Abstract
Background:
The venom of Echis carinatus contains both procoagulant and anticoagulant components that can either promote or block the blood coagulation cascade, and some of these components affect platelet function in different ways.Objectives:
The present study focuses on setting up a procedure for the purification of crude venom and designing appropriate clotting tests in order to characterize the procoagulant and anticoagulant fractions of E. carinatus venom.Methods:
Chromatographic methods, including gel filtration, ion-exchange chromatography, and reverse-phase high-performance liquid chromatography (HPLC), were applied for purifying these fractions. Coagulant activity testing, prothrombin time (PT), and activated partial thromboplastin time (APTT) were used to determine procoagulant and anticoagulant properties. For measuring molecular weight, 15% SDS-PAGE electrophoresis with a molecular weight standard ranging from 6.5 to 200 kDa was used.Results:
We obtained five fractions named F1, F2, F3, F4, and F5. The F1 and F2 fractions showed procoagulant activity, and the F5 fraction had anticoagulant activity. The molecular weight of F2.4.2 from fraction F2 and F5.1 from fraction F5 were analyzed by SDS-PAGE electrophoresis under the reducing condition. These factors were identified as a single protein band at the end of purification. The molecular weights of these purified fractions were estimated to be 7.5 kDa and 38 kDa for F5.1(b) and F2.4.2(b), respectively.Conclusions:
Our findings suggest an efficient and suitable procedure for the identification and purification of the procoagulant and anticoagulant factors of the venom of Iranian E. carinatus using the PT and APTT assays.Keywords
1. Background
Among the most important and main components of snake venom are proteins (70 - 90%). The chemical constituents of snake venom could be classified into two non-protein and protein components. The protein component is further subdivided into enzymatic and non-enzymatic proteins. The non-enzymatic proteins of snake venoms include neurotoxin, cardiotoxin, myotoxin, cytotoxin, 3FTXs, cysteine-rich secretory protein (CRISP), Kunitz-type serine protease inhibitors, sarafotoxins, disintegrins, waprins, C-type lectins, veficolins, and vespryns (1-4). Enzymatic proteins include phospholipase A2, phosphodiesterase, acetylcholinesterase, hyaluronidase, hemorrhagic metalloproteinases, proteolytic enzymes, heparinase, arginine ester hydrolase, RNase, 5-nucleotidase, lactate dehydrogenase, phosphomonoesterase, thrombin-like enzyme, phospholipase B, phospholipase C, phosphodiesterase, L-amino acid oxidase, and proteolytic enzymes such as serine proteases and metalloproteinases (4-7). The venom composition of snakes varies from species to species, their geographical distribution, living conditions, and season, contributing to the clinical variations and unpredictability of snakebites and envenomation. Venomous snake families are classified into four categories: Atractaspididae, Elapidae, Colubridae, and Viperidae, whose venoms contain shared protein families. Although the venom of these families contains some homologous enzymes, the venom of each family has distinct and exclusive biological and biochemical properties caused by differences in amino acid sequences and relative abundance of related proteins (8-10). The venoms of Viperidae snakes are rich in proteins that target the hemostatic system and blood coagulation cascade via either promoting or inhibiting one of the three mutually exclusive functional processes: Platelet aggregation, blood coagulation (anticoagulation or pro-coagulation), and fibrinolysis (11-15).
The venom of Iranian Echis carinatus (IEc), belonging to the Viperidae family, is rich in proteins and peptides that are effective in the hemostatic system. These proteins and peptides include procoagulant, anticoagulant, and fibrinolytic factors affecting the intrinsic, common, and extrinsic paths of the coagulation cascade (15-17).
2. Objectives
In the present study, both the procoagulant and anticoagulant properties of endemic IEc’s venom and its fractions were investigated to identify fractions with procoagulant and anticoagulant activities.
3. Methods
3.1. Biological Material and Venom Extraction
Iranian IEc’s lyophilized crude venom was obtained from Venomous Animals and Antivenom Production Department, Razi Vaccine and Serum Research Institute, Karaj, Iran.
3.2. Chemicals
Tris-buffer, bovine serum albumin (BSA), acetonitrile (high-performance liquid chromatography (HPLC) grade), water (HPLC grade), calcium chloride (CaCl2), Sephadex G-50, cepharose, ammonium acetate, TFA, foline, DEAE-sepharose, CM-cepharose and all other chemicals and reagents used in this study were of analytical grade and purchased from Merck (Darmstadt, Germany). CaCl2, prothrombin time (PT) reagent, and PTT reagent were purchased from Fisher Diagnostics (USA).
3.3. Protein Determination
Lowry et al.’s method was used to determine protein concentration. BSA was used as a standard (18).
3.4. LD50
The LD50 was calculated by the Reed & Munch method according to WHO guidelines (Iran National Committee for Ethics in biomedical research, Shahid Beheshti University of Medical Sciences) (19, 20).
3.5. Sodium Dodecyl Sulfate-Polyacrylamide Gel Electrophoresis
The molecular weight and homogeneity of purified venom fractions and crude venom were examined by 20% and 15% SDS-PAGE electrophoresis, based on the method described by Laemmli (21).
