Abstract
Keywords
Anti-Helicobacter pylori Urease inhibitory Bioactive phytochemicals Molecular docking Oliveria decumbens Vent
Introduction
Helicobacter pylori infection has been identified as the main cause of gastrointestinal disorders such as duodenal ulcer disease, peptic ulcer disease, gastric carcinoma, primary gastric B cell lymphoma, and hyperemesis gravidarum (1). The prevalence of diseases associated with H. pylori infection and the corresponding health care costs highlight the importance of the subject and the development of efficient therapeutic strategies based on anti-H. pylori agents (2).
Urease is a nickel-containing enzyme that plays a vital role in inducing H. pylori infection since it provides the desired pH for surviving this pathogen. As urease is one of the virulence factors responsible for H. pylori colonization, urease inhibition has been a strong tool for treating H. pylori infection (3).
Nowadays, medicinal plants and natural products are highly in demand around the world due to their versatile pharmaceutical and medicinal properties (4). There is a lot of research focusing on the antibacterial activity of the plants as well as isolated bioactive compounds because of failure in the treatment of infections by conventional antibiotics (5).
Phenolic compounds and flavonoids are abundant and ubiquitous in natural products, which have shown efficient anti-H.pylori and antibacterial properties through various mechanisms (5-7). For example, quercetin is known for the inhibition of H. pylori urease as well as bacterial DNA gyrase (8). According to Zhang et al. (2009) report, apigenin inhibits the β‐hydroxyacyl‐acyl carrier protein dehydratase from H. pylori (HpFabZ) (9). Kaempferol derivatives have also depicted antibacterial activity (10) and considerable inhibitory effects against the growth of H. pylori (11).
The literature review shows that the plant species in the family Apiaceae are a remarkable source of flavonoids, mainly flavonols and flavones (12). Oliveria decumbens Vent. belongs to the family Apiaceae which is distributed in the west and southwest of Iran and traditionally used for the treatment of pulmonary infections and gastrointestinal diseases (13). Indigenous people of the southwest of Iran used aqueous infusion or dried powders of aerial parts of O. decumbens locally known as “Moshkoorak” to treat peptic ulcer and gastrointestinal infections and relieve thirst in children (14).
Previous studies revealed that O. decumbens essential oil possessed antibacterial and antifungal activities because of a high percentage of thymol and carvacrol (15). A previous study revealed that hydroalcoholic extract of O. decumbens demonstrated remarkable antibacterial activity against methicillin- and cefixime-resistant Staphylococcus aureus strains(16). Although there are several reports on the biological properties of the plant, no complementary phytochemical studies have been achieved, and they usually are limited to essential oil analysis (15).
In the present study, we were focusing on the probable anti-H. pylori activity of O. decumbens according to traditional medicine and available studies, anti-H. pylori, urease inhibitory, and antibacterial activities of different fractions of O. decumbens aerial parts were evaluated. Also, phytochemical analysis of aerial parts of O. decumbens was conducted, and isolated compounds were a candidate to be evaluated against urease inhibition. Finally, molecular docking was performed to recognize possible interaction modes between those compounds and urease active sites.
Experimental
General procedures
All solvents and materials were analytical grades. Silica gel with different mesh (Merck), Sephadex LH-20 (Fluka, BioChemika), and RP-18 (Fluka) was used for column chromatography. TLC aluminum sheets (Si gel 60 F254 and RP_18 F254) (Merck) were used for monitoring of spots under UV. 1HNMR and 13CNMR spectra were recorded on a Bruker NMR 500 spectrometer (Bruker, Germany). Chemical shifts (δ) were expressed in ppm using tetramethylsilane (TMS) as the internal standard, and coupling constants were calculated (J) in Hz.
Collection and authentication of plant
The aerial parts of O. decumbens Vent. were collected in June 2014 from Kohgiluyeh and Boyer Ahmad province, Iran. The sample was authenticated and deposited in the Central Herbarium of Tehran University, Tehran, Iran (Voucher specimen: 451500 TUH).
Extraction and fractionation
Dried and finely powdered of the plant (1500 g) were extracted by methanol/distilled water (80/20) at room temperature three times using the maceration method. After removal of the solvent under vacuum at 40 ºC, the residue known as total extract was subjected to liquid-liquid extraction by different solvents (n-hexane, chloroform, and ethyl acetate). The residue left after fractionation was solved in methanol and filtered off to remove the solid. In each step, the solvent was evaporated, and the concentrates were lyophilized.
Evaluation of anti-Helicobacter pylori activity
Anti-Helicobacter pylori activity of the samples was investigated by an agar dilution method according to our previous study (17). The classic Clinical and Laboratory Standards Institute methods use broth microdilution only for conventional antibiotics, and modifications of those methods are suggested for other substances such as natural health products (NHPs). The Agar dilution method has been standardized previously as an appropriate method to determine minimum inhibitory concentrations (MIC) of water-insoluble and colored plant extracts. The Agar dilution method was performed in Brucella agar plates supplemented with 20% fetal bovine serum (FBS, Gibco, USA) containing different concentrations of the plant extract or fractions ranging from 50 to 2000 μg/mL. A volume of 100 μL standardized H. pylori inoculum strain RIGLD-HC180 obtained from antral biopsy samples of patients at Taleghani Hospital, Tehran, Iran (equal to 5 × 105 cfu) was spread onto the plates and incubated for 72 h at a microaerophilic condition in a jar containing Anaerocult® C (Merck, Germany) at 37 °C. Growth control plates were included in each experiment. The lowest concentrations of the total extract or different fractions that inhibited visible bacterial growth (containing no colonies) were expressed as minimum inhibitory concentrations (MICs). All the experiments were performed in triplicate.
Plates, including amoxicillin, were used as a positive control (17).
Evaluationof antibacterial activity
Antibacterial activity of total extract and different fractions were examined by a disc diffusion method based on the standard CLSI M100 against two Gram-positive bacteria, Staphylococcus aureus ATCC 6538, and Staphylococcus epidermidis ATCC 12229 and two Gram-negative bacteria, Escherichia coli ATCC 8739 and Pseudomonasaeruginosa ATCC 9027 as previously reported (17). Briefly, overnight cultures of bacteria were used to prepare a bacterial inoculum in sterile normal saline. The bacterial inoculum was standardized to the turbidity of 0.5 McFarland (the density equivalent to 1.5 × 108 cfu/mL). The surface of Mueller-Hinton (MH) agar plates was covered by 100 µL of diluted bacterial suspension containing 5 ×105 cfu. Different concentrations of the plant extract or fraction were prepared. Sterile blank discs (6.4 mm diameter) containing different concentrations of the plant extract or fractions (15 μL) were placed on the agar plates. The mean inhibition zone diameter was measured after overnight incubation at 37 °C. Ciprofloxacin and penicillin discs were used as positive controls (17).
