Abstract
Background:
Postoperative peritoneal adhesions are among common challenging problems in surgery. The availability of limited efficient strategies to prevent intra-abdominal adhesion reinforces the need to explore new methods. Given the favorable prolonged drug release characteristics of polycaprolactone (PCL) films and their ability to act as a biodegradable physical barrier implant, along with the anti-inflammatory and anti-adhesion properties of indomethacin and phospholipids, this study hypothesized that indomethacin sustained-release membrane composed of phosphatidylcholine (PC) and PCL blend could efficiently prevent abdominal adhesion formation.Methods:
Different polymeric and polymeric/lipidic hybrid formulations with three feeding materials to drug weight ratios were prepared, and their physicochemical characteristics and drug release kinetics were evaluated and compared. Abdominal adhesions were induced in 48 rats by the abrasion of the cecum and excision of a section of the opposite abdominal wall. Adhesion formation was evaluated by macroscopic scoring, histological, scanning electron microscopy, and polymerase chain reaction analyses.Results:
Both PCL and PCL-PC films exhibited sustained indomethacin release profiles. The X-ray diffraction and Fourier-transform infrared spectroscopy studies confirmed indomethacin incorporation in formulations in molecular dispersion form without any interaction. The films showed smooth surfaces and good mechanical properties. The treatment with indomethacin PCL-PC membrane significantly reduced the expression levels of tumor necrosis factor-alpha, transforming growth factor-beta, interleukin-1, interleukin-6, and fibrinogen in the adhesion tissues. The separation of the injured peritoneum, very low adhesion scores, and complete mesothelial cell regeneration were also achieved.Conclusions:
This study suggests that indomethacin-eluting PCL-PC membrane acting through the combination of physical barrier, anti-inflammatory agents, and controlled drug delivery warrants an effective approach to prevent intra-abdominal adhesion.Keywords
1. Background
Postoperative peritoneal adhesions are among the serious complications in surgery, with an incidence rate of 67 - 93%. Surgical injuries can result in inflammatory responses at the peritoneal surface, releasing serosanguinous fluid and exudates, extracellular matrix deposition, fibroblast migration and proliferation, and development of intra-abdominal and intra-pelvic adhesions. Adhesion-related complications might develop throughout the lifetime of patients with surgical history, causing substantial abdominal and pelvic pain, difficulty in reoperation, infertility, bowel obstruction, and impairing patients’ quality of life (1-3). Therefore, the inhibition of intra-abdominal adhesion formation is a high-priority goal to prevent the above-mentioned adverse effects.
Various approaches have been put forth to reduce the extent and severity of postsurgical adhesions, including physical barrier devices (to prevent the contact of the injured sites with the adjacent tissues) (4, 5) and pharmacological agents (e.g., anti-inflammatory agents, antibiotics, fibrinolytic agents, and anticoagulants) (6, 7). A combination of these two strategies can more successfully manage postoperative adhesions.
Some studies have focused on the local application of physical adhesion barriers, such as bioabsorbable and biocompatible polymeric films (8, 9). Polymeric films can also provide a good platform for controlled and localized drug delivery depots (10, 11). Sodium hyaluronate/carboxymethylcellulose (SeprafilmTM) is a bioreabsorbable membrane to prevent adhesion formation. SeprafilmTM is used as a physical barrier with no pharmacological agent and could partly prevent tissue adhesion (1-4).
SurgiWrapTM bioresorbable barrier film is fabricated from the lactic acid to prevent scar tissue formation and adhesion between tissues. The SurgiWrapTM is made from an amorphous bioresorbable copolymer of 70: 30 poly(L-lactide-co-D, L-lactide). SurgiWrapTM can heal the tissues and prevent adhesion; however, the use of SurgiWrapTM was not associated with complete anti-adhesion properties (5).
Polycaprolactone (PCL) polymer is biocompatible, biodegradable, and semicrystalline aliphatic polyester with immense potential for various biomedical applications (8). The PCL films were significantly efficacious in reducing adhesion formation in the rat abdominal adhesion model (8, 9). Polycaprolactone films can also provide a sustained delivery system (10, 11).
As inflammatory reactions induced by tissue injury play a critical role in adhesion development, numerous anti-inflammatory compounds have been detected to inhibit adhesion formation (12-14). Nonsteroidal anti-inflammatory drugs (NSAIDs) are used to manage inflammatory reactions and postoperative pain. However, following systemic administration, these drugs can cause adverse effects, including stomach pain, nausea, vomiting, and impairment of renal function (15-17). Moreover, the short-term residence of peritoneal delivery of these agents (18) could hamper the effective prevention of adhesion formation.
The current study hypothesized that a biocompatible, biodegradable, and controlled drug delivery film could provide a prolonged release of anti-inflammatory agents around the injured site and act as an anti-adhesive physical barrier to better manage abdominal adhesion and postoperative pain. The phospholipid enrichment of the drug films can have additional anti-adhesive and anti-inflammatory effects. Indomethacin can reduce inflammatory responses and consequently tissue adhesions at the injured sites.
The present study aimed to firstly prepare and characterize PCL and PCL-PC films for controlled indomethacin intraperitoneal delivery and secondly assess and compare the effectiveness of indomethacin-loaded PCL and PCL-PC films in the prevention of abdominal adhesion formation by macroscopic scoring, histological, scanning electron microscopy (SEM), and polymerase chain reaction (PCR) analyses.
2. Objectives
This study aimed to evaluate the potential in vivo superiority of drug films to drug suspension on the one hand and polymer-lipid hybrid films to polymer films on the other hand.
3. Methods
3.1. Materials
Indomethacin (purity > 98%) was kindly provided by Arya Pharmaceutical Company (Tehran, Iran). Soybean PC was purchased from Lipoid GmbH (Ludwigshafen, Germany). The PCL, sodium chloride, dichloromethane (DCM), chloroform, and methanol were purchased from Merck/Sigma-Aldrich (Germany).
3.2. Film Preparation
Films were prepared by the solvent casting technique. The required amounts of PCL, PC, and indomethacin were dissolved in 8 mL of chloroform using a magnetic stirrer for 10 minutes. Three ratios of drug to feeding materials (i.e., 1: 15, 1: 30, and 1: 45) and two PCL to PC ratios (i.e., 50: 50 and 70: 30) were chosen for film preparation (Table 1). The prepared solutions were cast into Petri dishes and dried overnight at room temperature. After the complete drying, they were peeled off and cut into pieces.
3.3. Film Characterization
3.3.1. Drug Content
The uniformity of drug distribution was investigated by random sampling of all drug films. The samples (2 mg) were dissolved in DCM and chloroform (50: 50 v/v) by vigorous mixing. After appropriate dilution with methanol, the solutions were filtered and analyzed spectrophotometrically (Shimadzu-1800 UV-visible spectrophotometer, Shimadzu, Japan) at 318 nm. For each drug film, the experiments were performed in triplicate.
3.3.2. Film Thickness
The thickness of film formulations was measured at ten different points (in the middle and in the four corners) using a micrometer (Mitutoyo, Japan).