3.6. Preparation of Citrated Human Plasma
Blood samples were obtained from healthy human volunteers (20 - 30 years old) and were immediately mixed with anticoagulant tri-sodium citrate 3.2% (9:1 v/v). Then it was centrifuged at 3000 g for 20 min at 4°C to obtain poor-platelet plasma (PPP) for coagulation studies: PT, PTT, and coagulant activity.
3.7. Hemostatic Assays
3.7.1. Coagulant Activity
Briefly, normal plasma (PPP) was incubated at 37°C for one min; then, sample aliquots containing specified concentrations of crude venom/fractions/subfractions were added, mixed, and shaken. The time elapsed to the appearance of the first clot was recorded.
3.7.2. PT and Activated Partial Thromboplastin Time Tests
The hemostatic effects of IEc venom and its purified fractions on the blood coagulation cascade were evaluated using the hemostatic tests of PT and activated partial thromboplastin time (APTT) according to the manufacturer’s instructions (Catalog No. 17-609-9 and 02-292-7.79718D0395, respectively) (Fisher Diagnostics, USA) (22, 23).
3.8. Purification Protocol
3.8.1. Crude Venom Fractionation
Lyophilized crude venom of IEc (100 mg) was dissolved in 3 mL of 0.05 M ammonium acetate buffer (pH 7.4) and then centrifuged at 14,000 g for 20 min at four °C and filtered through 0.2 a nylon syringe microfilter to wash out all insoluble materials (Sigma laboratory centrifuges 6k15,10680). The cleared venom filtrate was loaded onto a gel chromatographic column (150 x 2 cm, Sephadex G-50) pre-equilibrated with ammonium acetate buffer (0.05 M, pH 7.4) and then eluted with the same buffer. Fractions (5 mL/tube) were collected at a flow rate of 30 mL/hr at four °C using a Fraction Collector (Pharmacia Biotech, RediFrac Fraction Collector). The elution profile was monitored at 280 nm by a spectrophotometer (Biochrom Libra S22 UV/Vis Spectrophotometer 60-2115-20). The E. carinatus fractions obtained were denominated as F1 to F5 and assayed for clotting activity, as well as anticoagulant and procoagulant capacities.
3.9. Ion-Exchange Chromatography
3.9.1. Anion -Exchange Chromatography
Specific amounts of fraction F2 and F5 (7.5 mL of F2 and 5 mL of F5) were concentrated by ultrafiltration (UF) and dissolved in 7.5 and 5mL of 20 mM Tris-base buffer (pH 8.2), respectively. The solutions were loaded continually onto a DEAE-sepharose column (20 * 1.6 cm) at a flow rate of 30 mL/h equilibrated in 20 mM Tris-base buffer (pH 8.2) and washed with the same buffer containing 0.2 M NaCl. The output solution of anion exchange chromatography (5 mL/tube) was collected by an automatic collector (Pharmacia Biotech RediFrac Fraction Collector) at the flow rate of 30 mL/h for 24 h at a linear gradient of 0 - 1 M of NaCl. The effluent was monitored at 280 nm by a spectrophotometer (Biochrom Libra S22 UV/Vis Spectrophotometer 60-2115-20) (24, 25). The fractions obtained from F2 (F2.1, F2.2, F2.3, and F2.4) and F5 (F5.1) were analyzed for clotting time and procoagulant and anticoagulant activity with regard to human plasma.
3.9.2. Cation Exchange Chromatography
The sub-fractions F2.4 (from F2) and F5.1 (from F5) were selected for further purification. A specified amount (3.5 mL of F2.4) of fraction F2.4 was dissolved in 3.5 mL of 20 mM sodium acetate buffer (pH 5.7) after concentration by ultrafiltration, loaded continually onto a CM-sepharose column (20 * 1 cm) at a flow rate of 15 mL/h equilibrated in 20 mM sodium acetate buffer (pH 5.7), and washed with the same buffer containing 0.2 M NaCl. The output solution of cation exchange chromatography (3.5 mL/tube) was collected by an automatic collector at a flow rate of 15 mL/h for 24 h at a linear gradient of 0 - 0.8 M of NaCl. The effluent was monitored at 280 nm by a spectrophotometer.
3.9.3. Reversed-Phase High-Performance Liquid Chromatography
The fractions exhibiting procoagulant (F2.4.2) and anticoagulant (F5.1) activities in the previous step were pooled and cconcentrated by ultrafiltration. The supernatant was filtered on a 0.02 microfilter and then was applied into a reversed-phase high-performance liquid chromatography (RP-HPLC) column (HPLC, Shimadzu high-performance liquid chromatography) (C4 for F2.4.2 and C18 for F5.1, five μm, 9.4 × 250 mm Column, Agilent Inc., USA). Elution was carried out at a linear gradient of H2O containing 0.1% (v/v) trifluoroacetic acid (solvent A) and acetonitrile containing 0.1% trifluoroacetic acid (solvent B) at a flow rate of 0.5 mL/minute. The peaks were monitored at 280 and 215 nm.
4. Results
Table 1 and Table 2 show the biological and hematological properties of the crude venom.