Isolation and purification
n-Hexane, ethyl acetate, and methanol fractions were selected for phytochemical investigations. n-Hexane fraction (5.7 g) was placed on silica gel (30-70 mesh, 5 × 50 cm) and eluted with a gradient mixture of EtOAc/n-hexane (0:10 to 10:0) obtained six fractions (1-6). Fraction 1 afforded purified compound 1 as a colorless oil (30 mg). Fraction 2 was subjected to silica gel (70-230 mesh, 1.5 × 50 cm), and four fractions were obtained (2a-2d). Fraction 2b was subjected to Sephadex LH-20 to obtain compounds 2 and 3 (red oil, 40 mg) mixture. Moreover, compound 4 (white crystals, 25 mg) were afforded from fraction 2c.
The ethyl acetate fraction (1 g) was chromatographed on a column of silica gel (30-70 mesh, 1.5 × 50 cm) eluted with a gradient mixture of EtOAc /n-hexane (0:10 to 10:0) to yield ten fractions (1-10). Fractions 4 and 5 were further separated on a Sephadex LH-20 column to give compound 5 (22.8 mg, Rf = 0.6 using ethyl acetate/methanol (9:1)) and compound 6 (33.2 mg, Rf = 0.8 using ethyl acetate/methanol (9:1)). Both compounds 5 and 6 were obtained as yellow crystals, which were observed as black spots under UV light at 254 on a TLC sheet. Moreover, these spots were shown in yellow when sprayed with p-anisaldehyde-sulfuric acid and then heated.
Fraction 6 was subjected to Sephadex LH-20, and four fractions (6a-6d) were yielded. Fraction 6c was moved to reverse-phase silica to obtain the mixture of compounds 7 and 8 (86 mg, amorphous powder, Rf = 0.5 using ethyl acetate/methanol (9:1) system). Those corresponding spots were black under UV light at 254 and were appeared as purple spots when sprayed with p-anisaldehyde-sulfuric acid.
Furthermore, methanol fraction (10 g) was moved on a vacuum column chromatography (VLC) (silica gel 30-70 mesh, 10× 15 cm) eluted with a gradient mixture of MeOH/ CHCl3 (0:10 to 10:0) to yield seven fraction (1-7). Fraction 4 was further separated using Sephadex LH-20 to obtain compound 9 (22.2 mg, Rf = 0.77 using quaternary solvent system, ethyl acetate/water/acetic acid/formic acid (100:26:11:11). The compound was obtained as yellow crystals, which were observed as a black spot under UV light at 254 on a TLC sheet and a yellow spot after spraying with the reagent (p-anisaldehyde-sulfuric acid) and subsequently heating.
The structure of all compounds was identified by a spectroscopic analysis comparing with those reported in the literature.
Evaluation of urease inhibitory activity
Urease inhibitory activity was evaluated based on Tanaka et al. report (18). Jack bean urease (Sigma-Aldrich, Germany) was used as a surrogate for H. pylori urease. Each well of a 96-well plate contained 25 μL jack bean urease (6U, Sigma-Aldrich) and 25 μL different concentrations of tested materials that were prepared in phosphate buffer (PBS, pH 6.8 ) and DMSO 1% as a co-solvent. After 30 min incubation at room temperature, 200 μL of a stock solution composed of PBS (100 mM), urea (150 mM), and phenol red were added to each well. The absorbance changes were measured at 570 nm by a microplate reader. Hydroxyurea was used as the positive control (18).
Molecular docking of isolated compoundsfromO. decumbenson the urease active site
To get insight into possible interaction modes between active sites of jack bean urease and isolated compounds from O. decumbens, the molecular docking study was performed using Autodock software version 4.2 (19). A three-dimensional (3D) X-ray crystal structure of urease (E.C. 3.5.1.5) with 4H9M PDB code was obtained from Protein Data Bank (PDB) at the 1.52 Å resolution of Jack bean (Canavalia ensiformis) (20). The 2D structures of the chemical molecules were sketched and optimized using HyperChem software version 7 (Hypercube, Inc., Gainesville, FL, USA; http://www.hyper.com) as previously described (21). The canter of the grid box was positioned on the centroids of the residues, including Kcx490, His492, His519, His545, Asp633, Ni901, and Ni902 at the coordinates X = 19.067, Y = -56.327, Z = -21.334 (22). The Lamarckian genetic algorithm (LGA) as the most effective method was considered (23). For conventional systems, AutoDock was run several times (here 200 times) as previously described. Among the various conformations obtained from the docking study for each compound, the conformation with the lowest binding free energy was selected for more assessment of binding mode (24). The resulting 2D poses were analyzed visually to understand the interaction pattern by the Ligplot software (25).
Results
Compounds isolation and identification
The structure of isolated and identified compounds from O. decumbens was depicted in Figure 1 (1-9).
Compound 1 was obtained as a colorless oil from the n-hexane fraction. Based on NMR spectrum (Figure S1a, in supplementary file) and ESI-MS analysis (Figure S1b) and compared with available data (26, 27), compound 1 was identified as octacosane (C28H58).
Compounds 2 and 3 were mixed together. According to the NMR spectrum of the mixture (Figure S2) in comparison with available data as well as TLC analysis comparing with available standards, it was identified to be the mixture of carvacrol and thymol (28, 29).
Compound 4 was obtained as white crystals, identified as stigmasterol based on NMR spectra (Figure S3), and compared with available literature (30).