Composition and Characterization of Various Polymer and Polymer-Lipid Indomethacin Films a
Formulation | Composition (PCL: PC) | Feeding Materials to Drug Weight Ratio | Drug Content (%) | Thickness (mm) |
---|---|---|---|---|
F1 | 100: 0 | 15 | 92.9 ± 1.3 | 0.074 ± 0.005 |
F2 | 100: 0 | 30 | 100.5 ± 2.4 | 0.138 ± 0.009 |
F3 | 100: 0 | 45 | 104.2 ± 3.2 | 0.152 ± 0.019 |
F4 | 70: 30 | 15 | 102.8 ± 2.3 | 0.078 ± 0.006 |
F5 | 70: 30 | 30 | 98.0 ± 1.3 | 0.138 ± 0.011 |
F6 | 70: 30 | 45 | 100.5 ± 5.2 | 0.146 ± 0.013 |
F7 | 50: 50 | 15 | 93.0 ± 1.2 | 0.068 ± 0.005 |
F8 | 50: 50 | 30 | 91.8 ± 2.0 | 0.107 ± 0.006 |
F9 | 50: 50 | 45 | 94.1 ± 1.1 | 0.202 ± 0.005 |
3.3.3. In Vitro Release Study
For in vitro drug release study, the film pieces (containing 2 mg indomethacin) were placed in Falcon tubes filled with 30 mL of normal saline and shaken at 37°C and 50 rpm. The samples were withdrawn at predetermined time intervals (i.e., 2, 4, 6, 8, and 24 hours) for 24 hours and then every 24 hours until the end of the study and refilled with an equal volume of fresh medium. Every 24 hours, the release medium was completely refreshed. Release studies were carried out in triplicate for all drug formulations. The drug concentration was determined using a spectrophotometer (Shimadzu, Japan) set at an absorbance wavelength of 318 nm.
3.3.4. X-Ray Diffraction Analysis
The X-ray diffraction (XRD) patterns of indomethacin, PCL, PC, F3, F4, plain PCL films, and plain PCL-PC films were recorded using an EQuinox 3000 diffractometer (Inel, France) with Cu Ka radiation (45 kV and 40 mA). The scanning angle was adjusted from 10° to 80°.
3.3.5. Fourier-Transform Infrared Spectroscopy
The Fourier-transform infrared spectroscopy (FTIR) spectra of the samples (i.e., indomethacin, PCL, PC, physical mixture, F3, F4, plain PCL films, and plain PCL-PC films) were measured on a WQF-510 (Rayleigh Optics, China) using potassium bromide disks within the spectral range of 400 - 4000 cm-1 with a resolution of 4 cm-1.
3.3.6. Scanning Electron Microscopy and Atomic Force Microscopy
The films were cut with a razor blade, coated with gold, and then observed with an SEM instrument (Tescan/Mira, Brno, Czech Republic) to analyze surface morphology. Atomic force microscopy (AFM) images were obtained using a JPK NanoWizard (JPK Instruments, Germany) integrated with a Zeiss Axiovert 200M (Carl Zeiss, Germany) in the tapping mode.
3.3.7. Mechanical Properties
The mechanical properties of F3 and F4 (employing 15 mm × 15 mm films) were determined at room temperature using a SANTAM STM-20 instrument (Tehran, Iran). Tensile strength, Young’s modulus, and elongation at break were calculated using the following equations (19, 20):
Where Fmax is the maximum load; A is the initial cross-sectional area; F is the force; ΔL is the change in length; L0 is the original length.
3.4. In Vivo Experiments
3.4.1. Animals
Male Wistar rats (250 - 270 g) were provided by Pasteur Institute of Iran. The rats were kept in a temperature- and humidity-controlled room under artificial lighting of a 12-hour light/dark cycle. The in vivo study was performed following the guidelines of the Ethics Committee of Shahid Beheshti University of Medical Sciences, Tehran, Iran, and in accordance with the National Institutes of Health Guide for the Care and Use of Laboratory Animals (21).
3.4.2. Surgical Procedure and Insertion of Anti-adhesion Films
After anesthesia with a mixture of medetomidine hydrochloride (0.5 mg/kg) and ketamine hydrochloride (50 mg/kg), the animals were maintained in the supine position, and the abdominal area was shaved and disinfected with 70% alcohol solution. A 5-cm incision was made in the skin and abdominal muscles along the linea alba. The cecum defect was induced by abrading with a sterile nail file 20 times until petechiae without hemostasis occurred. Then, a 2 × 2 cm anterior area of the superficial layer of the parietal peritoneum directly exposed to the cecum was excised from the abdominal wall by a scalpel (No. 20), and the cecum was placed in the peritoneal cavity. The animals were randomly allocated into six groups with eight rats in each group, namely (1) control untreated group (G1); (2) suspension group (G2) with 1 mL drug suspension applied on the injured site; (3) plain PCL film (G3); (4) plain PCL-PC film (G4); (5) indomethacin-PCL film (G5); and (6) indomethacin-PCL-PC film (G6). The drug dose was 3 mg per rat. The films were positioned onto the abdominal wall with four 5/0 nylon sutures to form a barrier between the damaged cecum and peritoneum. The abdominal wall was finally closed using 4/0 Vicryl sutures.
3.4.3. Adhesion Evaluation
3.4.3.1. Macroscopic Scoring
Two weeks after surgery, the animals were euthanized for the gross evaluation of the severity and extent of postoperative abdominal adhesion. The abdomen was reopened, and the injured site was inspected to score peritoneal adhesions. The extent and severity of the adhesions were evaluated based on the scoring system of Table 2 (22).
Scoring System for In Vivo Evaluation of Tissue Adhesion Using a Rat Abdominal Adhesion Model
Adhesion Grade | Grade Description | Separation Description |
---|---|---|
0 | No adhesive bands between the cecum and muscles of the abdominal wall | - |
1 | One loose adhesive band between the cecum and muscles of the abdominal wall | Adhesion is easily separated |
2 | Two adhesive bands between the cecum and muscles of the abdominal wall | Adhesion is easily separated |
3 | More than two firmed adhesive bands between the cecum and muscles of the abdominal wall | Adhesion is separated by slight pulling |
4 | The cecum tightly adheres to the muscles of the abdominal wall | Adhesion is separated by hard pulling |
3.4.3.2. Histological Analysis
The specimens obtained from the damaged cecum and abdominal wall were fixed in 4% formaldehyde, embedded in paraffin, and serial 6 µm sections were prepared. The sections were examined and imaged after hematoxylin and eosin (H&E) and Masson’s trichrome staining.
3.4.3.3. SEM Analyses
After resection, the damaged abdominal wall, damaged cecum, and adhesion tissues were immediately fixed in 2.5% glutaraldehyde. The specimens were gradually dehydrated by incubation in ethanol solutions, air-dried, mounted on aluminum holders, and finally sputtered with gold.