Biological Properties of the Crude Venom of Echis carinatus
Sample | Concentration of Proteins | LD50 |
---|---|---|
Venom | 94% | 11.311 µg/mice |
Hematological Properties of Different Concentrations of Echis carinatus Venom
Sample | Concentration (mg/mL) | PT (s) | PTT (s) |
---|---|---|---|
Venom | 1 | 7 | 12 |
Venom | 0.1 | 10 | 23.70 |
Venom | 0.01 | 12 | 34 |
Control | - | 10.11 | 31.9 |
4.1. Isolation and Purification
In the Sephadex G-50 fractionation of the crude venom, five peaks were identified (Figure 1). The obtained fractions were denominated as F1 to F5, indicating E. carinatus fractions 1 to 5, respectively. The fractions and subfractions were assayed for clotting time, as well as anticoagulant and procoagulant capacities with regard to human plasma (Table 3 and Figure 2). Further purification was carried out by ion-exchange chromatography on DEAE-sepharose and CM-sepharose following HPLC chromatography (Figures 3, 4, 5, 6, and 7).
Sephadex G-50 chromatography of the venom of Iranian snake, Echis carinatus.
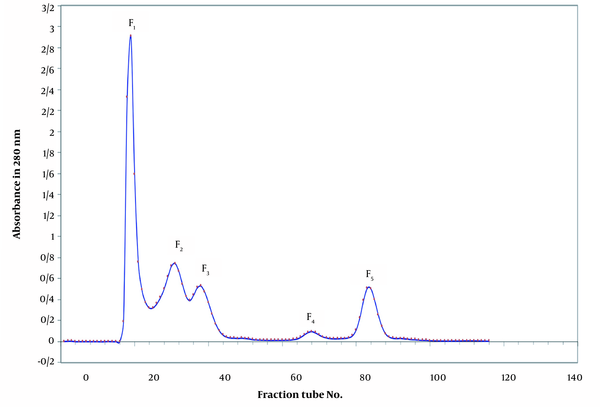
Hematologic Properties of the Crude Venom and Venom Fractions of IEc Obtained from Gel Filtration a
Sample | Protein, (µg/mL) | Clotting Time (s) | PT (s) | PTT (s) |
---|---|---|---|---|
Venom, 1 (mg/mL) | 1 (mg/mL) | 6.20b | 7c | 12d |
F1 | 933 | 4.52 | 6.74 | 11 |
F2 | 470 | 14 | 9 | 28 |
F3 | 313 | 33.95 | 10.24 | 26.9 |
F4 | 28 | 80 | 9.925 | 30.7 |
F5 | 338 | No clot | 20 | 80 |
Control | - | No clote | 10.11f | 31.9g |
F2.1 | 13 | 39 | 14 | 34 |
F2.2 | 15 | 39 | 13 | 35 |
F2.3 | 14 | 40 | 12 | 34 |
F2.4 | 55 | 25 | 8 | 28 |
F5.1 | 112 | No clot | 23 | 80 |
Control | No clot | 10.11 | 31.9 | |
F2.4.1 | 12 | No clot | 15 | 40 |
F2.4.2 | 45 | 25 | 9 | 28 |
F2.4.3 | 12 | 40 | 15 | 40 |