Compound 5 was obtained as yellow crystals and identified as 3‐O‐β‐‐(6″‐O‐coumaroyl)glucopyranoside (tiliroside) according to 13H- and 13CNMR spectra as well as available data (31-33). 1HNMR (500 MHz, DMSO-d6): 8.02 (2H, d, J = 8.5 Hz, H-2’, H-6’), 7.59 (d, 1H, J = 16.0 Hz, H- γ), 7.54 ( 2H, d, J = 8.0 Hz, H-2’’, H-6’’), 6.89 (2H, d, J = 8.5Hz, H-3’, H-5’), 6.79 (2H, d, J = 8.0 Hz, H-3’’, H-5’’),6.40 (1H, d, J = 16.0 Hz, H-β), 6.40 ( 1H, s, H-8), 6.17 ( 1H, s, H-6), 5.75 ( 1H, d, J = 7.5 Hz, H-1’’), 3.35- 4.87 (6H, m, the rest of sugars protons). 13CNMR (125 MHz, DMSO-d6): 177.1 (C-4), 165.8 (C- α), 164.5 (C-7), 161.1 (C-5), 160.0 (C-4’’’), 159.8 (C-4’), 156.3 (C-2), 156.1 (C-9), 144.9 (C-γ), 132.5 (C-3), 130.7 (C-2’’’/C-6’’’), 130.3 (C-2’/C-6’), 125.1 (C-1’), 120.7 (C-1’’’), 115.8 (C- β), 115.1 (C-3’/C-5’) , 114.3 (C-3’’’/C-5’’’), 103.8 (C-10), 98.7 (C-1’’), 98.1 (C-6), 93.7 (C-8), 77.7 (C-2’’), 74.1 (C-3’’), 70.1(C-4’’), 60.6 (C-5’’), 48.9 (C-6’’)( Figures S4 & S5).
Compound 6 was obtained as yellow crystals. According to the following NMR characterization and comparing those data with the literature (34) it was identified as kaempferol-3-O-(6’’-O-trans-coumaroyl) glucopyranoside 7-O- 6’’’’’ coumaroyl glucopyranoside. 1HNMR (500 MHz, DMSO-d6): 8.05 (2H, d, J = 8.0 Hz, H-2’, H-6’), 7.97 (2H, d, J = 8.0 Hz, H-2’’, H-6’’), 7.62 (d, 1H, J = 15.0 Hz, H- γ), 7.55 (2H, d, J = 8.0 Hz, H-2’’’, H-6’’’’), 7.36 (d, 1H, J = 15.0 Hz, H- γ´), 6.93 (2H, d, J = 8.0 Hz, H-3’, H-5’), 6.87 (2H, d, J = 8.0 Hz, H3’’’, H-5’’’’ ),6.80 (2H, d, J = 8.0 Hz, H3’’’’, H-5’’’), 6.42 (1H, s, H-8 ), 6.40 (1H, d, J = 15.0 Hz, H-β ), 6.17 ( 1H, d, J = 15.0 Hz, H-β´), 6.13 ( 1H, s, H-6), 5.84 (1H, d, J = 7.5 Hz, H-1’’’), 5.74 ( 1H, d, J = 7.5 Hz, H-1’’), 3.35- 4.87 (the rest of sugars protons). 13C NMR (125 MHz, DMSO-d6): 177.1 (C-4), 166.1 (C-α), 165.8 (C-α´), 165.7 (C-7), 165.0 (C-4’’’’’), 164.3 (C-4’’’), 160.1 (C-5), 159.9 (C-4’), 159.9 (C-2), 156.4 (C-9), 145.2 (C- γ), 144.6 (C-γ´), 132.5 (C-3), 131.3 (C-2’’’, C-6’’’), 130.9 (C-2’, C-6’), 130.3 (C-2’’’’’, C-6’’’’’), 125.1 (C-1’), 125.0 (C-1’’’), 124.9 (C-1’’’’), 115.8 (C-β), 115.2 (C-β´), 115.1 ( C-3’, C-5’) , 114.3 (C-3’’’, C-5’’’), 113.8 (C-3’’’’’, C-5’’’’’), 103.9 (C-1’’’’), 103.8 (C-1’’), 98.3 (C-10), 98.2 (C-6), 93.7 (C-8), 75.2 (C-2’’’’), 74.6 (C-2’’), 74.3 (C-3’’’’), 73.9 (C-3’’), 71.9 (C-4’’’’), 71.4 (C-4’’), 71.0 (C-5’’’’’), 70.2 (C-5’’), 62.8 (C-6’’’’), 60.5 (C-6’’). 1H-1H COSY spectrum showed connectivities among H-2’ and H-3’ as well as H-5’ and H-6’ on the B ring of kaempferol. Also, the correlation between H-2’’’ and H-3’’’, H-5’’’ and H-6’’’, H-2’’’’’ and H-3’’’’’, and H-5’’’’’’ and H-6’’’’’ on the aromatic rings of coumaroyl moieties attached to glucopyranoside group at C-3 and C-7 of kaempferol, respectively was demonstrated. It should be noted that desired correlations between olefinic protons (β /γ and β’ /γ ‘) of coumaroyl moieties were observed. Major NOSEY correlations showed that B ring protons of kaempferol correlated with protons of aromatic ring of cumaroyl moiety attached to glucopyranoside at C-3. In addition, there was a correlation between olefinic protons (β’/γ’) coumaroyl moiety attached to glucopyranoside at C-7 and H-8 (Figures S6-S9).
Compound 7 was identified as 3-hydroxythymol-6-O-glucopyranoside according to data from NMR spectra and available literatures (35, 36). 1HNMR (500 MHz, DMSO-d6): 7.29 (1H, s, H-5), 6.93 (1H, s, H-2), 4.94 (1H, d, J = 7.0 Hz, H-1´), 3.85-3.99 ( 1H, m, H-8), 3.35-4.11 (6 H, m, H-2´- 6’ ), 2.5 (3H, s, H-7), 1.53 (3H, d, J = 6.8 Hz, H-9), 1.53 (3H, d, J = 6.8 Hz, H-10). 13C NMR (125 MHz, DMSO-d6): 148.9 (C-6), 147.4 (C-3), 132.1 (C-4), 121.3 (C-1), 119.1 (C-2), 111.8 (C-5), 102.8 (C-1’), 77.0 (C-2’), 76.9 (C-3’), 73.5 (C-4’), 70.1 (C-5’), 48.7 (C-6’), 25.6 (C-8), 23.1 (C-9), 22.6 (C-10), 15.8 (C-7) (Figures S10 and S11).
Compound 8 was identified as 6-hydroxythymol-3-O-glucopyranoside based on data obtained from NMR spectra and literatures (35, 37). 1HNMR (500 MHz, DMSO-d6): 7.20 (1H, s, H-5), 7.00 (1H, s, H-2), 4.94 (1H, d, J = 7.0 Hz, H-1’), 3.85-3.99 (1H, m, H-8), 3.35-4.11 (6H, m, H-2’- 6’), 2.50 (3H, s, H-7), 1.53 (3H, d, J = 6.8 Hz, H-9), 1.53 (3H, d, J = 6.8 Hz, H-10). 13C NMR (125 MHz, DMSO-d6): 150.4 (C-6), 148.8 (C-3), 136.0 (C-4), 124.9 (C-1), 116.6 (C-2), 114.6 (C-5), 103.0 (C-1’), 77.0 (C-2’), 76.9 (C-3’), 73.6 (C-4’), 73.2 (C-5’), 60.9 (C-6’), 26.2 (C-8), 23.3 (C-9), 22.7 (C-10), 15.8 (C-7) (Figures S10 and S11).