3.4.3.4. Real-Time PCR Assays
Quantitative reverse transcription-polymerase chain reaction (RT-PCR) was performed to measure the messenger ribonucleic acid (mRNA) levels of tumor necrosis factor-alpha (TNF-α), transforming growth factor-beta (TGF-β), interleukin-1 (IL-1), interleukin-6 (IL-6), fibrinogen, and alpha-smooth muscle actin (α-SMA). The harvested tissues were subjected to Trizol extraction (Invitrogen, Belgium) for total RNA isolation. One μg of total RNA was reverse transcribed into the first-strand complementary deoxyribonucleic acid (cDNA). The resulting cDNA was subjected to quantitative RT-PCR analysis targeting TNF-α, TGF-β, IL-1, IL-6, fibrinogen, and α-SMA on a StepOnePlus Real-Time PCR System (Applied Biosystems, USA) using EvaGreen®. The mRNA expression levels were measured according to the comparative ΔΔCT method.
3.5. Statistical Analysis
The results were reported as mean ± standard error of mean. The obtained results were statistically compared by the Student’s t-test and analysis of variance with the least significant difference posthoc test using SPSS software (version 17.0). A p-value less than 0.05 was considered statistically significant.
4. Results
4.1. In Vitro Preparation and Characterization of Indomethacin Film Formulations
Different polymeric and polymeric/lipidic hybrid formulations with three feeding materials to drug weight ratios (i.e., 15, 30, and 45) were prepared (Table 1). Homogeneous films were achieved with all compositions; therefore, all formulations were characterized regarding film thickness, drug content, and release profile.
Table 1 shows the results of film thickness and drug content. The expected trend in film thickness as a function of feeding materials to drug weight ratio was evident; by increasing the ratio from 15 to 45, film thickness increased from 0.07 - 0.08 to 0.15 - 0.20 mm for both PCL and PCL-PC films. According to the results (Table 1), the drug content of PCL films (F1-F3), PCL-PC films containing 30% lipid (F4-F6), and PCL-PC films containing 50% lipid (F7-F9) was observed to be within the acceptable ranges of 92.9 - 104.2%, 98.0 - 102.8%, and 91.8 - 94.1%, respectively.
Figure 1 shows the release profiles of indomethacin from different formulations. During the first 24 hours, polymeric formulations showed drug release percentages of 17.4%, 12.4%, and 9.7% for films prepared with 15, 30, and 45 polymers to drug (P: D) ratios, respectively.
In vitro indomethacin release profiles from A, polycaprolactone (PCL) films; B, PCL-phosphatidylcholine (PC) films containing 30% PC; C, PCL-PC films containing 50% PC in normal saline at 37°C (n = 3; mean ± standard error of mean)
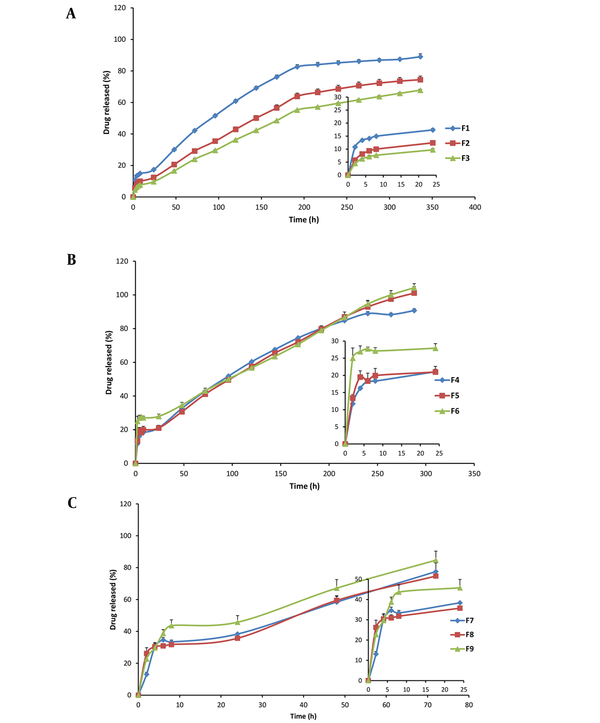
Cumulative drug release of 89.0%, 74.4%, and 67.8% after 24 hours related to the PCL films was achieved for F1, F2, and F3 films, respectively, at the end of the 2 weeks. F3 with a P: D ratio of 45 showed significantly lower initial and 14-day cumulative drug release than F2 and F3 (P < 0.05); therefore, F3 was selected among polymeric films. In the case of PCL-PC formulations with 30% PC, cumulative drug release at the end of 24 hours was observed to be 21.0%, 21.1%, and 27.9% for F4, F5, and F6, respectively. Cumulative drug release percentages were about 90% for F4 and about 100% for F5 and F6 in 288 hours. F4 was also selected due to the lower P: D ratio and comparable release rate to F5 and F6. In the case of PCL-PC formulations with 50% PC, cumulative drug release at the end of 24 hours was observed to be 38.4%, 35.7%, and 45.8% for F7, F8, and F9, respectively. Cumulative drug release of all 50% PC enriched formulations was higher than 75% in 72 hours. As the PC percentage became higher, the indomethacin release became faster. Therefore, F7, F8, and F9 formulations were excluded from further studies.
Figure 2 depicts the XRD patterns of indomethacin, PCL, and phospholipid, their physical mixture, and formulations. The PCL exhibited two broad characteristic peaks at 21.7° and 24.2° suggesting its semicrystalline nature. Only one broad and diffuse peak (∼2θ = 20°) was observed in the PC pattern implying its amorphous state. The presence of characteristic peaks at 2θ of 10.4, 11.9, 16.9, 19.9, and 22.1° in the diffractogram of indomethacin confirmed its highly crystalline characteristics. However, no crystalline pattern was detected after incorporation into films.
X-ray diffraction spectra of indomethacin, polycaprolactone (PCL), phosphatidylcholine (PC), plain PCL film, plain PCL-PC film, indomethacin PCL film, indomethacin PCL-PC film, and physical mixture
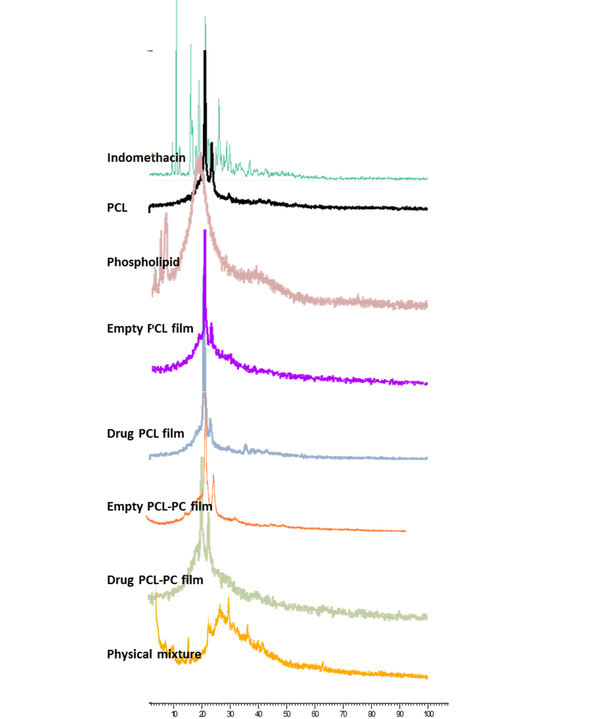
The bands at 834, 1590, 1690, and 1712 cm-1 presented in the FTIR spectrum of indomethacin resulted from the vibrations of C-Cl, C = C, amide C = O, and carboxylic acid C = O, respectively. The bands of PCL that could be observed at 1728, 2864, and 2933 cm-1 corresponded to carbonyl, symmetric aliphatic, and asymmetric aliphatic stretching, respectively (23). The most prominent bands of PC spectrum at 1246, 1461, 1735, 2854, and 2924 cm-1 corresponded to the antisymmetric PO2-, CH2 scissoring, C = O, symmetric CH2, and antisymmetric CH2, respectively (24). In the spectrum of drug films, the characteristic absorption peaks of indomethacin were almost masked by those of PCL and PC (Figure 3).