F2.4.4 | 8 | No clot | 15 | 40 |
F2.4.5 | 9 | No clot | 20 | 40 |
Control | No clot | 10.11 | 31.9 | |
F2.4.2(a) | 15 | No clot | 12 | 34 |
F2.4.2(b) | 40 | 25 | 9 | 28 |
F2.4.2(c) | 10 | No clot | 13 | 35 |
F5.1(a) | 8 | No clot | 16 | 60 |
F5.1(b) | 52 | No clot | 26 | 60 |
Control | No clot | 10.11 | 31.9 |
The clotting activity of fraction F2. The results of the clotting time for different concentrations of fraction F2 (Spt.d)
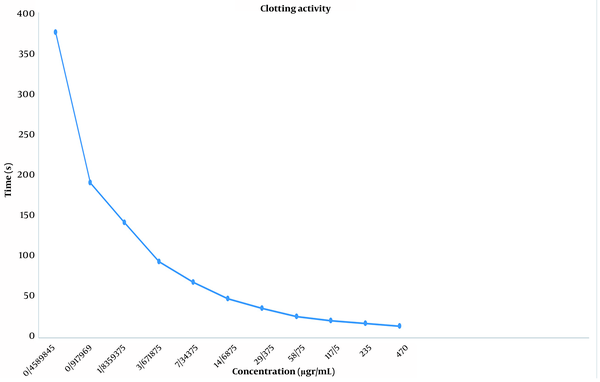
Purification of the F2 fraction by DEAE-sepharose chromatography. Ion-exchange chromatography profile (Spt.d)
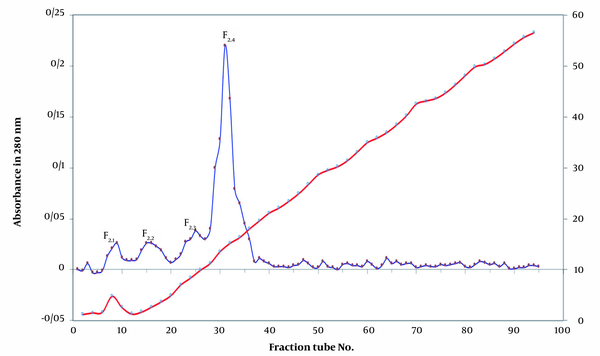
Purification of the F5 fraction by DEAE-sepharose chromatography. Ion-exchange chromatography profile (Spt.d)
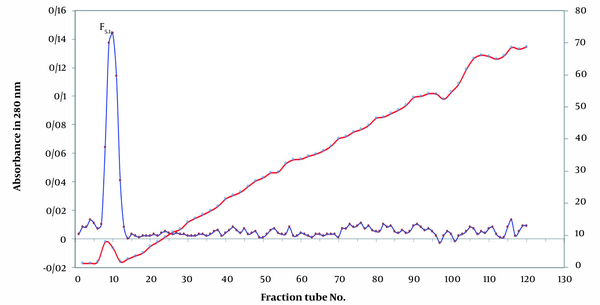
Purification of the F2.4 sub-fraction by CM-sepharose chromatography. Ion-exchange chromatography profile (Spt.d)
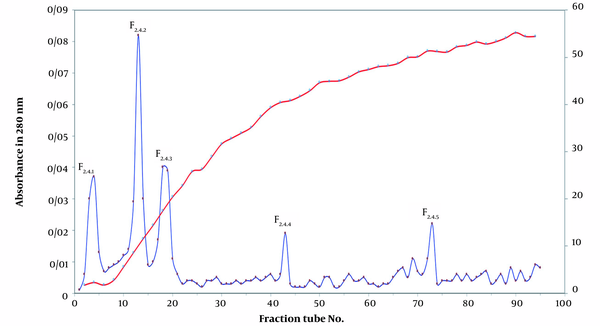
Further purification of sub-fraction F5.1. HPLC chromatography of the F5.1 fraction obtained from DEAE-sepharose chromatography (Spt.d)
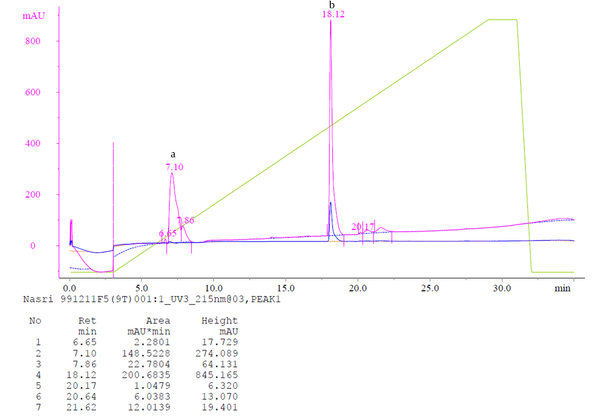
Further purification of the sub-fraction F2.4.2. HPLC chromatography of the F2.4.2 fraction obtained from CM-sepharose chromatography (Spt.d)
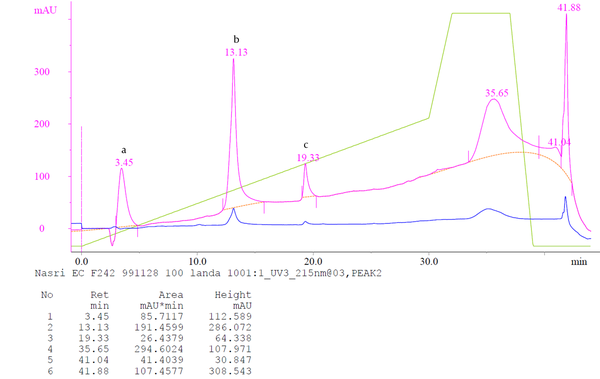
In size-exclusion chromatography for purification, a 92% recovery rate was obtained.
Figure 8 shows the molecular weight pattern of the fractions extracted from the gel filtration column.
15% SDS-PAGE profile of the crud venom and venom fractions of IEc venom obtained from gel filtration
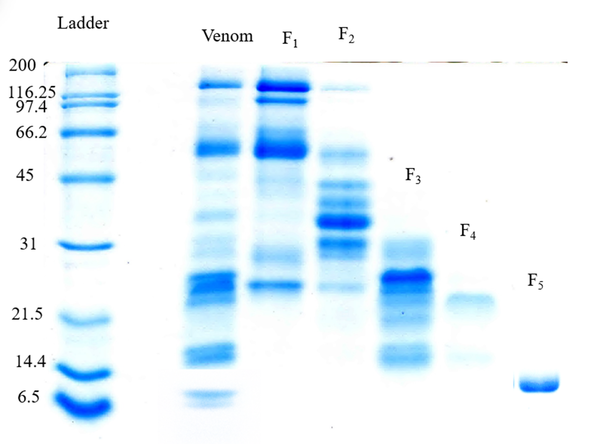
Fractions F2 and F5 were selected as procoagulant and anticoagulant fractions, respectively, and subjected to more purification.