Compound 9 was yield as yellow crystals. According to 1HNMR and 13CNMR spectra as well as data obtaining from literature, it was identified as kaempferol 3-Oβ -O-neohesperidoside-7-O-[2-O-(cis-feruloyl)] β -D-glucopyranoside (34, 38, 39). 1HNMR (500 MHz, DMSO-d6): 7.98 (2H, d, J = 8.5 Hz, H-2’, H-6’ ), 7.85 ( 1H, s, H-2’’’’’), 7.52 (1H, d, J = 11.0 Hz, H-γ), 6.91 (2H, d, J = 8.5 Hz, H-3’, H-5’), 6.87 ( 1H, d, J = 8.0 Hz, H-6’’’’’ ), 6.84 (1H, d, J = 8.0 Hz, H-5’’’’’ ), 6.42 (1H, s, H-8 ), 6.39, (1H, d, J = 11.0 Hz, H-β), 6.20 (1H, s, H-6), 5.44 (1H, d, J = 7.0 Hz, H-1’’), 5.42 (1H, d, J = 7.5 Hz, H-1’’’’), 5.08 (1H, bs, H-1’’’), 3.20-4.41 (the rest of sugars protons), 3.83 (3H, s, H-3’’’’’), 0.97 (3H, d, J = 6.0 Hz, H-6’’’),.
13C NMR (125 MHz, DMSO-d6): 177.3 (C-4), 164.5 (C- α), 161.2 (C-7), 160.0 (C-5), 156.5 (C-4’), 156.4 (C-9), 149.4 (C-2), 148.4 (C-3’’’’’), 146.9 (C-4’’’’’), 144.7 (C-γ), 133.2 (C-3), 130.9 (C-6’’’’’133.0 (C-2’ , C-6’), 122.2 (C-1’’’’’), ) 121.0 (C-1’), 115.2 ( C-3’, C-5’), 115.1(C- β), 114.3 (C-5’’’’’), 113.3 (C-2’’’’’), 103.9 (C-10),101.2 (C-1’’’), 100.9 (C-1’’), 100.7 ( C-1’’’’), 98.8 (C-6), 93.8 (C-8), 76.4 (C-3’’’’), 75.9 (C-2’’), 75.7 (C-3’’), 74.3 (C-5’’), 74.1 (C-5’’’’), 71.7 (C-4’’’), 70.9 (C-2’’’’ ), 70.3 (C-2’’’), 70.2 (C-3’’’), 70.1 (C-4’’), 68.3 (C-5’’’), 68.2(C- 4’’’’), 66.9 (C-6’’), 66.8 (C-6’’’’ ), 55.6 (C3’’’’’). 17.7 (C-6’’’’) (Figures S12 and S13).
Anti-Helicobacter pylori and antibacterial activity
Anti-Helicobacter pylori activity of total extract and different fractions were assessed using different concentrations in an agar dilution method (Table 1). As shown in Table 1, the n-hexane fraction depicted the most significant anti-H. pylori activity with the MIC value of 50 µg/mL. However, the other fractions showed much lower activity against H. pylori (MICs = 1000, 1500, and 1750 µg/mL, respectively), and the total extract showed no inhibitory activity even at the concentration of 2000 µg/mL.
As reported in Table 2, all fractions demonstrated antibacterial activity toward S. aureus while all of them showed no antibacterial activity against P. aeruginosa even at a concentration up to 500 µg/mL. It should be noted that the total extract and all fractions except ethyl acetate fraction depicted no antibacterial activity toward S. epidermidis and E. coli in the tested concentration range.
Urease inhibitory
The inhibitory activity of total extract and different fractions toward jack bean urease was reported in Table 3. The best inhibitory activity was demonstrated by n-hexane (IC50 = 285.44 µg/mL) and ethyl acetate (IC50 = 285.06 µg/mL) fractions as compared to hydroxyurea as a positive control (IC50 = 59.51 µg/mL). Chloroform and methanol fractions showed lower urease inhibitory activity with IC50 values of 375.36 and 709.42 µg/mL, respectively. However, the total extract showed no inhibitory activity.
Moreover, the urease inhibitory of the isolated compounds as IC50 (mM) was evaluated (Table 4). Among them, stigmasterol, 3‐O‐β‐‐(6″‐O‐coumaroyl), glucopyranoside (tiliroside), and carvacrol were found to be the most active as compared to positive control. It should be noted that thymol showed lower activity (IC50 = 1.33 mM) than its isomer carvacrol (IC50 = 0.70 mM). The mixture of hydroxyl thymol glucosides and compound 9 showed similar anti-urease activity (Inhibition percentage at 100 (µg/mL) concentration = 24.6%), and no activity was induced by compound 6 up to 250 µg/mL.
Molecular docking of the isolated compounds in the binding site of urease
Table 5 summarized all details related to the docking study of the isolated compounds in the binding site of urease. Stigmasterol, tiliroside, and carvacrol as the most active compounds inhibited the catalytic activities of urease through hydrogen bonding, metal/ion contact with Ni ions, and hydrophobic interactions. The interaction of the best-docked conformation of the ligand with active site residues is depicted in Figures 2 and S14-S18.
The hydroxyl groups of thymol and carvacrol interacted with the Ni ion and imidazole ring of His492 (Table 5, Figure S14). In addition, the docked pose of carvacrol tightly engaged in nickel chelation along with several hydrogen bonds within the binding cavity (Figure 2a). The hydroxyl group of cyclohexyl moiety of stigmasterol highlighted the formation of a bidentate bond with two-nickel ions (1.92 Å, 3.22 Å) and Gly550 (2.66 Å) (Table 5). The substituted rings are deeply embedded into the hydrophobic pocket of the Ile411, Arg439, Ala440, His492, Asp494, Cme592, Gln635, Ala636, and Met637 (Figure 2b).
