Abstract
Background:
Diabetes mellitus (DM) is frequently linked with problems of several organ systems, including retinopathy, neuropathy, and nephropathy. Additionally, patients have changes in sexual functioning, such as decreased libido and fertility. Vincamine, a monoterpenoid indole alkaloid, has hypoglycemic and antioxidant effects.Objectives:
This research assessed the impact of vincamine on testicular dysfunction in alloxan-induced male rats by measuring fasting blood glucose, oxidative stress, seminal analysis, and histological examination of the testis.Methods:
Wister-albino male rats were randomized into the following groups at random: Untreated-healthy, untreated-DM, vincamine-treated (20 mg/kg) DM, vincamine-treated (40 mg/kg) DM, and clomiphene-treated DM (5 mg/kg). On day 14, rats were sacrificed, and semen/blood samples were collected. Sperm count, motility, and morphological abnormalities were noted by microscopic examination. The testis was examined histopathologically and assessed using Johnsen’s score.Results:
Compared with the untreated diabetic group, a dosage of 40 mg/kg vincamine generate a significant reduction in fasting blood sugar (FBG). Compared with the untreated diabetic group, the vincamine-treated rats produced greater plasma testosterone levels and Johnsen scores. In the vincamine 20 mg/kg group, sperm concentration was higher than in the vincamine 40 mg/kg group.Conclusions:
It is possible that vincamine has a potential preventive effect against diabetes-related reproductive problems attributable to its antioxidant activity and capacity to restore testicular steroidogenesis.Keywords
1. Background
Hyperglycemia is caused by diabetes mellitus (DM), insulin secretion (DM-type I), or insulin activity (DM-type II) deficiency, the prevalence of which is estimated to be 8.3% worldwide and 382 million people worldwide have this disease (1). It causes various systemic abnormalities such as renal disorders, reproductive/cardiovascular, neuropathies, and ophthalmopathies (2). The majority of diabetic patients are infertile or subinfertile as a result of testicular problems, decreased libido, ejaculation, erection, and spermatogenesis problems. It has also been shown that serum testosterone levels are impaired, and there are testicular and epididymal structural abnormalities in experimental DM models (1).
Although there is a link between the overproduction of reactive oxygen species (ROS) caused by hyperglycemia and testicular dysfunction, the sexual and testicular dysfunctions associated with diabetes have not been clarified yet.
Decreased insulin production alters spermatogenesis by altering blood levels of FSH and LH in diabetic rats and causes a significant decrease in testosterone levels (2). Reactive oxygen species can affect steroidogenic enzymes by reducing the synthesis of steroid hormones. Decreased insulin production alters spermatogenesis by altering blood levels of FSH and LH in diabetic rats. Decreased insulin in diabetes causes a significant decrease in testosterone levels.
Spermatogenesis in the male reproductive system is a hormone-dependent complex cell development process, including apoptosis, in which spermatogonia turn into mature sperm. Testicular stem cells, called spermatozoa, form spermatozoa by successive differentiation of mitotic, meiotic, and haploid spermatids, respectively, under the influence of steroid hormones, and this process occurs. It has a complex biological content in which spermatozoa are produced.
1.1. Male Fertility
Testicular structures and structures that play an important role in spermatogenesis: Seminiferous tubules, peritubular myoid bodies, Leydig cells, Sertoli cells, germ cells, and blood-testicular barrier. Adult spermatogonia type A and type B spermatogonia are divided into two (3).
Fertilization depot, depends on the glucose uptake of the sperm cell. Tyrosine phosphorylation is dependent on important changes such as take-off movements and hyperactivity. Glucose is not only for the continuation of spermatogenesis in vivo; it is very important for sperm reproductive capacity.
Spermatogonial stem junctions (SSCs) are adult tissue stem cells found in the testicles and are important for maintaining the stem cell pool in post-puberty males to continue producing sperm for self-renewal and differentiation. Similar to other adult tissue stem cells, SSCs are rare, accounting for only 0.03% of total germ formation in mice. In the mouse, spermatogonia consist of primordial germ cells (PGCs) first identified in the extraembryonic mesoderm on an embryonic day. Primordial germ cells migrate from the allantois along the hindgut and settle in the genital prominence. There, PGCs grow continuously and then enter G0/G1 arrest. As the proliferating PGCs are surrounded by Sertoli cells, the basic structure of the testicular cords is formed (4).
