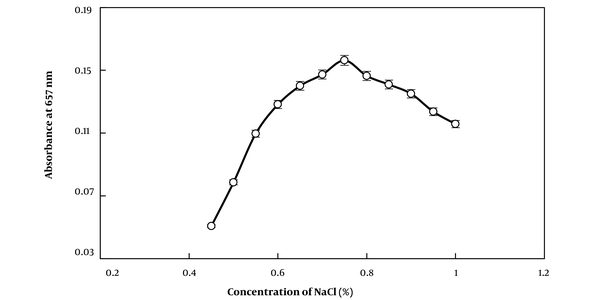
3.2. Optimization Using RSM Based on Box-Behnken Design
Applying the Box–Behnken design, a quadratic model was obtained to relate the analytical signal to the effective factors and their interactions. According to Appendix 2, all factors such as pH, ionic liquid, APDC, NaCl, and Triton X-114, except for their interactions [(pH × SAIL), (pH × APDC), (pH × Triton X-114), (pH × NaCl), (SAIL × APDC), (SAIL × TritonX-114), (SAIL × NaCl), (APDC × TritonX-114), (APDC × NaCl), and (Triton X-114 × NaCl)] were effective on the extraction efficiency. By ignoring the interactions from the suggested model, the following model was obtained, which is more consistent with the experimental data.
A = -2.5655 + 0.072075 pH + 0. 78696 SAIL + 4.440 APDC + 3.776 Triton X-114 + 1.3162 NaCl - 0.006097 pH × pH - 1.3502 SAIL × SAIL - 37.02 APDC × APDC - 1.9847 Triton X-114 × Triton X-144 - 0.8623 NaCl × NaCl
The model adequacy was evaluated by various statistical analysis tests such as the analysis of variance, residual analysis, and coefficient of determination. According to the ANOVA results in Appendices 2 and 3, all of the main effects, except for their interactions, were significant (P < 0.05), and the lack of fit of the model was not significant (P > 0.719) confirming the validity of the model. The validation was also done using the coefficient of determination (R2) and the analysis of residuals. The R2 (0.9983), R2 adj. (0.9978), and R2 pred. (0.9971) were much close to one, showing the adequacy of the proposed model. The analyses of residuals confirmed a normal distribution, which is the other evidence of the model's validity (Figure 6). The response surface plots (Appendix 4) represent the effects of experimental variables and their mutual interactions on the analytical signal. These results were obtained by varying two important factors, while the third factor (in the middle point) was kept constant. These surfaces also showed the main five factors' effects (pH, SAIL, APDC, NaCl, and Triton X-114) on the analytical signal. Derringer's desirability function (D) was used to find the optimal conditions to maximize the analytical signal. The overall desirability is a geometric mean of the individual desirability values (di) that should be close to one (39, 40). The optimum conditions were as follows: pH = 5.8939, SAIL concentration (mM): 0.2899, APDC volume (mL): 0.0599, NaCl concentration (%): 0.7641, and Triton X-114 volume (mL): 0.9515, which are required for the maximum analytical signal with overall desirability of D = 1. These results are in good agreement with those obtained without implementing the experimental design.
Normal probability plot of residuals for extraction efficiency of cobalt ions
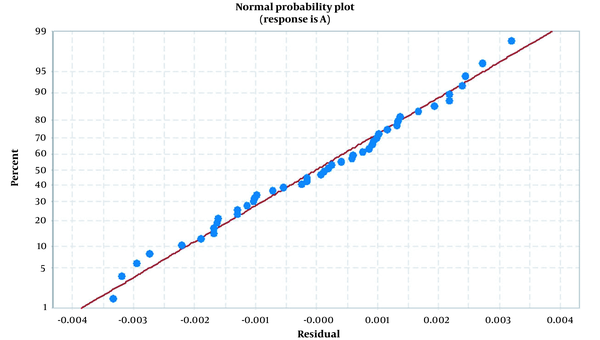
3.3. Interference Study
To evaluate the selectivity of the described SAIL-assisted CPE method, we investigated the effects of some possible interfering ions in the determination of cobalt. The cobalt content of a 10 mL sample of standard solution (0.2 mg/L) was measured in the presence of various amounts of the examined interfering ions. A 5% change in the analytical signal was considered a criterion for evaluating the effect of external ions as interference. The recovery results are summarized in Table 2.