Figure 2c shows that the hydroxyl groups of kaempferol moiety in the tiliroside were also engaged in the binding cavity through nickel ions (2.14 Å, 2.67 Å) hydrogen bonds with the oxygen atom of Asp633 and Cme592 side chains. In addition, both OH groups of glucoside moiety mediated through two hydrogen bonds with the side chain of Arg439. The lowest free binding energy of tiliroside was -8.49 kcal/mol with Ki of 598.78 nM. This is in good accordance with the experimentally observed value of urease inhibition percentage.
Similarly, compound 7 with glucopyranoside group at position 6 showed slightly better results than its counterpart (compound 8). The different OH groups of compound 7 formed hydrogen bonding and polar bonds with Arg439, Ala440, Kcx490, His492, Asp494, Gly550, Arg609, Asp633, and nickel ions (Table 5, Figure S15). As shown in figure S16, the non-glucopyranoside moiety of compound 8 was observed more active than its homolog (compound 7) by interacting with Ni ions, Kcx490, His492, and His519. Four additional hydrogen bonds were also observed between oxygen’s glucopyranoside of compound 8 and the key residues, including Asp494, Cme592, and Ala636 (Table 5). Compounds 6 and 9 have interacted with other residues shown in Table 5 and Figures S17- S18.
Discussion
The data obtained from phytochemical analysis of the aerial parts of O. decumbens confirmed the presence of the acylated kaempferol glycosides in the ethyl acetate and methanol fractions which were first isolated from O. decumbens. In the previous studies, acylated kaempferol glycosides were isolated and identified from two genera Foeniculum and Eryngium, belonging to the family Apiaceae (40, 41). Also, two thymol glucosides (compounds 7 and 8) isolated from ethyl acetate fraction of O. decumbens were previously reported to be isolated from Carum ajowan as a genus of family Apiaceae (35).
Totally, ethyl acetate fraction showed desired antibacterial activity, which can be associated with the presence of kaempferol glycosides (tiliroside) and various phenolic compounds in this fraction. Falcão‐Silva et al., reported that combination of isolated tiliroside from Herissantia tiubae (Malvaceae) with antibiotics such as norfloxacin, ofloxacin and ciprofloxacin led to modulated antibacterial activity and much lower MIC values (2-16 fold). It was found that the tiliroside played a significant role as a putative efflux pump inhibitor in bacteria due to the lipophilicity of the aglycon moiety (42). Also, Liang et al. showed that thymol derivatives such as hydroxythymol and thymol-β-glucopyranoside demonstrated antibacterial effect (43). It seems that thymol and kaempferol glycosides derivatives are responsible for inducing antibacterial activity of ethyl acetate fraction, which can be endorsed by the study reported by Nostro et al. on the effect of thymol on biofilm-grown S. epidermidis and S. aureus strains which emphasized the favorable activity of thymol as a lead scaffold for the desired antibacterial activity (44).
On the other hand, the n-hexane fraction of O. decumbens was the most active fraction against H. pylori that is probably due to the presence of stigmasterol and carvacrol as the most active anti-urease compounds. Among the isolated compounds, stigmasterol as one of the active compounds of the series (Table 5), with the lowest free binding energy -8.75 kcal/mol and Ki = 386.65 nM showed appropriate docking score and suitable interactions in agreement with the biological activity, suggesting that this compound could be an efficient inhibitor. Furthermore, Vazirzadeh et al. 2019 showed that the administration of different derivatives of O. decumbens had remarkable antibacterial activity against streptococcus and also enhanced antioxidant status and post-challenge immunity in Nile tilapia (45). Carvacrol adopted a similar orientation to thymol. The potency of carvacrol as the most active component (Table 4) might be attributed to the hydrogen bonding interactions between the hydroxyl group and Ala440, Kcx490, His492, Ni ions (Figure 2a). In consist with our findings, previous studies revealed that carvacrol showed anti-H. pylori activity is more significant than thymol (17, 46).
Moreover, similar to our findings, Hřibová et al. 2014, showed tiliroside inhibits urease with a docking score equal to ‐8.9 kcal/M in-silico study (46).
However, the binding mode of compounds 7 and 8 are in an almost similar way which has been investigated by the mixture of both combinations in inhibition of catalytic activities of the studied enzyme (Table 4).
The inactivity of compound 6 was clarified by the investigation of the binding mechanism. It is obvious that the inactivity of this compound largely depends on the bulky substitution in which caused poor interaction and steric clashes. The steric hindrance of groups on adjacent positions might be responsible for the contrast behavior of compound 6. This created a complete shift in the conformation and ultimately prevented interactions with the key active site residues and Ni ions (Figure S17). While in the case of tiliroside, possessing a lower number of substitutions is well oriented within the binding cavity in comparison to compound 6. Then, the importance of hydrogen bonding interaction with key residues and metal/ionic contact with Ni is also confirmed by experimental results. In addition, compounds 6 and 9 bearing bulky substitutions were found to be lower effective than tiliroside. The OH group of the neohesperidoside moiety of compound 9 mediated bidentate interactions with Ni ions as shown in Table 5, while the kaempferol part of the compound 9 presented an inert behavior which may be attributed to the spatial hindrance of some groups, resulting in moderate inhibition (Figure S18, Table 4). From the above discussion, it can be concluded that electron-rich hydroxyl groups played vital roles in the urease inhibitory activity. The hydroxyl groups of rings of all compounds except compound 6 interacted with crucial residues and Ni ions. To sum up, all desired interactions of stigmasterol, tiliroside and carvacrol with key residues of the jack bean urease (7, 20 and 47) were completely in accordance with those data from in-vitro urease inhibitory activity assay.