5. Discussion
Studies have shown that toxins from snake venoms, especially the Viperidae family, affect different targets in the hemostatic system, inducing coagulopathy and hemorrhage because of the actions of toxins such as clotting factor inhibitors and activators, toxins affecting platelets and fibrinolysis, and hemorrhage that disrupts the endothelium. A number of these factors interact with the components of the human blood coagulation system. The factors that demonstrate coagulative effects are classified into two separate groups: Anticoagulants and procoagulants. The members of the recent group act as agonists and behave similar to a natural factor or activator, whereas others act as antagonists and interfere with the function of a natural factor or with the progression of a specific step of the coagulation cascade (26-29). Research on snake venoms that affect blood coagulation is important in understanding the mechanisms of activation and inhibition of blood clotting factors. Moreover, snake venoms with such activities can be beneficial as therapeutic agents. A number of procoagulant toxins from snake venoms, especially from the Viperidae family, have been used as diagnostic tools and therapeutic agents for disorders that involve unbalanced hemostasis, such as myocardial infarction, deep vein thrombosis, priapism, stroke, and sickle cell crises (30). Viprinex and Defibrase are two valuable examples of SVSP-based medical products. Defibrase® (a commercial name), also known as batroxobin, is a thrombin-like enzyme used for measuring fibrinogen levels to detect deficiencies or abnormalities in fibrinogen. Therefore, these products can help inhibit thrombogenesis and prevent thrombotic diseases. In an assay known as Reptilase™ time, a procoagulant SVSP-based agent is derived from Bothrops atrox moojeni venom (31-33). Viprinex® (also called Ancrod or commercially named Arvin) with de-fibrinogenating activity has been assayed for treating acute ischemic stroke. This thrombin-like serine protease is isolated from the venom of Malayan pit viper snake (Agkistrodon rhodostoma or Calloselasma rhodostoma) (32, 33). It is used to treat patients suffering from stroke, deep vein thrombosis, myocardial infarction, peripheral arterial thrombosis, priapism, and sickle cell crises (32-34). RVV-X (from D. russelli (Russell's viper (Daboia russelii)) venom) is beneficial for measuring lupus anticoagulants (35). Identification of new factors surely could open new perspectives in the development of various new therapeutic agents for treating cardiovascular, hematological, thrombosis, and hemostatic disorders. On the other hand, envenomation by snake bites, especially by Echis family snake vipers (E. carinatus, E. coloratus, E. ocellatus, and E. pyramidum) can cause severe coagulopathy with hard-to-manage signs and symptoms. Further, thrombin-like enzymes, unlike human thrombin, cannot be inhibited by heparin, the traditional treatment for thrombotic coagulopathies. Due to the widespread geographic distribution of IEc (Saw scaled viper), possessing a potentially fatal venom, the present study aimed to evaluate this crude venom and the effects of its fractions on hematological parameters (24).
Choudhury et al. isolated and characterized EC-PΙII, a dimeric procoagulant protein (110 kDa), and a novel p-III class of non-hemorrhagic procoagulant SVMP metalloproteinases extracted from Indian Ec venom using a combination of gel-filtration column and anion-exchange (sepharose Q column) chromatography. They evaluated its blood coagulant activity using the re-calcification time assay. Peptide mass fingerprinting confirmed that the fraction obtained had high homology with the other members of the SVMP family (25). Mehdizadeh Kashani et al. investigated the anti-platelet activity of venoms from two Iranian vipers, Ec and Cerastes persicus Fieldi. They isolated two potent platelet aggregation inhibitors from C. persicus fieldi by gel filtration using Sephadex G-100 column chromatography but suggested further studies to understand the mechanistic actions of these factors and decipher their diverse clinical and hematological manifestations (36). Babaie et al. isolated a procoagulant factor (prothrombin activator) from the venom of IEc using gel filtration (Sephadex G-75), ion-exchange chromatography (DEAE-sepharose), and reverse-phase HPLC and confirmed its procoagulant activity by the PT test and coagulant activity assay (37). In another study, three fractions were identified by gel chromatography (Sephadex G-75) and purified using ion-exchange chromatography (38). The anticoagulant sub-fractions were then intravenously injected into mice. Blood samples were taken before and after the injection of these sub-fractions, and PT, PTT, and FT were recorded. According to changes in PT and PTT and compared with the control, the rate of blood coagulation and activation of the extrinsic and intrinsic pathways considerably decreased (37). Amrollahi Byoki and Zare Mirakabadi revealed the anticoagulation activity of the crude venom, as well as its fractions and purified peptides using the PT and TT tests. A purified anticoagulant factor (EC217), identified as a single protein band in SDS-PAGE electrophoresis under reducing conditions, was also identified in the recent study (39). In the present study, lyophilized crude venom was applied to the gel chromatographic column, resulting in the appearance of five fractions (named F1, F2, F3, F4, and F5). The fractions were analyzed for blood clotting activity, of which the F2.4.2 factor from the F2 fraction and F5.1 factor from the F5 fraction were applied to the HPLC column. The final fractions were evaluated by coagulation tests. The molecular weights of the purified fractions of F5.1(b) and F2.4.2(b) were estimated to be 7.5 and 38 kDa, respectively (Figure 9). Procoagulant proteins are either serine proteinases or metalloproteinases, and their sizes vary between 24 and 300 kDa. Snake venom toxins that prolong blood coagulation are proteins or glycoproteins with molecular masses ranging from 6 to 350 kDa. These factors inhibit blood coagulation by different mechanisms. Our study presented an efficient and simple procedure for the identification and purification of procoagulant and anticoagulant factors from IEc venom, as shown by the PT and APTT tests.