Tolerance Limits of Interfering Ions in the Determination of Cobalt Ions by Surface-active Ionic Liquid-assisted Cloud Point Extraction Method
Tested Ion | Foreign Ion to Cobalt Concentration Ratio | Recovery (%) |
---|---|---|
Ca2+ | 1000 | 97.5 |
Mg2+ | 1000 | 103.0 |
Cl- | 1000 | 103.0 |
Na+ | 1000 | 95.0 |
NO3- | 1000 | 98.5 |
Mn2+ | 100 | 95.5 |
Hg2+ | 50 | 104.0 |
Cu2+ | 20 | 97.0 |
Pb2+ | 50 | 96.0 |
Cr3+ | 50 | 101.0 |
Zn2+ | 50 | 103.0 |
Fe3+ | 10 | 98.0 |
Ni2+ | 50 | 96.0 |
Fe2+ | 20 | 97.0 |
3.4. Analytical Performance
The analytical features of the proposed method in the presence and absence of C16MeImCl were investigated under optimal experimental conditions. With the presence of C16MeImCl in the micellar system, a wider linear range and lower detection limit were obtained, and the relative standard deviation was decreased from 2.0% to 0.9%, indicating the effect of C16MeImCl on the complete separation of the extraction phase from the aqueous phase, which directly affects the repeatability. In addition, the improvement of the enhancement factor is another considerable point that shows the favorable influence of the SAIL on extraction efficiency (Table 3).
Comparison of Analytical Characteristics of the Proposed Method in the Presence and Absence of Surface-active Ionic Liquid
3.5. Analysis of Real Samples
To validate the capability of the proposed method for the determination of cobalt ions in real samples, we applied the developed procedure to assay the cobalt content of real samples, including vitamin B12 tablets and ampoules. The results were compared to those obtained by applying the standard method (24). As the recovery results show (Table 4), there was a good agreement between them. In addition, considering the results obtained by analyzing the spiked samples, the acceptable accuracy of the proposed method was confirmed.
Determination of Cobalt Ions in Real Samples by Surface-active Ionic Liquid-assisted Cloud Point Extraction Method
Sample | Cobalt Content a | Spiked | Found b | Recovery (%) |
---|---|---|---|---|
B12 tablet | 0.325 (mg g-1) | - | 0.310 ± 0.003 (0.30 ± 0.001) c | 103.3 |
0.1 (mg g-1) | 0.410 ± 0.005 | 96.5 | ||
0.5 (mg g-1) | 0.820 ± 0.009 | 99.4 | ||
1.0 (mg g-1) | 1.290 ± 0.012 | 97.4 | ||
B12 Ampoule | 43.48 (µg mL-1) | - | 42.50 ± 0.38 (42.0 ± 0.16) c | 101.1 |
10.0 (µg mL-1) | 52.35 ± 0.47 | 97.9 | ||
20.0 (µg mL-1) | 62.80 ± 0.57 | 98.9 | ||
40.0 (µg mL-1) | 84.30 ± 0.76 | 101.0 |
4. Conclusions
A SAIL-based mixed micellar system combined with CPE was proposed for extraction and preconcentration of cobalt ions in water, vitamin B12 tablets, and ampoule samples. The results were compared with the data obtained using the conventional CPE method. According to our observations, the SAIL could improve the extraction efficiency and precision of the analytical data obtained by the mixed micellar cloud point extraction process. In addition, using a SAIL instead of a hydrophobic IL enabled us to attain the benefits of the unique properties of ILs besides the profits of a homogeneous extraction medium, without the need for a dispersing agent. These specifications distinguish the proposed method from the conventional hydrophobic-IL-based microextraction methods. The proposed method offers a rapid, simple, sensitive, precise, and inexpensive alternative to the other separation/preconcentration techniques.
Acknowledgements
References
-
1.
Li HB, Chen F. Determination of vitamin B12 in pharmaceutical preparations by a highly sensitive fluorimetric method. Fresenius J Anal Chem. 2000;368(8):836-8. [PubMed ID: 11227572]. https://doi.org/10.1007/s002160000595.
-
2.
Li C, Huang C, Periasamy AP, Roy P, Wu W, Hsu C, et al. Synthesis of photoluminescent carbon dots for the detection of cobalt ions. RSC Advances. 2015;5(3):2285-91. https://doi.org/10.1039/c4ra11704b.
-
3.