![Structure of the isolated compounds from different fractions of Oliveria decumbens Vent. (a) Compound 1: Octacosane, (b)Compound 2: Carvacrol, (c) Compound 3: Thymol, (d) Compound 4: Stigmasterol, (e) Compound 5: Kaempferol-3-O-(6''-O-trans-coumaryl) glucoside (Tiliroside), (f) Compound 6: Kaempferol 3 -O-(6’’-O-trans-coumaryl)glucoside 7-O-(6’’’-O-trans-coumaryl) glucoside, (g) Compound 7: 3-Hydroxythymol-6-O-D-Glucopyranoside, (h) Compound 8: 6- Hydroxythymol-3-O-D-Glucopyranoside, (i) Compound 9: Kaempferol 3-O-neohesperidoside-7-O-[2-O-(cis-feruloyl)]-D-glucopyranoside](https://services.brieflands.com/cdn/serve//315dc/7185affa34eaac00b504098db3fa744231949fb4/ijpr-20-476-g001.jpg)
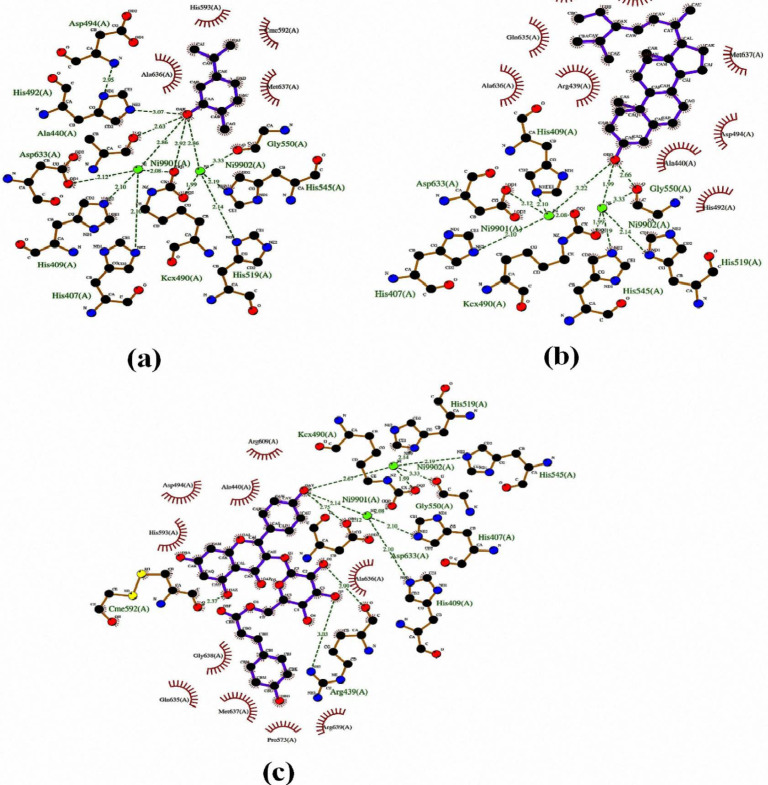
Total extract and different fractions of Oliveria decumbens Vent.: Minimum inhibitory concentrations (MIC) for the growth of Helicobacter pylori by the agar dilution method
Sample | MIC (µg/mL) |
---|---|
Total extract | > 2000 |
Hexane fraction | 50 |
Chloroform fraction | 1500 |
Ethyl acetate fraction | 1000 |
Methanol fraction | 1750 |
Amoxicillin as positive control | 0.5 |
Inhibition zone (mm) of total extract and different fractions of Oliveria decumbens Vent. and antibiotic discs against some pathogenic bacteria by the disc diffusion method
Sample | S. aureus | S. epidermidis | E. coli | P. aeruginosa |
---|---|---|---|---|
Total extract | 12 mm | NZ | NZ | NZ |
Hexane fraction (500 µg/disc) | 8 mm | NZ | NZ | NZ |
Chloroform fraction (500 µg/disc) | 12 mm | NZ | NZ | NZ |
Ethyl acetate fraction (500 µg/disc) | 20 mm | 9 mm | 10 mm | NZ |
Methanol fraction (500 µg/disc) | 16 mm | NZ | NZ | NZ |
Ciprofloxacin (5 µg/disc) | 24 mm | 36 mm | 33 mm | 32 mm |
Penicillin (10 µg/disc) | 24 mm | 26 mm | 10 | - |
Urease inhibitory of total extract and different fractions of Oliveria decumbens Vent. as IC50 (µg /mL).
Sample | IC50(µg/mL) ± SD |
---|---|
Total | > 1000 |
Hexane | 285.44 ± 0.01 |
Chloroform | 375.36 ± 0.02 |
Ethyl acetate | 285.06 ± 0.02 |
Methanol | 709.42 ± 0.03 |
Hydroxyurea | 59.51 ± 0.01 |
Urease inhibitory of the isolated compounds of Oliveria decumbens aerial parts as IC50 (mM)
Compounds | IC50 (mM) ± SD |
---|---|
Thymol | 1.33 ± 0.06 |
Carvacrol | 0.70 ± 0.01 |
Stigmasterol | ± 0.010.27 |
Tiliroside | 0.45± 0.05 |
The mixture of hydroxyl thymol glucoside compounds | * |
Kaempferol-3-O-(6''-O-trans-coumaroyl) glucopyranoside 7-O- 6''''' coumaroyl glucopyranoside | N |
Kaempferol 3-Oβ -O-neohesperidoside-7-O-[2-O-(cis-feruloyl)] β -D-glucopyranoside | * |
Hydroxyurea | 0.78± 0.01 |
Energy-based interactions detail of the identified structures isolated from Oliveria decumbens as the urease inhibitors
Ligand No | Docking Score | Estimated Inhibition Constant (Ki) | Interactions detail | Distance (Å) | ||
---|---|---|---|---|---|---|
Ligand | Receptor | |||||
Thymol | -4.44 | 553.48 µM | OAK | His492 | NE2 | 2.80 |
Carvacrol | -4.88 | 263.60 µM | OAK | Ala440 | O | 2.63 |
Stigmasterol | -8.75 | 386.65 nM | OBD | Gly550 | O | 2.66 |
Tiliroside | -8.49 | 598.78 nM | O3 | Arg439 | NH2 | 3.03 |
3-Hydroxythymol-6-O-D-Glucopyranoside | -6.52 | 16.69 µM | O3 | Arg439 | O | 3.19 |
6- Hydroxythymol-3-O-D-Glucopyranoside | -6.11 | 33.31 µM | OAO | Kcx490 | OQ1 | 3.07 |
Kaempferol-3-O-(6''-O-trans-coumaroyl) glucopyranoside 7-O- 6' coumaroyl glucopyranoside | -6.11 | 33.28 µM | OBE | Glu493 | OE1 | 2.65 |
Kaempferol 3-Oβ -O-neohesperidoside-7-O-[2-O-(cis-feruloyl)] β -D-glucopyranoside | -1.60 | 67.38 mM | OAV | Asp494 | OD1 | 2.87 |
Conclusion
In conclusion, a phytochemical analysis of O. decumbens was developed to identify new derivatives of kaempferol and thymol, which were not reported previously. Also, the results obtained from the evaluation of different fractions of the plant toward H. pylori, various strains of bacteria as well as anti-urease activity of isolated compounds demonstrated satisfactory biological properties of the plant associated with gastrointestinal disease.
Conflict of interest
The authors declare that they have no conflict of interest.