Fourier-transform infrared spectroscopy spectra of indomethacin, polycaprolactone (PCL), phosphatidylcholine (PC), plain PCL film, plain PCL-PC film, indomethacin PCL film, indomethacin PCL-PC film, and physical mixture
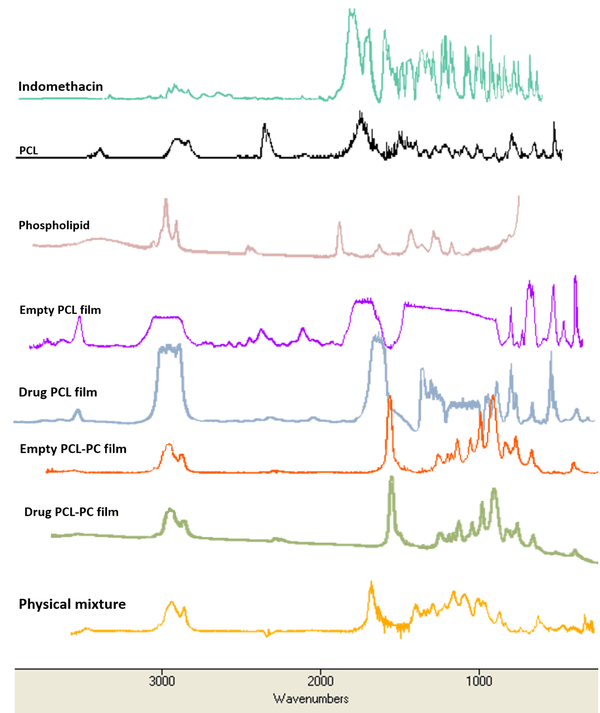
According to SEM observation (Figure 4), drug-loaded films showed smooth surfaces with almost no pore. The SEM results represented a smoother surface of the film in the absence of PC. The AFM observations were in good agreement with the SEM results.
Scanning electron microscopy (SEM) and atomic force microscopy (AFM) [two-dimensional (2D) and three-dimensional (3D)] images of indomethacin polycaprolactone (PCL) film (upper panel) and indomethacin PCL-phosphatidylcholine (PC) film (lower panel)
![Scanning electron microscopy (SEM) and atomic force microscopy (AFM) [two-dimensional (2D) and three-dimensional (3D)] images of indomethacin polycaprolactone (PCL) film (upper panel) and indomethacin PCL-phosphatidylcholine (PC) film (lower panel) Scanning electron microscopy (SEM) and atomic force microscopy (AFM) [two-dimensional (2D) and three-dimensional (3D)] images of indomethacin polycaprolactone (PCL) film (upper panel) and indomethacin PCL-phosphatidylcholine (PC) film (lower panel)](https://services.brieflands.com/cdn/serve/315e0/ebc841984c2f03f79df98ea30ce5cc3c4b242b44/ijpr-21-1-127353-i004-preview.png)
The mechanical curves of PCL and PCL-PC films and differences in the tensile strength, Young’s modulus, and elongation at break values upon the addition of PC were investigated (Figure 5). The PC enrichment changed Fmax (from 10.7 to 1.1 N) and modulus values (from 71.40 to 90.22 MPa) in film formulations. Elongation at break percentages were 61.10% and 57.24% for PC-free and PC-containing formulations.
Mechanical properties of indomethacin polycaprolactone (PCL) and PCL-phosphatidylcholine (PC) films
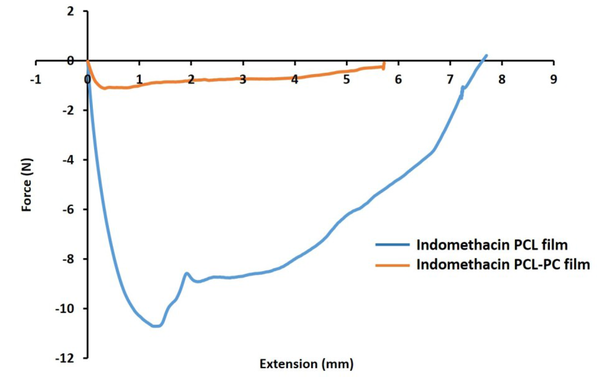
4.2. In Vivo Anti-adhesion Efficacy of Indomethacin Film Formulations
The effects of local administration of indomethacin and phospholipid enrichment of polymeric film formulations were investigated in a rat cecal adhesion model (Figure 6) 14 days after surgery using the scoring system shown in Table 2. Intra-abdominal adhesion formation and macroscopic adhesion scores of all groups are shown in Figure 7 and Table 3, respectively. The animals in the untreated group (G1) showed severe adhesions with seven rats developing score 4 adhesions. The treatment with drug suspension (G2) and plain PCL films (G3) reduced intraperitoneal adhesions with respect to the control group, as 62% of animals with a score 2 and 38% of animals with a score 1 were in the drug suspension group, and 50% of animals with a score 2 and 50% of animals with a score 3 were in PCL film group. The addition of PC to PCL formulations decreased adhesion scores in plain films (G4) (five rats scored 1; three rats scored 2) and in drug-loaded films (G6) (seven rats scored 0; one rat scored 1).
Surgical procedure of intra-abdominal adhesion induction and insertion of anti-adhesion films in a rat model
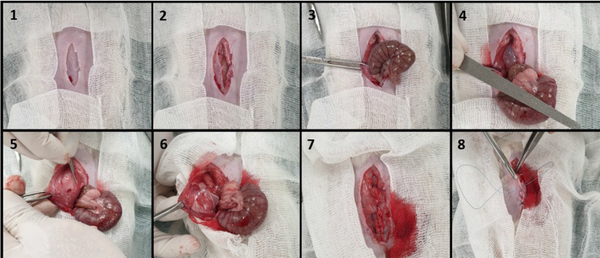
With no tissue adhesion in seven out of eight rats, the observations indicated that drug-loaded PCL-PC films could be used as a potential adhesion barrier.
macroscopic scoring and histopathological evaluation of injured peritoneal sites of various treatment groups 2 weeks after surgery. Polycaprolactone (PCL) and PCL-phosphatidylcholine (PCL-PC) films with and without drug were evaluated.