Clomiphene citrate is an orally active fertility drug called 1-(p-(β-diethyl aminoethoxy) phenyl)-1,2-diphenyl chloroethylene, a racemic mixture of two non-steroidal isoforms. Clomiphene citrate selectively stimulates adrenal androgen biosynthesis. Stimulated steroids can be converted to testosterone. In addition, it stimulates Sertoli cells that produce spermatocytes, which play an important role in supplying iron to developing germ cells and can increase seminal transferrin levels. As a result of all these, clomiphene citrate contributes to the initiation and maintenance of spermatogenesis by increasing testosterone, LH, and endogenous FSH levels. In the absence of any organ pathology, this increase in endogenous gonadotropins contributes to improving sperm functions (motility, number, morphology) up to a point (5).
An increasing number of phytochemicals such as saponins, polysaccharides, alkaloids, glycosides, flavonoids, terpenoids, and terpenoids are being extracted from medicinal plants and have been found to have anti-diabetic properties (6).
Vincamine is defined as a monoterpenoid indole alkaloid, extracted from Vinca rosea named Catharanthus roseus. According to the literature, vincamine has several biological effects along with anticoagulant, memory-boosting, nootropic, hypoglycemizing, hypolipidemizing, vasodilator, and antioxidant action (7). It has been daily administered at doses ranging from 40 - 80 mg for more than a month without causing any negative effects (8).
Diabetes mellitus-induced autooxidation of glucose and production of superoxide, glycation of proteins and formation of advanced glycation end (AGE) products, polyol pathway activity, increased protein kinase C activity, and hexosamine pathway (9) are associated with testicular injuries and excessive cellular ROS related to its production (10). High levels of fatty acids with reduced antioxidant levels make mammalian spermatozoa vulnerable to oxidative damage.
In a study with vincamine, it was shown that vincamine induces the formation of ROS in A549 cells in a dose-dependent manner.
Some forms of vincamine have also been found to modulate insulin and glucagon secretion (11, 12). This highlights the use of vincamine in DM to prevent diabetes-related hyperglycemia, hyperlipidemia, and oxidative damage.
Changes in the male reproductive system have been widely reported in diabetic individuals. The blood-testicular barrier is a tight blood-tissue barrier that divides the seminiferous tubule epithelium into adluminal and basal tearing, protects the adluminal environment, and creates the microenvironment required for functional sperm formation (13). Claudins are one of the important molecules mediating tight junction permeability and epithelial barrier function. Whether the integrity of the barrier is preserved or not can be interpreted by examining the expression level of claudin molecules. In a previous study, it was shown that diabetes reduces the expression level of claudin-11 (CLDN-11) (14), and also previous studies have shown that excessive hydroxyl radicals and advanced glycation end products caused by hyperglycemia may further increase ROS production in patients with diabetes, leading to increased oxidative stress and exacerbation of sperm loss (15).
2. Objectives
The study was carried out to investigate whether vincamine may have a beneficial effect on the relief of testicular injury in alloxan-diabetic rats, as well as whether inflammation, oxidative stress, and apoptosis could have played a role in the process.
3. Methods
3.1. Chemicals
Clomiphene and diagnostic kits were purchased from Sigma, Germany. Vincamine and alloxan were purchased from Sigma, St.Lous. The other compounds employed in the research were analytical grade and originate from well-known sources.
3.2. Experimental Animals
Wistar-albino rats weighing 250 - 270 g were gathered from MEDITAM, Istanbul Medipol University. Animals were hosted in cages with unlimited water and food, at 25 ± 2°C. Before conducting the trials, the rats were exposed to the environment for at least one week.
3.3. Induction of Experimental Diabetes
Fasting blood sugar (FBG) levels were assessed in all rats following a 12-hour fast with free access to water. Alloxan monohydrate (150 mg/kg) dissolved in sterile saline sodium chloride (%0.9), pH 7, was intraperitoneally injected into rats for induction of hyperglycemia. The solution should be prepared soon before administration and should be fresh. To avoid time-dependent alloxan induced-hypoglycemia, glucose solution (5%) was given in water bottles for 24 hours. After 5 days of alloxan administration, the rats were considered to have hyperglycemia when FBG levels were above 250 mg/dL (16).