Author contributions
Study concept and design: Mahnaz Khanavi, Mohammad Reza Shams Ardekani and Mahdieh Eftekhari; Phytochemical investigation: Mahdieh Eftekhari, Mahnaz Khanavi; Biological activities: Mahdieh Eftekhari, Mohsen Amin, Docking analysis: Mahboubeh Mansourian; Interpretation of data: Mahdieh Eftekhari, Tahmineh Akbarzadeh and Mina Saeedi; Drafting of the manuscript: Mahdieh Eftekhari, Mina Saeedi, Mahnaz Khanavi.
Acknowledgements
References
-
1.
Mahady G. Medicinal plants for the prevention and treatment of bacterial infections. Curr. Pharm. Des. 2005;11:2405-27. [PubMed ID: 16026296].
-
2.
Lawrence RS, Durch JS, Stratton KR. Vaccines for the 21st Century: A Tool For Decisionmaking. National Academies Press, Washington (D.C.); 2001. 25121214 p.
-
3.
Hassan ST, Šudomová M. The development of urease inhibitors: what opportunities exist for better treatment of Helicobacter pylori infection in children? Children. 2017;4:2.
-
4.
Valgas C, Souza SMd, Smânia EF, Smânia Jr A. Screening methods to determine antibacterial activity of natural products. Braz. J. Microbiol. 2007;38:369-80.
-
5.
Nascimento GG, Locatelli J, Freitas PC, Silva GL. Antibacterial activity of plant extracts and phytochemicals on antibiotic-resistant bacteria. Braz. J. Microbiol. 2000;31:247-56.
-
6.
Parreira P, Fátima Duarte M, Reis CA, Martins MCL. Helicobacter pylori infection: a brief overview on alternative natural treatments to conventional therapy. Crit. Rev. Microbiol. 2016;42:94-105. [PubMed ID: 24606042].
-
7.
Grochowski DM, Locatelli M, Granica S, Cacciagrano F, Tomczyk M. A review on the dietary flavonoid tiliroside. Compr. Rev. Food Sci. Food Saf. 2018;17:1395-421. [PubMed ID: 33350157].
-
8.
Xiao ZP, Wang XD, Peng ZY, Huang S, Yang P, Li QS, Zhou LH, Hu XJ, Wu LJ, Zhou Y, Zhu HL. Molecular docking, kinetics study, and structure–activity relationship analysis of quercetin and its analogous as Helicobacter pylori urease inhibitors. J. Agric. Food Chem. 2012;60:10572-7. [PubMed ID: 23067328].
-
9.
Zhang L, Kong Y, Wu D, Zhang H, Wu J, Chen J, Ding J, Hu L, Jiang H, Shen X. Three flavonoids targeting the β‐hydroxyacyl‐acyl carrier protein dehydratase from Helicobacter pylori: Crystal structure characterization with enzymatic inhibition assay. Protein Sci. 2008;17:1971-8. [PubMed ID: 18780820].
-
10.
Xie Y, Yang W, Tang F, Chen X, Ren L. Antibacterial activities of flavonoids: structure-activity relationship and mechanism. Curr. Med. Chem. 2015;22:132-49. [PubMed ID: 25245513].
-
11.
Escandón RA, Del Campo M, López-Solis R, Obreque-Slier E, Toledo H. Antibacterial effect of kaempferol and (−)-epicatechin on Helicobacter pylori. Eur. Food Res. Technol. 2016;242:1495-502.
-
12.
Gebhardt Y, Witte S, Forkmann G, Lukačin R, Matern U, Martens S. Molecular evolution of flavonoid dioxygenases in the family Apiaceae. Phytochemistry. 2005;66:1273-84. [PubMed ID: 15913674].
-
13.
Eftekhari M, Ardekani MS, Khanavi M, Amini M. The effect of collection time on essential oil of Oliveria decumbens Vent. Res. J. Pharmacogn. 2017;4:89.
-
14.
Mosaddegh M, Naghibi F, Moazzeni H, Pirani A, Esmaeili S. Ethnobotanical survey of herbal remedies traditionally used in Kohghiluyeh va Boyer Ahmad province of Iran. J. Ethnopharmacol. 2012;141:80-95. [PubMed ID: 22366675].
-
15.
Mahboubi M, Feizabadi M. Antifungal activity of essential oil from Oliveria decumbens Vent and its synergy with amphotricin B. Int. J. Essential Oil Therap. 2008;2:26-8.
-
16.
Moghadam MS, Maleki S, Darabpour E, Motamedi H, Nejad SMS. Antibacterial activity of eight Iranian plant extracts against methicillin and cefixime restistant Staphylococcous aureus strains. Asian Pac. J. Trop. Med. 2010;3:262-5.
-
17.
Eftekhari M, Ardekani S, Reza M, Attar F, Akbarzadeh T, Safavi M, Karimpour-Razkenari E, Amini M, Isman M, Khanavi M. Oliveria decumbens, a bioactive essential oil: Chemical composition and biological activities. Iran. J. Pharm. Res. 2019;18:412-21. [PubMed ID: 31089376].
-
18.
Tanaka T, Kawase M, Tani S. α-Hydroxyketones as inhibitors of urease. Bioorg. Med. Chem. 2004;12:501-5. [PubMed ID: 14723968].
-
19.
Morris GM, Huey R, Lindstrom W, Sanner MF, Belew RK, Goodsell DS and Olson AJ. AutoDock4 and AutoDockTools4: Automated docking with selective receptor flexibility. J. Comput. Chem. 2009;30:2785-91. [PubMed ID: 19399780].
-
20.
Ali B, Khan KM, Hussain S, Hussain S, Ashraf M, Riaz M, Wadood A, Perveen S. Synthetic nicotinic/isonicotinic thiosemicarbazides: In-vitro urease inhibitory activities and molecular docking studies. Bioorg. Chem. 2018;79:34-45. [PubMed ID: 29723741].
-
21.
Mansourian M, Saghaie L, Fassihi A, Madadkar-Sobhani A, Mahnam K. Linear and nonlinear QSAR modeling of 1, 3, 8-substituted-9-deazaxanthines as potential selective A 2B AR antagonists. Med. Chem. Res. 2013;22:4549-67.
-
22.
Bano B, Khan KM, Begum F, Lodhi MA, Salar U, Khalil R, Ul-Haq Z, Perveen S. Benzylidine indane-1, 3-diones: As novel urease inhibitors; synthesis, in-vitro, and in-silico studies. Bioorg. Chem. 2018;81:658-71. [PubMed ID: 30253339].