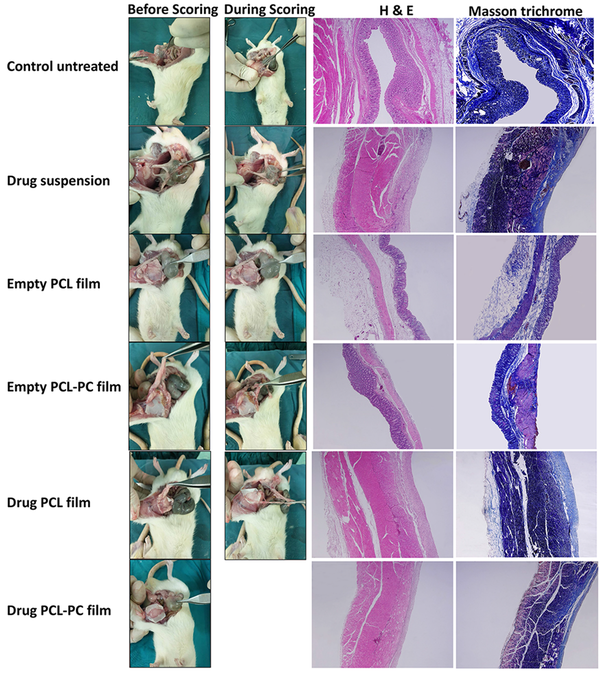
Afterward, histological analysis was performed by H&E stain and Masson’s trichrome staining (Figure 7). The histopathological studies of abdominal wall defect of the control group showed remarkable inflammatory cells and no sign of remesothelialization. Microscopic images related to the drug suspension group exhibited less inflammation and minimal collagen deposition. In the cecal tissue samples of animals treated with plain PCL films, there was an incomplete mesothelial cell layer with negligible inflammation. Mesothelial cells and only a negligible inflammatory reaction could be observed in the plain PCL-PC film group. Drug-loaded PCL-PC films exhibited a complete regeneration of the injured cecum and no inflammation.
Tissue Adhesion Scores Evaluated In Vivo Using a Rat Abdominal Adhesion Model (n = 8)
Score | Control Untreated | Drug Suspension | Plain PCL Film | Plain PCL-PC Film | Drug PCL Film | Drug PCL-PC Film |
---|---|---|---|---|---|---|
0 | 0 | 0 | 0 | 0 | 0 | 7 |
1 | 0 | 3 | 0 | 5 | 4 | 1 |
2 | 0 | 5 | 4 | 3 | 4 | 0 |
3 | 1 | 0 | 4 | 0 | 0 | 0 |
4 | 7 | 0 | 0 | 0 | 0 | 0 |
The SEM observations (Figure 8) in the control group were consistent with the infiltration of numerous white blood cells and giant foreign body cells, along with the formation of a tight and intense connective tissue. In plain films and drug suspension, the penetration of macrophages, lymphocytes, and fibroblasts was reduced. Moreover, the loose connective tissues and zones of incomplete mesothelial cell deposition were observed. The drug-loaded PCL-PC barrier induced optimal mesothelialization and showed adhesion-free sectors (Figure 8).
Scanning electron microscopy micrographs of the surface of injured peritoneum in various treatment groups 2 weeks after surgery. Polycaprolactone (PCL) and PCL-phosphatidylcholine (PCL-PC) films with and without drug were evaluated.
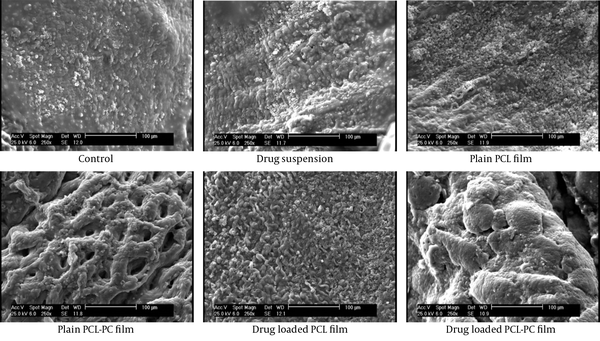
In this study, the levels of proinflammatory cytokines (i.e., TNF-α, TGF-β1, IL-1β, and IL-6), fibrinogen, and α-SMA were quantified to examine the anti-adhesion efficacy of indomethacin films. When compared to the control group, decreased TNF-α and TGF-β1 gene expression were observed in all treated animals, with statistically significant values only in the drug-loaded PCL-PC film group. The IL-1β, IL-6, and fibrinogen levels were reduced in treated rats, compared to those of the control group, with maximum reduction in the drug-loaded PCL-PC film barrier. The order of α-SMA gene expression was drug PCL-PC film > drug PCL film ≈ plain PCL-PC film ≈ plain PCL film > drug suspension > control group (Figure 9).
Relative expression of messenger ribonucleic acid encoding A, tumor necrosis factor-alpha (TNF-α); B, transforming growth factor-beta (TGF-β); C, interleukin-1 (IL-1); D, interleukin-6 (IL-6); E, fibrinogen; and F, alpha-smooth muscle actin (α-SMA) in various treatment groups 2 weeks after surgery quantified by quantitative real-time reverse transcription polymerase chain reaction (n = 6; mean ± standard error of mean); (1) control untreated group; (2) drug suspension group; (3) plain polycaprolactone (PCL) film; (4) plain PCL-phosphatidylcholine (PC) film; (5) indomethacin-PCL film, and (6) indomethacin-PCL-PC film; * P < 0.05, ** P < 0.01 vs. control untreated group; # P < 0.05, ## P < 0.01 vs. drug suspension group
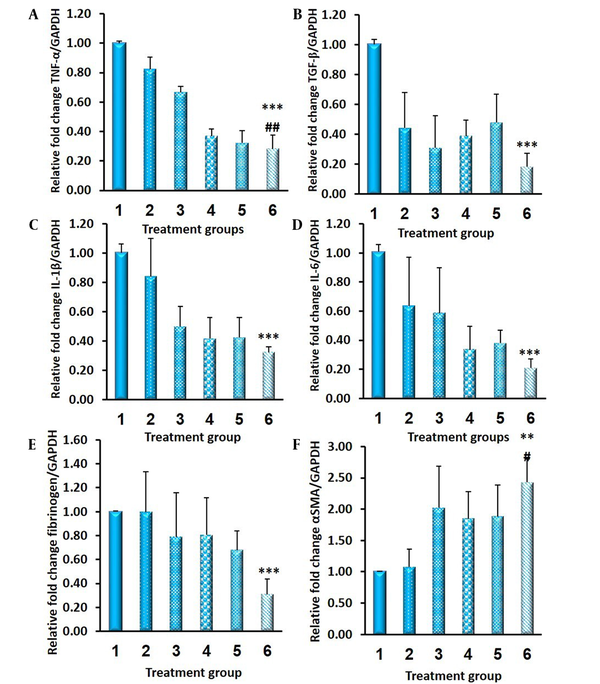
5. Discussion
Postoperative abdominal adhesions are among common challenging problems in surgery. Adhesions cause significant morbidity and serious complications, including intermittent cramps, chronic pain, female infertility, small bowel obstruction, and complicated additional operations (1, 2). In this context, considerable efforts have been focused on the prevention of adhesion formation by physical barriers (4, 5) and the administration of therapeutic agents (6, 7). The combination of these two strategies can more successfully manage postoperative adhesion.