SDS-PAGE electrophoresis (20%) of the F2.4.2(b) and F5.1(b) fractions of Iranian Echis carinatus venom obtained from HPLC (Spt.d)
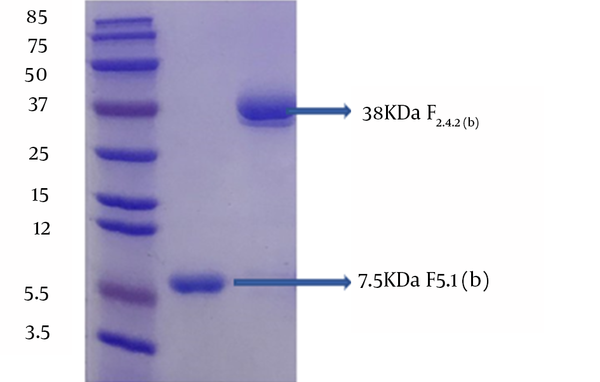
5.1. Conclusions
Procoagulant proteins from snake venom include factor VII activators, prothrombin activators, factor X activators, factor V activators, and thrombin-like enzymes, most of which require cofactors such as Ca2+ ions, phospholipids, or activated factor V (i.e., Va). Prothrombin activators are divided into four groups: A, B, C, and D. Pro-coagulant and anticoagulant factors are common in the venoms of the snakes of the Viperidae family. This study and others suggest that snake venoms with procoagulant activity may contain one or more of the prothrombin activators mentioned above (40). Group A prothrombin activators are metalloproteinases that efficiently activate prothrombin without the need for any cofactor, such as Ca2+ ions, phospholipids, or factor Va (41). They are widely distributed in many viper venoms. The best-known example is Ecarin (55 kDa) in the venom of the saw-scale viper Ec, which is a single-chain protease composing of a metalloproteinase catalytic domain, a disintegrin-like domain, and a cysteine-rich domain. For coagulation testing, we used citrated plasma (sodium citrate is a chelating agent for calcium ions) and showed that citrate plasma coagulated after the addition of the F2.4.2 fraction (indicating that the F2.4.2 fraction belonged to the group A prothrombin activators because it clotted citrate plasma without the need for any cofactor (26, 41, 42). The F5.1 fraction could belong to one of these anticoagulant families: Molecules with enzymatic activity such as phospholipases A2 (PLA2) enzymes, fibrinogenolytic SVMPs, protein C activators, L-amino acid oxidases, as well as non-enzymatic molecules such as C-type lectin-like proteins (Snaclecs or SVCLPs), three-finger toxins (TFTs), and Kunitz-type proteinase inhibitors (26). More studies are needed to understand the exact mechanisms of action of these factors.
Acknowledgements
References
-
1.
Almeida JR, Resende LM, Watanabe RK, Carregari VC, Huancahuire-Vega S, da S. Caldeira CA, et al. Snake Venom Peptides and Low Mass Proteins: Molecular Tools and Therapeutic Agents. Curr Med Chem. 2017;24(30):3254-82. [PubMed ID: 27804880]. https://doi.org/10.2174/0929867323666161028155611.
-
2.
Gutierrez JM, Calvete JJ, Habib AG, Harrison RA, Williams DJ, Warrell DA. Snakebite envenoming. Nat Rev Dis Primers. 2017;3:17063. [PubMed ID: 28905944]. https://doi.org/10.1038/nrdp.2017.63.
-
3.
Fry BG, Wuster W. Assembling an arsenal: origin and evolution of the snake venom proteome inferred from phylogenetic analysis of toxin sequences. Mol Biol Evol. 2004;21(5):870-83. [PubMed ID: 15014162]. https://doi.org/10.1093/molbev/msh091.
-
4.
Mackessy SP, Baxter LM. Bioweapons synthesis and storage: The venom gland of front-fanged snakes. Zool Anz J Comp Zool. 2006;245(3-4):147-59. https://doi.org/10.1016/j.jcz.2006.01.003.
-
5.
Calvete JJ, Sanz L, Angulo Y, Lomonte B, Gutierrez JM. Venoms, venomics, antivenomics. FEBS Lett. 2009;583(11):1736-43. [PubMed ID: 19303875]. https://doi.org/10.1016/j.febslet.2009.03.029.
-
6.
Vonk FJ, Jackson K, Doley R, Madaras F, Mirtschin PJ, Vidal N. Snake venom: From fieldwork to the clinic: Recent insights into snake biology, together with new technology allowing high-throughput screening of venom, bring new hope for drug discovery. Bioessays. 2011;33(4):269-79. [PubMed ID: 21271609]. https://doi.org/10.1002/bies.201000117.
-
7.
Vaiyapuri S, Reeks T, Fry BG; Sunagar; Gibbins; Jackson. Kallikrein enzymes. In: Fry BG, editor. Venomous reptiles and they're toxins: evolution, pathophysiology, and biodiscovery. New York: Oxford University Press; 2015. p. 267-80.
-
8.
Calvete JJ, Fry BG; Casewell; Sunagar; Takacs; Jackson. Snake Venom Metalloprotease Enzymes. In: Fry BG, editor. Venomous Reptiles and Their Toxins: Evolution, Pathophysiology and Biodiscovery. New York: Oxford University Press; 2015. p. 347-63.
-
9.
Fry BG, Vidal N, van der Weerd L, Kochva E, Renjifo C. Evolution and diversification of the Toxicofera reptile venom system. J Proteomics. 2009;72(2):127-36. [PubMed ID: 19457354]. https://doi.org/10.1016/j.jprot.2009.01.009.