3.4. Experimental Design
Animals were unsystematically separated into five groups (n = 8): Untreated-healthy, untreated-DM, vincamine-treated (20 mg/kg) DM, vincamine-treated (40 mg/kg) DM, and clomiphene-treated DM (5 mg/kg). After the diagnosis of diabetes, vincamine was dissolved in 0.5% dimethyl sulfoxide (DMSO) and administered orally by gavage (0.1 mL) once daily for 14 days after the rats were diagnosed with diabetes.
3.5. Biochemical Analyses of Serum Parameters
Following the last dose, animals were fasted overnight and sacrificed under isoflurane anesthesia. Blood was drawn and centrifuged for 10 minutes before being utilized for biochemical testing. The content of testosterone in the blood was detected utilizing an enzyme-linked immunosorbent assay (ELISA) kit (17). The FBG level was determined by a glucometer (Accu-Chek, Germany) utilizing the glucose oxidase technique, and the results were analyzed. FBG was found from blood sampling from the lateral tail vein of the rats.
3.6. Sperm Concentration and Motility Analysis
The adipose tissue around the epididymis was removed to isolate and clean the epididymis. The cauda section of the epididymis was dissected and put in a Tyrode buffer that had been pre-warmed to 37°C before being used. Following a 15-mins waiting period, sperm were inspected under a microscope for motility and concentration.
(1) Sperm concentration: 10 μL of semen sample was located in the Macler® counting chamber (Sefi Medical Instruments, Israel). One-hundred frames will be counted under a phase contrast microscope with a 20 X objective, and the result will be expressed in mil/mL.
(2) Sperm total motility: To assess the total motion of sperm as well as progressive motion, sperm were separated into four groups, and counted at least 100 sperm under a 40 X objective under a phase contrast microscope
(3) A to D motility: Rapid, slow, non, progressive motility to immobility. The results were expressed in terms of million/mL.
3.7. Histopathological Evaluation
Each testis was extracted post-mortem from rats. To avoid histologic alterations caused by ischemia damage, tissue was obtained within 15 minutes after death. One testis per animal was preserved in Bouin’s solution and the other was frozen for immunohistochemistry. Tissue samples were then dehydrated using graduated ethanol alcohols (%70, 90, 96, and 100) and xylene before being embedded in paraffin to create tissue blocks. Slides were obtained by cutting sample blocks into 5 μm sections using a microtome (Thermo Scientific, HM340E). Slides were dyed with eosin and hematoxylin. Nikon® Eclipse was used to inspect them. As previously reported by Johnsen, sections were scored quantitatively to determine the extent of histological alterations associated with seminiferous tubule damage (18). A score of 1 to 10 was allocated to the testicular biopsies based on this scoring method (10: Perfect tubules, spermatogenesis; 1: No cells at all within the tubules) (19).
3.8. Immunohistochemical Analysis
Sections taken with a 5 μm thick Cryomicrotome (CM1950, Leica, Germany) on positive slides were fixed with 4% paraformaldehyde for 20 minutes and soaked with PBS. To prevent endogenous peroxidase activity, it was incubated at 25°C with 3% H2O2 for 10 minutes.
Sections were then heated in 0.01 mol/L citrate buffer for 15 minutes for microwaving for antigen retrieval. Two percent blocking solution containing 10% NGS was dripped onto the sections and waited for 1 hour for blocking. Immune reactivity of specific protein in sections incubated overnight at +4°C in anti-CLDN-11 (1: 100, Thermo Fischer 36 - 4500), Mouse and Rabbit Specific HRP (ABC) Detection IHC Kit (Abcam, ab93677) detected using. Browning of the DAB chromogen (Abcam, ab64238) was observed within 3 minutes after washing with PBS. Hematoxylin (Bio-Optica 05-06002E) was then applied for core staining. Afterward, the sections were cleaned under running tap water for 10 minutes and dried in alcohol series. Images were captured with Nikon Eclipse by coating with Biomount (Bio-Optica 1611).
3.9. Statistical Analysis
The GraphPad Prism 7.0 tool was used to analyze the data. All results were taken as mean ± standard error of the mean (SEM). All statistical comparisons were made with one-way ANOVA and then Dunnett tests, and the results were considered significant at P < 0.05.