The ultimate goal of anti-adhesion barriers is the reduction of clinical consequences that occur after surgery. Recent studies have suggested the partial prevention of adhesions after the use of some commercial barriers, such as SeprafilmTM (2, 25) and SurgiWrapTM (5, 26); however, the formulation designed in this study prevents adhesion due to the presence of indomethacin as an anti-inflammatory agent. Given the anti-inflammatory and anti-adhesion properties of indomethacin and phospholipids, along with the favorable potential characteristics of PCL films as a sustained drug delivery matrix and a biodegradable physical barrier, the present study hypothesized that implantable indomethacin sustained-release membrane composed of PC and PCL blend will prevent abdominal adhesion formation. In this regard, this study prepared indomethacin-eluting membranes and characterized the formulations regarding drug content, in vitro drug release kinetics, XRD, FTIR, and surface morphology. The anti-adhesion efficacy of this combined approach was compared to the untreated control, blank PCL and PCL-PC films, and drug suspension in a rat abdominal abrasion model.
Drug content is one of the important characterization parameters for any drug dosage form. The casting and drying method for film preparation might have inherent challenges in the achievement of thickness uniformity, which directly influences drug content per unit area and dosage uniformity (27). The incorporated drug amount in each formulation was quantified in at least three sites of drug-loaded films to estimate the uniform drug distribution in the films. On the contrary, in this study, as presented in Table 1, the standard error of mean values of film thickness were relatively low, which can be interpreted as homogeneous drug content.
The in vitro drug release rate is one of the critical parameters for predicting in vivo drug release kinetics and performance of implants. A reasonable prolonged drug release profile is the initial requirement of an appropriate anti-adhesive barrier membrane (28, 29). The incorporation of drugs into polymeric films can provide this demand (11, 30). The total drug release amount from PCL film casts was slower than from PCL-PC films (Figure 1). It seems that PCL better incorporated and retained indomethacin molecules than PC. As indomethacin, PCL, and PC possess very poor aqueous solubility, this observation can be explained by stronger Van der Waals and hydrophobic interactions between drug and PCL molecules. Drug release from PCL films was tri-phasic, with an initial release over 4 hours, followed by a second phase with a slower controlled release for about 1 week, and the third phase with a more sustained release rate. The PCL-PC films did not exhibit the third phase (Figure 1). The initial drug release could be attributed to the surface-bound drug or the porosity of films. The later sustained release phase might be due to drug diffusion through the matrix and the release of drug by the slow degradation of PCL.
The XRD and FTIR analyses are powerful tools for analyzing the crystalline or amorphous nature of the samples and any possible intermolecular interactions between drug and formulation components (31, 32). The XRD patterns of drug, PCL, and PC (Figure 2) in this study are in good agreement with those of previous reports (33-36). The disappearance of drug crystalline peaks in the XRD results of drug films suggested the loss of drug crystallinity by well loading into PCL and PCL-PC membranes in an amorphous state. The FTIR provides information on intermolecular interactions and structural changes by the features of band-shift and/or broadening in the spectra (32). The absence of interaction between the drug and components can be proved by the FTIR results (Figure 3).
The SEM and AFM analyses are very useful tools to determine the surface morphology of film samples. The AFM investigation can provide two-dimensional and three-dimensional images and was performed to validate the morphological properties obtained from SEM analysis (Figure 4). The pore-free structure of films observed by SEM and AFM can make them a promising barrier to the separation of the injured sites.
The anti-adhesion effect of sustained-release indomethacin membrane composed of a phospholipid and PCL blend was investigated in vivo by creating defects in both the cecum and peritoneum of rats. At the early stage after tissue injury, various proinflammatory mediators (e.g., TNF-α, TGF-β, IL-1, and IL-6) are released at the damaged peritoneum, and inflammatory responses promote tissue fibrosis (37, 38). Insufficient fibrinolysis in traumatized sites results in the deposition of extracellular matrix components and the formation of fibrinogen-rich adhesion tissue (1). Therefore, in this study, the levels of proinflammatory cytokines, fibrinogen, and α-SMA were quantified to examine the anti-adhesion efficacy of indomethacin films. Two weeks after implantation, intra-abdominal adhesions were observed and scored according to the adhesion grading system (Table 2) (22). The repair of peritoneal injury was examined using histopathological and SEM analyses (Figures 7 and 8).
The control group presented the highest adhesion level with dense and severe adhesions in the injured sites, damaged epithelium with infiltration of inflammatory cells, and lack of remesothelialization. The role of local delivery of indomethacin suspension in the inhibition of inflammation and fibrosis was validated by comparing the data from G1 and G2 groups. The NSAIDs inhibit cyclooxygenase (COX) enzymes, thereby reducing prostaglandin synthesis in the inflammatory cascade (39). Indomethacin with nonspecific and wider activity against COX isoenzymes showed superior anti-adhesion efficacy, compared to selective COX-2 inhibitors in pericardial adhesions in a pig model (12). Moreover, the in vivo results of plain films suggested that the application of a physical barrier device was not enough to provide the complete protection of postsurgical adhesion. Additionally, compared to PC-free implants, PC-enriched plain and drug-loaded formulations had lower adhesion grades reinforcing the anti-adhesion and anti-inflammatory effects of phospholipids on postoperative tissue adhesion as described by previous studies (40, 41). The aforementioned results showed that the controlled delivery of indomethacin, barrier properties of films, and PC enrichment had a role in the suppression of abdominal adhesion in the rat animal model by the complete recovery of injured abdominal wall without any fibrosis in drug-loaded PC and PCL blend film.
5.1. Conclusion
This study developed and characterized a sustained release polymeric-lipidic membrane to prevent postsurgical abdominal adhesion. The current study demonstrated the potent anti-inflammatory and anti-adhesion effects of indomethacin-eluting, PCL-PC membrane in a rodent cecal abrasion model by macroscopic scoring and histological, SEM, and PCR analyses. There was a significant suppression in adhesion severity and extent with the maximal efficacy observed in the combination of indomethacin, PC, and PCL in a drug-eluting film. Such a multifunctional barrier offers a practical approach for future clinical applications.
References
-
1.