Ozturk Er E, Bakirdere EG, Unutkan T, Bakirdere S. Trace determination of cobalt in biological fluids based on preconcentration with a new competitive ligand using dispersive liquid-liquid microextraction combined with slotted quartz tube-flame atomic absorption spectrophotometry. J Trace Elem Med Biol. 2018;49:13-8. [PubMed ID: 29895362]. https://doi.org/10.1016/j.jtemb.2018.04.027.
-
4.
Ebrahimi M, Khoushnoud MJ, Zia-Behbahani M. Effect of vitamins B1, B6, and B12 (Neurobion) on Diisopropylfluorophosphate-induced Delayed Neuropathy in Mice. Iran J Pharm Res. 2018;17(3):1116-24. [PubMed ID: 30127834]. [PubMed Central ID: PMC6094411].
-
5.
Shahat A, Awual MR, Naushad M. Functional ligand anchored nanomaterial based facial adsorbent for cobalt(II) detection and removal from water samples. J Chem Eng. 2015;271:155-63. https://doi.org/10.1016/j.cej.2015.02.097.
-
6.
Han Q, Huo Y, Yang X, He Y, Wu J, Cai H. Determination of ultra-trace levels of cobalt in water and wheat flour samples using cloud point extraction coupled with laser thermal lens spectrometry. Anal Methods. 2018;10(6):634-40. https://doi.org/10.1039/c7ay02522j.
-
7.
Chaiyamate P, Seebunrueng K, Srijaranai S. Vortex-assisted low density solvent and surfactant based dispersive liquid-liquid microextraction for sensitive spectrophotometric determination of cobalt. RSC Adv. 2018;8(13):7243-51. [PubMed ID: 35540320]. [PubMed Central ID: PMC9078415]. https://doi.org/10.1039/c7ra11896a.
-
8.
Welton T. Room-Temperature Ionic Liquids. Solvents for Synthesis and Catalysis. Chem Rev. 1999;99(8):2071-84. [PubMed ID: 11849019]. https://doi.org/10.1021/cr980032t.
-
9.
Gao S, Yang X, Yu W, Liu Z, Zhang H. Ultrasound-assisted ionic liquid/ionic liquid-dispersive liquid-liquid microextraction for the determination of sulfonamides in infant formula milk powder using high-performance liquid chromatography. Talanta. 2012;99:875-82. [PubMed ID: 22967637]. https://doi.org/10.1016/j.talanta.2012.07.050.
-
10.
Gong A, Zhu X. Dispersive solvent-free ultrasound-assisted ionic liquid dispersive liquid-liquid microextraction coupled with HPLC for determination of ulipristal acetate. Talanta. 2015;131:603-8. [PubMed ID: 25281147]. https://doi.org/10.1016/j.talanta.2014.08.021.
-
11.
Tuzen M, Uluozlu OD, Mendil D, Soylak M, Machado LOR, Dos Santos WNL, et al. A simple, rapid and green ultrasound assisted and ionic liquid dispersive microextraction procedure for the determination of tin in foods employing ETAAS. Food Chem. 2018;245:380-4. [PubMed ID: 29287384]. https://doi.org/10.1016/j.foodchem.2017.10.115.
-
12.
Tuzen M, Pekiner OZ. Ultrasound-assisted ionic liquid dispersive liquid-liquid microextraction combined with graphite furnace atomic absorption spectrometric for selenium speciation in foods and beverages. Food Chem. 2015;188:619-24. [PubMed ID: 26041239]. https://doi.org/10.1016/j.foodchem.2015.05.055.
-
13.
Zeng H, Wang Y, Kong J, Nie C, Yuan Y. Ionic liquid-based microwave-assisted extraction of rutin from Chinese medicinal plants. Talanta. 2010;83(2):582-90. [PubMed ID: 21111178]. https://doi.org/10.1016/j.talanta.2010.10.006.
-
14.
Tuzen M, Kazi TG, Citak D, Soylak M; Naeemullah. Pressure-assisted ionic liquid dispersive microextraction of vanadium coupled with electrothermal atomic absorption spectrometry. J Anal At Spectrom. 2013;28(9):1441. https://doi.org/10.1039/c3ja50174d.
-
15.
Bamdad F, Ardalani M, Sangi MR. Trace Determination of Cadmium Ions by Flame Atomic Absorption Spectrometry after Pre-Concentration Using Temperature-Controlled Ionic Liquid Dispersive-Liquid Phase Microextraction. J Braz Chem Soc. 2013;2:246-52. https://doi.org/10.5935/0103-5053.20130290.