-
23.
Morris GM, Goodsell DS, Halliday RS, Huey R, Hart WE, Belew RK, Olson AJ. Automated docking using a Lamarckian genetic algorithm and an empirical binding free energy function. J. Comput. Chem. 1998;19:1639-62.
-
24.
Nasab RR, Hassanzadeh F, Khodarahmi GA, Rostami M, Mirzaei M, Jahanian-Najafabadi A, Mansourian M. Docking study, synthesis and antimicrobial evaluation of some novel 4-anilinoquinazoline derivatives. Res. Pharm. Sci. 2017;12:425. [PubMed ID: 28974981].
-
25.
Wallace AC, Laskowski RA, Thornton JM. LIGPLOT: a program to generate schematic diagrams of protein-ligand interactions. Protein Eng. Des. Sel. 1995;8:127-34.
-
26.
Rajkumar S, Jebanesan A. Mosquitocidal activities of octacosane from Moschosma polystachyum Linn (lamiaceae). J. Ethnopharmacol. 2004;90:87-9. [PubMed ID: 14698514].
-
27.
Rahuman AA, Gopalakrishnan G, Venkatesan P, Geetha K, Bagavan A. Mosquito larvicidal activity of isolated compounds from the rhizome of Zingiber officinale. Phytother. Res. 2008;22:1035-9. [PubMed ID: 18618523].
-
28.
Tang X, Chen S, Wang L. Purification and identification of carvacrol from the root of Stellera chamaejasme and research on its insecticidal activity. Nat. Prod. Res. 2011;25:320-5. [PubMed ID: 21294044].
-
29.
Mulinacci N, Melani F, Vincieri F, Mazzi G, Romani A. 1H-NMR NOE and molecular modelling to characterize thymol and carvacrol β-cyclodextrin complexes. Int. J. Pharm. 1996;128:81-8.
-
30.
Pierre LL, Moses MN. Isolation and characterisation of stigmasterol and β-sitosterol from Odontonema strictum (acanthaceae). J. Innov. Pharm. Biol. Sci. 2015;2:88-96.
-
31.
Qin N, Li CB, Jin MN, Shi LH, Duan HQ, Niu WY. Synthesis and biological activity of novel tiliroside derivants. Eur. J. Med. Chem. 2011;46:5189-95. [PubMed ID: 21856048].
-
32.
Vermes B, Chari VM, Wagner H. Structure elucidation and synthesis of Flavonol Acylglycosides The synthesis of tiliroside. Helvetica. Chimica. Acta. 1981;64:1964-7.
-
33.
Raturi R, Sati S, Singh H, Sati M, Badoni P. Phytochemical Examination of Anaphalis Busua Leaves. In: Khemani L, Srivastava M, Srivastava S, editors. . Chemistry of Phytopotentials: Health, Energy and Environmental Perspectives. Springer, Berlin, Heidelberg; 2012. p. 105-6.
-
34.
Agrawal PK. Carbon-13 NMR of flavonoids. 1st Ed. New York; 1989. 497 p.
-
35.
Ishikawa T, Sega Y, Kitajima J. Water-soluble constituents of ajowan. Chem. Pharm. Bull. 2001;49:840-4.
-
36.
Kamel M, Assaf M, Hasanean H, Ohtani K, Kasai R, Yamasaki K. Monoterpene glucosides from Origanum syriacum. Phytochem. 2001;58:1149-52.
-
37.
Kumar TVS, Sankar K-U, Divakar S. Synthesis of thymol glycosides under SCCO 2 conditions using amyloglucosidase from Rhizopus mold. J. Food Sci. Technol. 2013;50:803-8. [PubMed ID: 24425985].
-
38.
Carotenuto A, De Feo V, Fattorusso E, Lanzotti V, Magno S, Cicala C. The flavonoids of Allium ursinum. Phytochem. 1996;41:531-6.
-
39.
Selyutina IY, Tankhaeva L, Olennikov D. Acylated kaempferol diglycoside from Allium senescens. Chem. Nat. Compd. 2008;44:769-70.
-
40.
Soliman FM, Shehata AH, Khaleel AE, Ezzat SM. An acylated kaempferol glycoside from flowers of Foeniculum vulgare and F. Dulce. Molecules. 2002;7:245-51.
-
41.
Hohmann J, Pall Z, Günther G, Mathe I. Flavonolacyl glycosides of the aerial parts of Eryngium campestre. Planta med. 1997;63:96.
-
42.
Falcão‐Silva VS, Silva DA, Souza MdFV, Siqueira‐Junior JP. Modulation of drug resistance in Staphylococcus aureus by a kaempferol glycoside from Herissantia tiubae (Malvaceae). Phytother. Res.: An International Journal Devoted to Pharmacological and Toxicological Evaluation of Natural Product Derivatives. 2009;23:1367-70.
-
43.
Liang H, Bao F, Dong X, Tan R, Zhang C, Lu Q, Cheng Y. Antibacterial thymol derivatives isolated from Centipeda minima. Molecules. 2007;12:1606-13. [PubMed ID: 17960076].
-
44.
Nostro A, Roccaro AS, Bisignano G, Marino A, Cannatelli MA, Pizzimenti FC, Cioni PL, Procopio F, Blanco AR. Effects of oregano, carvacrol and thymol on Staphylococcus aureus and Staphylococcus epidermidis biofilms. J. Med. Microbiol. 2007;56:519-23. [PubMed ID: 17374894].
-
45.
Vazirzadeh A, Jalali S, Farhadi A. Antibacterial activity of Oliveria decumbens against Streptococcus iniae in Nile tilapia (Oreochromis niloticus) and its effects on serum and mucosal immunity and antioxidant status. Fish Shellfish Immunol. 2019;94:407-16. [PubMed ID: 31521784].
-
46.
Hřibová P, Khazneh E, Žemlička M, Švajdlenka E, Ghoneim MM, Elokely KM, Ross SA. Antiurease activity of plants growing in the Czech Republic. Nat. Prod. Res. 2014;28:868-73. [PubMed ID: 24579848].
-
47.
Taha M, Ullah H, Al Muqarrabun LMR, Khan MN, Rahim F, Ahmat N, Javid MT, Ali M, Khan KM. Bisindolylmethane thiosemicarbazides as potential inhibitors of urease: Synthesis and molecular modeling studies. Bioorg. Med. Chem. 2018;26:152-60. [PubMed ID: 29183662].