Moris D, Chakedis J, Rahnemai-Azar AA, Wilson A, Hennessy MM, Athanasiou A, et al. Postoperative Abdominal Adhesions: Clinical Significance and Advances in Prevention and Management. J Gastrointest Surg. 2017;21(10):1713-22. [PubMed ID: 28685387]. https://doi.org/10.1007/s11605-017-3488-9.
-
2.
Beyene RT, Kavalukas SL, Barbul A. Intra-abdominal adhesions: Anatomy, physiology, pathophysiology, and treatment. Curr Probl Surg. 2015;52(7):271-319. [PubMed ID: 26258583]. https://doi.org/10.1067/j.cpsurg.2015.05.001.
-
3.
Chandel AKS, Shimizu A, Hasegawa K, Ito T. Advancement of Biomaterial-Based Postoperative Adhesion Barriers. Macromol Biosci. 2021;21(3). e2000395. [PubMed ID: 33463888]. https://doi.org/10.1002/mabi.202000395.
-
4.
Wang H, Sun W, Fu D, Shen Y, Chen YY, Wang LL. Update on biomaterials for prevention of epidural adhesion after lumbar laminectomy. J Orthop Translat. 2018;13:41-9. [PubMed ID: 29662790]. [PubMed Central ID: PMC5892378]. https://doi.org/10.1016/j.jot.2018.02.001.
-
5.
Li J, Feng X, Liu B, Yu Y, Sun L, Liu T, et al. Polymer materials for prevention of postoperative adhesion. Acta Biomater. 2017;61:21-40. [PubMed ID: 28780432]. https://doi.org/10.1016/j.actbio.2017.08.002.
-
6.
Imai A, Takagi H, Matsunami K, Suzuki N. Non-barrier agents for postoperative adhesion prevention: clinical and preclinical aspects. Arch Gynecol Obstet. 2010;282(3):269-75. [PubMed ID: 20232204]. https://doi.org/10.1007/s00404-010-1423-3.
-
7.
Alonso Jde M, Alves AL, Watanabe MJ, Rodrigues CA, Hussni CA. Peritoneal response to abdominal surgery: the role of equine abdominal adhesions and current prophylactic strategies. Vet Med Int. 2014;2014:279730. [PubMed ID: 24587939]. [PubMed Central ID: PMC3918701]. https://doi.org/10.1155/2014/279730.
-
8.
Lo HY, Kuo HT, Huang YY. Application of polycaprolactone as an anti-adhesion biomaterial film. Artif Organs. 2010;34(8):648-53. [PubMed ID: 20698842]. https://doi.org/10.1111/j.1525-1594.2009.00949.x.
-
9.
Hsu SH, Dai LG, Hung YM, Dai NT. Evaluation and characterization of waterborne biodegradable polyurethane films for the prevention of tendon postoperative adhesion. Int J Nanomedicine. 2018;13:5485-97. [PubMed ID: 30271142]. [PubMed Central ID: PMC6149831]. https://doi.org/10.2147/IJN.S169825.
-
10.
Frederiksen K, Guy RH, Petersson K. The potential of polymeric film-forming systems as sustained delivery platforms for topical drugs. Expert Opin Drug Deliv. 2016;13(3):349-60. [PubMed ID: 26609868]. https://doi.org/10.1517/17425247.2016.1124412.
-
11.
Tran TTD, Tran PHL. Controlled Release Film Forming Systems in Drug Delivery: The Potential for Efficient Drug Delivery. Pharmaceutics. 2019;11(6). [PubMed ID: 31226748]. [PubMed Central ID: PMC6630634]. https://doi.org/10.3390/pharmaceutics11060290.
-
12.
Alizzi AM, Summers P, Boon VH, Tantiongco JP, Thompson T, Leslie BJ, et al. Reduction of post-surgical pericardial adhesions using a pig model. Heart Lung Circ. 2012;21(1):22-9. [PubMed ID: 22078313]. https://doi.org/10.1016/j.hlc.2011.10.002.
-
13.
Bayhan Z, Zeren S, Kocak FE, Kocak C, Akcilar R, Kargi E, et al. Antiadhesive and anti-inflammatory effects of pirfenidone in postoperative intra-abdominal adhesion in an experimental rat model. J Surg Res. 2016;201(2):348-55. [PubMed ID: 27020818]. https://doi.org/10.1016/j.jss.2015.11.033.
-
14.
Mao Y, Chen M, Guidoin R, Li Y, Wang F, Brochu G, et al. Potential of a facile sandwiched electrospun scaffold loaded with ibuprofen as an anti-adhesion barrier. Mater Sci Eng C Mater Biol Appl. 2021;118:111451. [PubMed ID: 33255038]. https://doi.org/10.1016/j.msec.2020.111451.
-
15.
Gupta A, Bah M. NSAIDs in the Treatment of Postoperative Pain. Curr Pain Headache Rep. 2016;20(11):62. [PubMed ID: 27841015]. https://doi.org/10.1007/s11916-016-0591-7.
-
16.
Harirforoosh S, Asghar W, Jamali F. Adverse effects of nonsteroidal antiinflammatory drugs: an update of gastrointestinal, cardiovascular and renal complications. J Pharm Pharm Sci. 2013;16(5):821-47. [PubMed ID: 24393558]. https://doi.org/10.18433/j3vw2f.
-
17.
Garcia-Rayado G, Navarro M, Lanas A. NSAID induced gastrointestinal damage and designing GI-sparing NSAIDs. Expert Rev Clin Pharmacol. 2018;11(10):1031-43. [PubMed ID: 30139288]. https://doi.org/10.1080/17512433.2018.1516143.
-
18.
Bajaj G, Yeo Y. Drug delivery systems for intraperitoneal therapy. Pharm Res. 2010;27(5):735-8. [PubMed ID: 20198409]. [PubMed Central ID: PMC2854252]. https://doi.org/10.1007/s11095-009-0031-z.
-
19.
Jantrawut P, Chaiwarit T, Jantanasakulwong K, Brachais CH, Chambin O. Effect of Plasticizer Type on Tensile Property and In Vitro Indomethacin Release of Thin Films Based on Low-Methoxyl Pectin. Polymers (Basel). 2017;9(7). [PubMed ID: 30970971]. [PubMed Central ID: PMC6432188]. https://doi.org/10.3390/polym9070289.
-
20.
Hoffmann EM, Breitenbach A, Breitkreutz J. Advances in orodispersible films for drug delivery. Expert Opin Drug Deliv. 2011;8(3):299-316. [PubMed ID: 21284577]. https://doi.org/10.1517/17425247.2011.553217.
-
21.
National Institutes of Health. Guide for the care and use of laboratory animals. Washington D.C., USA: National Academies; 1985.
-
22.
Liu B, Zhang Q, Wu X, Fu Y, Wang H, Guan Y, et al. Effect of Bletilla striata on the Prevention of Postoperative Peritoneal Adhesions in Abrasion-Induced Rat Model. Evid Based Complement Alternat Med. 2019;2019:9148754. [PubMed ID: 31281407]. [PubMed Central ID: PMC6590513]. https://doi.org/10.1155/2019/9148754.