-
16.
Zhou Q, Bai H, Xie G, Xiao J. Trace determination of organophosphorus pesticides in environmental samples by temperature-controlled ionic liquid dispersive liquid-phase microextraction. J Chromatogr A. 2008;1188(2):148-53. [PubMed ID: 18346747]. https://doi.org/10.1016/j.chroma.2008.02.094.
-
17.
Amde M, Liu JF, Tan ZQ, Bekana D. Ionic liquid-based zinc oxide nanofluid for vortex assisted liquid liquid microextraction of inorganic mercury in environmental waters prior to cold vapor atomic fluorescence spectroscopic detection. Talanta. 2016;149:341-6. [PubMed ID: 26717850]. https://doi.org/10.1016/j.talanta.2015.12.004.
-
18.
Bagda E, Tuzen M. A simple and sensitive vortex-assisted ionic liquid-dispersive microextraction and spectrophotometric determination of selenium in food samples. Food Chem. 2017;232:98-104. [PubMed ID: 28490150]. https://doi.org/10.1016/j.foodchem.2017.03.104.
-
19.
Fan Y, Liu S, Xie Q. Rapid determination of phthalate esters in alcoholic beverages by conventional ionic liquid dispersive liquid-liquid microextraction coupled with high performance liquid chromatography. Talanta. 2014;119:291-8. [PubMed ID: 24401417]. https://doi.org/10.1016/j.talanta.2013.11.023.
-
20.
Padro JM, Marson ME, Mastrantonio GE, Altcheh J, Garcia-Bournissen F, Reta M. Development of an ionic liquid-based dispersive liquid-liquid microextraction method for the determination of nifurtimox and benznidazole in human plasma. Talanta. 2013;107:95-102. [PubMed ID: 23598198]. https://doi.org/10.1016/j.talanta.2012.12.050.
-
21.
Bamdad F, Raziani A. Application of surface-active ionic liquids in micelle-mediated extraction methods: pre-concentration of cadmium ions by surface-active ionic liquid-assisted cloud point extraction. J Iran Chem Soc. 2019;17(2):327-32. https://doi.org/10.1007/s13738-019-01770-8.
-
22.
Donati GL, Nascentes CC, Nogueira ARA, Arruda MA, Nóbrega JA. Acid extraction and cloud point preconcentration as sample preparation strategies for cobalt determination in biological materials by thermospray flame furnace atomic absorption spectrometry. Microchem J. 2006;82(2):189-95. https://doi.org/10.1016/j.microc.2006.01.006.
-
23.
Song Z, Hou S. Sub-picogram determination of Vitamin B12 in pharmaceuticals and human serum using flow injection with chemiluminescence detection. Analytica Chimica Acta. 2003;488(1):71-9. https://doi.org/10.1016/s0003-2670(03)00665-2.
-
24.
Stoica AI, Peltea M, Baiulescu GE, Ionica M. Determination of cobalt in pharmaceutical products. J Pharm Biomed Anal. 2004;36(3):653-6. [PubMed ID: 15522545]. https://doi.org/10.1016/j.jpba.2004.07.030.
-
25.
Eftekhari M, Javedani-Asleh F, Chamsaz M. Ultra-Trace Determination of Co (II) in Real Samples Using Ion Pair-Based Dispersive Liquid-Liquid Microextraction Followed by Electrothermal Atomic Absorption Spectrometry. Food Anal Methods. 2015;9(7):1985-92. https://doi.org/10.1007/s12161-015-0378-1.
-
26.
Beikzadeh E, Sarrafi AH. Determination of trace levels of cobalt ion in different real samples using dispersive liquid–liquid microextraction followed by flame atomic absorption spectrometry. J Food Meas Charact. 2017;11(3):994-1002. https://doi.org/10.1007/s11694-017-9474-9.
-
27.
Shirani M, Salari F, Habibollahi S, Akbari A. Needle hub in-syringe solid phase extraction based a novel functionalized biopolyamide for simultaneous green separation/preconcentration and determination of cobalt, nickel, and chromium (III) in food and environmental samples with micro sampling flame atomic absorption spectrometry. Microchem J. 2020;152:104340. https://doi.org/10.1016/j.microc.2019.104340.
-
28.