-
10.
Kang TS, Georgieva D, Genov N, Murakami MT, Sinha M, Kumar RP, et al. Enzymatic toxins from snake venom: structural characterization and mechanism of catalysis. FEBS J. 2011;278(23):4544-76. [PubMed ID: 21470368]. https://doi.org/10.1111/j.1742-4658.2011.08115.x.
-
11.
Fox JW, Serrano SM. Exploring snake venom proteomes: multifaceted analyses for complex toxin mixtures. Proteomics. 2008;8(4):909-20. [PubMed ID: 18203266]. https://doi.org/10.1002/pmic.200700777.
-
12.
Georgieva D, Arni RK, Betzel C. Proteome analysis of snake venom toxins: pharmacological insights. Expert Rev Proteomics. 2008;5(6):787-97. [PubMed ID: 19086859]. https://doi.org/10.1586/14789450.5.6.787.
-
13.
Alvarez-Flores MP, Faria F, de Andrade SA, Chudzinski-Tavassi AM. Snake Venom Components Affecting the Coagulation System. In: Gopalakrishnakone P, Inagaki H, Mukherjee A, Rahmy T, Vogel CW, editors. Snake Venoms. Toxinology. Dordrecht: Springer; 2016. p. 1-20. https://doi.org/10.1007/978-94-007-6648-8_31-1.
-
14.
Yamazaki Y, Morita T. Snake venom components affecting blood coagulation and the vascular system: structural similarities and marked diversity. Curr Pharm Des. 2007;13(28):2872-86. [PubMed ID: 17979732]. https://doi.org/10.2174/138161207782023775.
-
15.
Tans G, Rosing J. Snake venom activators of factor X: an overview. Haemostasis. 2001;31(3-6):225-33. [PubMed ID: 11910189]. https://doi.org/10.1159/000048067.
-
16.
Estevao-Costa MI, Sanz-Soler R, Johanningmeier B, Eble JA. Snake venom components in medicine: From the symbolic rod of Asclepius to tangible medical research and application. Int J Biochem Cell Biol. 2018;104:94-113. [PubMed ID: 30261311]. https://doi.org/10.1016/j.biocel.2018.09.011.
-
17.
Jenkins TP, Ahmadi S, Bittenbinder MA, Stewart TK, Akgun DE, Hale M, et al. Terrestrial venomous animals, the envenomings they cause, and treatment perspectives in the Middle East and North Africa. PLoS Negl Trop Dis. 2021;15(12). e0009880. [PubMed ID: 34855751]. [PubMed Central ID: PMC8638997]. https://doi.org/10.1371/journal.pntd.0009880.
-
18.
Lowry O, Rosebrough N, Farr A, Randall R. Protein Measurement with the Folin Phenol Reagent. J Biol Chem. 1951;193(1):265-75. https://doi.org/10.1016/s0021-9258(19)52451-6.
-
19.
Hamilton MA, Russo RC, Thurston RV. Trimmed Spearman-Karber method for estimating median lethal concentrations in toxicity bioassays. Environ Sci Technol. 2002;11(7):714-9. https://doi.org/10.1021/es60130a004.
-
20.
Dizaji R, Sharafi A, Pourahmad J, Abdollahifar MA, Vatanpour H, Hosseini MJ. Induction of two independent immunological cell death signaling following hemoglobinuria -induced acute kidney injury: In vivo study. Toxicon. 2019;163:23-31. [PubMed ID: 30890325]. https://doi.org/10.1016/j.toxicon.2019.03.011.
-
21.
Laemmli UK. Cleavage of structural proteins during the assembly of the head of bacteriophage T4. Nature. 1970;227(5259):680-5. [PubMed ID: 5432063]. https://doi.org/10.1038/227680a0.
-
22.
Mackie IJ, Kitchen S, Machin SJ, Lowe GD; Haemostasis; Haemostasis and Thrombosis Task Force of the British Committee for Standards in Haematology. Guidelines on fibrinogen assays. Br J Haematol. 2003;121(3):396-404. [PubMed ID: 12716361]. https://doi.org/10.1046/j.1365-2141.2003.04256.x.
-
23.
Jennings I, Kitchen DP, Woods T, Kitchen S, Walker ID. Differences between multifibrin U and conventional Clauss fibrinogen assays: data from UK National External Quality Assessment Scheme surveys. Blood Coagul Fibrinolysis. 2009;20(5):388-90. [PubMed ID: 19542938]. https://doi.org/10.1097/MBC.0b013e328329e446.
-
24.
Marsh N, Williams V. Practical applications of snake venom toxins in haemostasis. Toxicon. 2005;45(8):1171-81. [PubMed ID: 15922782]. https://doi.org/10.1016/j.toxicon.2005.02.016.
-
25.
Choudhury M, Suvilesh KN, Vishwanath BS, Velmurugan D. EC-PIII, a novel non-hemorrhagic procoagulant metalloproteinase: Purification and characterization from Indian Echis carinatus venom. Int J Biol Macromol. 2018;106:193-9. [PubMed ID: 28782616]. https://doi.org/10.1016/j.ijbiomac.2017.08.006.
-
26.