-
23.
Badri W, Miladi K, Robin S, Viennet C, Nazari QA, Agusti G, et al. Polycaprolactone Based Nanoparticles Loaded with Indomethacin for Anti-Inflammatory Therapy: From Preparation to Ex Vivo Study. Pharm Res. 2017;34(9):1773-83. [PubMed ID: 28527126]. https://doi.org/10.1007/s11095-017-2166-7.
-
24.
Tantipolphan R, Rades T, McQuillan AJ, Medlicott NJ. Adsorption of bovine serum albumin (BSA) onto lecithin studied by attenuated total reflectance Fourier transform infrared (ATR-FTIR) spectroscopy. Int J Pharm. 2007;337(1-2):40-7. [PubMed ID: 17240095]. https://doi.org/10.1016/j.ijpharm.2006.12.021.
-
25.
Diamond MP, Burns EL, Accomando B, Mian S, Holmdahl L. Seprafilm(R) adhesion barrier: (1) a review of preclinical, animal, and human investigational studies. Gynecol Surg. 2012;9(3):237-45. [PubMed ID: 22837732]. [PubMed Central ID: PMC3401296]. https://doi.org/10.1007/s10397-012-0741-9.
-
26.
Gruber-Blum S, Petter-Puchner AH, Brand J, Fortelny RH, Walder N, Oehlinger W, et al. Comparison of three separate antiadhesive barriers for intraperitoneal onlay mesh hernia repair in an experimental model. Br J Surg. 2011;98(3):442-9. [PubMed ID: 21254024]. https://doi.org/10.1002/bjs.7334.
-
27.
Zhang L, Alfano J, Race D, Dave RN. Zero-order release of poorly water-soluble drug from polymeric films made via aqueous slurry casting. Eur J Pharm Sci. 2018;117:245-54. [PubMed ID: 29499350]. [PubMed Central ID: PMC5899018]. https://doi.org/10.1016/j.ejps.2018.02.029.
-
28.
Li L, Zheng X, Fan D, Yu S, Wu D, Fan C, et al. Release of celecoxib from a bi-layer biomimetic tendon sheath to prevent tissue adhesion. Mater Sci Eng C Mater Biol Appl. 2016;61:220-6. [PubMed ID: 26838844]. https://doi.org/10.1016/j.msec.2015.12.028.
-
29.
Kao CW, Lee D, Wu MH, Chen JK, He HL, Liu SJ. Lidocaine/ketorolac-loaded biodegradable nanofibrous anti-adhesive membranes that offer sustained pain relief for surgical wounds. Int J Nanomedicine. 2017;12:5893-901. [PubMed ID: 28860755]. [PubMed Central ID: PMC5566388]. https://doi.org/10.2147/IJN.S140825.
-
30.
Schlesinger E, Johengen D, Luecke E, Rothrock G, McGowan I, van der Straten A, et al. A Tunable, Biodegradable, Thin-Film Polymer Device as a Long-Acting Implant Delivering Tenofovir Alafenamide Fumarate for HIV Pre-exposure Prophylaxis. Pharm Res. 2016;33(7):1649-56. [PubMed ID: 26975357]. [PubMed Central ID: PMC4892981]. https://doi.org/10.1007/s11095-016-1904-6.
-
31.
Clas SD, Dalton CR, Hancock BC. Differential scanning calorimetry: applications in drug development. Pharm Sci Technol Today. 1999;2(8):311-20. [PubMed ID: 10441275]. https://doi.org/10.1016/s1461-5347(99)00181-9.
-
32.
Chadha R, Bhandari S. Drug-excipient compatibility screening--role of thermoanalytical and spectroscopic techniques. J Pharm Biomed Anal. 2014;87:82-97. [PubMed ID: 23845418]. https://doi.org/10.1016/j.jpba.2013.06.016.
-
33.
Koranne S, Thakral S, Suryanarayanan R. Effect of Formulation and Process Parameters on the Disproportionation of Indomethacin Sodium in Buffered Lyophilized Formulations. Pharm Res. 2018;35(1):21. [PubMed ID: 29305664]. https://doi.org/10.1007/s11095-017-2310-4.
-
34.
Colombo M, Minussi C, Orthmann S, Staufenbiel S, Bodmeier R. Preparation of amorphous indomethacin nanoparticles by aqueous wet bead milling and in situ measurement of their increased saturation solubility. Eur J Pharm Biopharm. 2018;125:159-68. [PubMed ID: 29371046]. https://doi.org/10.1016/j.ejpb.2018.01.013.
-
35.
Freag MS, Saleh WM, Abdallah OY. Self-assembled phospholipid-based phytosomal nanocarriers as promising platforms for improving oral bioavailability of the anticancer celastrol. Int J Pharm. 2018;535(1-2):18-26. [PubMed ID: 29102699]. https://doi.org/10.1016/j.ijpharm.2017.10.053.
-
36.
Rong HJ, Chen WL, Guo SR, Lei L, Shen YY. PCL films incorporated with paclitaxel/5-fluorouracil: Effects of formulation and spacial architecture on drug release. Int J Pharm. 2012;427(2):242-51. [PubMed ID: 22349052]. https://doi.org/10.1016/j.ijpharm.2012.02.007.
-
37.
Zhang Y, Li X, Zhang Q, Li J, Ju J, Du N, et al. Berberine hydrochloride prevents postsurgery intestinal adhesion and inflammation in rats. J Pharmacol Exp Ther. 2014;349(3):417-26. [PubMed ID: 24676878]. https://doi.org/10.1124/jpet.114.212795.
-
38.
Tian L, Li H, Li Y, Liu K, Sun Y, Cong Z, et al. A Combination of Chitosan, Cellulose, and Seaweed Polysaccharide Inhibits Postoperative Intra-abdominal Adhesion in Rats. J Pharmacol Exp Ther. 2018;364(3):399-408. [PubMed ID: 29263242]. https://doi.org/10.1124/jpet.117.244400.
-
39.
Ricciotti E, FitzGerald GA. Prostaglandins and inflammation. Arterioscler Thromb Vasc Biol. 2011;31(5):986-1000. [PubMed ID: 21508345]. [PubMed Central ID: PMC3081099]. https://doi.org/10.1161/ATVBAHA.110.207449.
-
40.
Tsaousi G, Stavrou G, Fotiadis K, Kotzampassi K, Kolios G. Implementation of phospholipids as pharmacological modalities for postoperative adhesions prevention. Eur J Pharmacol. 2019;842:189-96. [PubMed ID: 30391744]. https://doi.org/10.1016/j.ejphar.2018.10.054.
-
41.
Fotiadis K, Filidou E, Arvanitidis K, Valatas V, Stavrou G, Basdanis G, et al. Intraperitoneal application of phospholipids for the prevention of postoperative adhesions: a possible role of myofibroblasts. J Surg Res. 2015;197(2):291-300. [PubMed ID: 25976855]. https://doi.org/10.1016/j.jss.2015.04.036.