Sorouraddin SM, Farajzadeh MA, Ghorbani M. In situ-produced CO2-assisted dispersive liquid–liquid microextraction for extraction and preconcentration of cobalt, nickel, and copper ions from aqueous samples followed by graphite furnace atomic absorption spectrometry determination. J Iran Chem Soc. 2017;15(1):201-9. https://doi.org/10.1007/s13738-017-1224-8.
-
29.
Temel NK, Sertakan K, Gurkan R. Preconcentration and Determination of Trace Nickel and Cobalt in Milk-Based Samples by Ultrasound-Assisted Cloud Point Extraction Coupled with Flame Atomic Absorption Spectrometry. Biol Trace Elem Res. 2018;186(2):597-607. [PubMed ID: 29777525]. https://doi.org/10.1007/s12011-018-1337-7.
-
30.
Deniz S, Kasa A, Sel S, Büyükpınar Ç, Bakırdere S. Sensitive and Accurate Determination of Cobalt at Trace Levels by Slotted Quartz Tube-Flame Atomic Absorption Spectrometry Following Preconcentration with Dispersive Liquid–Liquid Microextraction. Anal Lett. 2018;52(5):745-53. https://doi.org/10.1080/00032719.2018.1493737.
-
31.
Li Y, Peng G, He Q, Zhu H, Al-Hamadani SM. Dispersive liquid-liquid microextraction based on the solidification of floating organic drop followed by ICP-MS for the simultaneous determination of heavy metals in wastewaters. Spectrochim Acta A Mol Biomol Spectrosc. 2015;140:156-61. [PubMed ID: 25590827]. https://doi.org/10.1016/j.saa.2014.12.091.
-
32.
Bartosiak M, Jankowski K, Giersz J. Determination of cobalt species in nutritional supplements using ICP-OES after microwave-assisted extraction and solid-phase extraction. J Pharm Biomed Anal. 2018;155:135-40. [PubMed ID: 29627730]. https://doi.org/10.1016/j.jpba.2018.03.058.
-
33.
Yang FY, Jiang SJ, Sahayam AC. Combined use of HPLC-ICP-MS and microwave-assisted extraction for the determination of cobalt compounds in nutritive supplements. Food Chem. 2014;147:215-9. [PubMed ID: 24206708]. https://doi.org/10.1016/j.foodchem.2013.09.141.
-
34.
Sorouraddin SM, Farajzadeh MA, Nouri S, Afshar Moghaddam MR. Air-assisted liquid liquid microextraction combined with graphite furnace atomic absorption spectrometry for preconcentration and determination of trace amount of Co (II) and Ni (II) ions in water samples. Anal Bioanal Chem Res. 2017;4(2):227-38.
-
35.
Han Q, Huo Y, Yang X, He Y, Wu J. Dispersive liquid–liquid microextraction coupled with graphite furnace atomic absorption spectrometry for determination of trace cobalt in environmental water samples. Int J Environ Anal Chem. 2019;100(8):945-56. https://doi.org/10.1080/03067319.2019.1645843.
-
36.
Babos DV, Barros AI, Ferreira EC, Neto JAG. Evaluation of solid sampling for determination of Mo, Ni, Co, and V in soil by high-resolution continuum source graphite furnace atomic absorption spectrometry. Spectrochim Acta B: At Spectrosc. 2017;130:39-44. https://doi.org/10.1016/j.sab.2017.02.005.
-
37.
Ferreira SL, Bruns RE, Ferreira HS, Matos GD, David JM, Brandao GC, et al. Box-Behnken design: an alternative for the optimization of analytical methods. Anal Chim Acta. 2007;597(2):179-86. [PubMed ID: 17683728]. https://doi.org/10.1016/j.aca.2007.07.011.
-
38.
Hulanicki A. Complexation reactions of dithiocarbamates. Talanta. 1967;14(12):1371-92. [PubMed ID: 18960244]. https://doi.org/10.1016/0039-9140(67)80159-0.
-
39.
Derringer G, Suich R. Simultaneous Optimization of Several Response Variables. J Qual Technol. 2018;12(4):214-9. https://doi.org/10.1080/00224065.1980.11980968.
-
40.
Bourguignon B, Massart DL. Simultaneous optimization of several chromatographic performance goals using Derringer's desirability function. J Chromatogr A. 1991;586(1):11-20. https://doi.org/10.1016/0021-9673(91)80019-d.