Yamada D, Sekiya F, Morita T. Isolation and characterization of carinactivase, a novel prothrombin activator in Echis carinatus venom with a unique catalytic mechanism. J Biol Chem. 1996;271(9):5200-7. [PubMed ID: 8617803]. https://doi.org/10.1074/jbc.271.9.5200.
-
27.
Hamza L, Gargioli C, Castelli S, Rufini S, Laraba-Djebari F. Purification and characterization of a fibrinogenolytic and hemorrhagic metalloproteinase isolated from Vipera lebetina venom. Biochimie. 2010;92(7):797-805. [PubMed ID: 20188789]. https://doi.org/10.1016/j.biochi.2010.02.025.
-
28.
Pook CE, Joger U, Stumpel N, Wuster W. When continents collide: phylogeny, historical biogeography and systematics of the medically important viper genus Echis (Squamata: Serpentes: Viperidae). Mol Phylogenet Evol. 2009;53(3):792-807. [PubMed ID: 19666129]. https://doi.org/10.1016/j.ympev.2009.08.002.
-
29.
Hao Z, Liu M, Counsell C, Wardlaw JM, Lin S, Zhao X. Fibrinogen depleting agents for acute ischaemic stroke. Cochrane Database Syst Rev. 2012;(3). CD000091. [PubMed ID: 22419274]. https://doi.org/10.1002/14651858.CD000091.pub2.
-
30.
Waheed H, Moin SF, Choudhary MI. Snake Venom: From Deadly Toxins to Life-saving Therapeutics. Curr Med Chem. 2017;24(17):1874-91. [PubMed ID: 28578650]. https://doi.org/10.2174/0929867324666170605091546.
-
31.
Serrano SM. The long road of research on snake venom serine proteinases. Toxicon. 2013;62:19-26. [PubMed ID: 23010164]. https://doi.org/10.1016/j.toxicon.2012.09.003.
-
32.
Asadi H, Yan B, Dowling R, Wong S, Mitchell P. Advances in medical revascularisation treatments in acute ischemic stroke. Thrombosis. 2014;2014:714218. [PubMed ID: 25610642]. [PubMed Central ID: PMC4293866]. https://doi.org/10.1155/2014/714218.
-
33.
Dempfle CE, Alesci S, Kucher K, Muller-Peltzer H, Rubsamen K, Borggrefe M. Plasminogen activation without changes in tPA and PAI-1 in response to subcutaneous administration of ancrod. Thromb Res. 2001;104(6):433-8. [PubMed ID: 11755954]. https://doi.org/10.1016/s0049-3848(01)00391-7.
-
34.
Qin J, Xu Z, Shi D, Chen D, Dai J, Teng H, et al. Deep vein thrombosis after total hip arthroplasty and total knee arthroplasty in patients with previous ischemic stroke. Int J Low Extrem Wounds. 2013;12(4):316-9. [PubMed ID: 24043672]. https://doi.org/10.1177/1534734613493291.
-
35.
McCleary RJ, Kini RM. Snake bites and hemostasis/thrombosis. Thromb Res. 2013;132(6):642-6. [PubMed ID: 24125598]. https://doi.org/10.1016/j.thromres.2013.09.031.
-
36.
Mehdizadeh Kashani T, Vatanpour H, Zolfagharian H, Hooshdar Tehrani H, Heydari MH, Kobarfard F. Partial Fractionation of Venoms from Two Iranian Vipers, Echis carinatus and Cerastes persicus Fieldi and Evaluation of Their Antiplatelet Activity. Iran J Pharm Res. 2012;11(4):1183-9. [PubMed ID: 24250552]. [PubMed Central ID: PMC3813148].
-
37.
Babaie M, Salmanizadeh H, Zolfagharian H. Blood coagulation induced by Iranian saw-scaled viper (echis carinatus) venom: identification, purification and characterization of a prothrombin activator. Iran J Basic Med Sci. 2013;16(11):1145-50. [PubMed ID: 24494066]. [PubMed Central ID: PMC3909625].
-
38.
Salmanizadeh H, Zolfagharian H, Babaie M. Coagulopathy Caused by the Main Anticoagulant Fractions of Echis carinatus Snake Venom on Blood. Int J Nano Stud Technol. 2015;4(4):93-9. https://doi.org/10.19070/2167-8685-1500018.
-
39.
Amrollahi Byoki E, Zare Mirakabadi A. Partial purification and characterization of anticoagulant factor from the snake (echis carinatus) venom. Iran J Basic Med Sci. 2013;16(11):1139-44. [PubMed ID: 24494065]. [PubMed Central ID: PMC3909624].
-
40.
Rosing J, Tans G. Structural and functional properties of snake venom prothrombin activators. Toxicon. 1992;30(12):1515-27. https://doi.org/10.1016/0041-0101(92)90023-x.
-
41.
Kini RM, Joseph JS, Rao VS. Prothrombin activators from snake venoms. In: Mènez A, editor. Perspectives in Molecular Toxinology. Chichester: John Wiley & Sons; 2002. p. 341-56.
-
42.
Takeya H, Nishida S, Miyata T, Kawada S, Saisaka Y, Morita T, et al. Coagulation factor X activating enzyme from Russell's viper venom (RVV-X). A novel metalloproteinase with disintegrin (platelet aggregation inhibitor)-like and C-type lectin-like domains. J Biol Chem. 1992;267(20):14109-17. [PubMed ID: 1629211].