4. Results
4.1. Biochemical Analyses of Serum Parameters
The effectiveness of vincamine on FBG levels in diabetic rats is presented in Figure 1. Alloxan-induced diabetic rats displayed a significant rise in blood sugar levels compared to the untreated-healthy group as a typical feature of hyperglycemia.
Effect of vincamine on fasting blood sugar level in diabetic and nondiabetic rats. All the values are represented in mean ± standard error of the mean (* P).
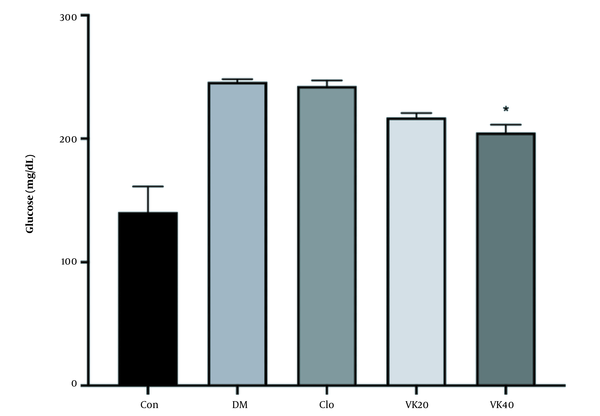
An oral medication of 40 mg/kg vincamine for 14 days considerably (P < 0.05) reduced high FBG concentrations as compared to the untreated DM. The results also showed vincamine decreased FBG concentrations as the applied dose increased. The clomiphene-treated group did not produce any meaningful effect on FBG concentrations.
According to the data described in Figure 2, there is a significant decrease in testosterone levels of diabetic rats compared to the control group (P < 0.05). In addition, clomiphene-treated diabetic rats significantly increased testosterone levels (P < 0.001). In addition, treatment of diabetic rats with 20 mg/kg (P < 0.01) and 40 mg/kg (P < 0.001) vincamine significantly prevented diabetes-induced serum testosterone reduction.
The effect of vincamine on serum testosterone levels of diabetic rats and non-diabetic rats. All the values are represented in mean ± standard error of the mean ** pï 1/4 œ 0.01, *** pï 1/4 œ 0.001 vs. diabetic control. Con: Untreated-healthy control, DM: Untreated diabetic control, VK20: Vincamine-treated (20 mg/kg) diabetics, VK40: Vincamine-treated (40 mg/kg) diabetics, Clo: Clomiphene treated diabetics (5mg/kg).
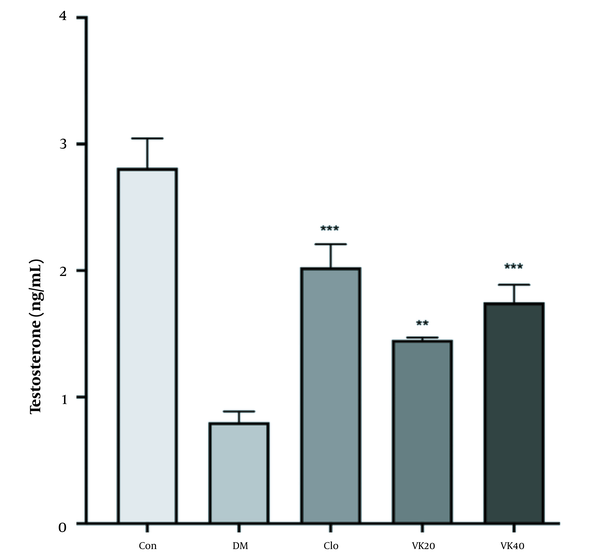
4.2. Sperm Parameters
As a result of the evaluation of various sperm parameters, it was revealed that there was no statistically significant difference between the tested groups in terms of sperm concentration and total motility. Vincamin 20 mg/kg (VK20) administration increased sperm concentration (Figure 3).
Effect of vincamine on sperm concentration. Con: Untreated-healthy control, DM: Untreated-diabetic control, VK20: Vincamine-treated (20 mg/kg) diabetics, VK40: Vincamine-treated (40 mg/kg) diabetics, Clo: Clomiphene-treated diabetics (5mg/kg). All the values are represented in mean ± standard error of the mean (* P).
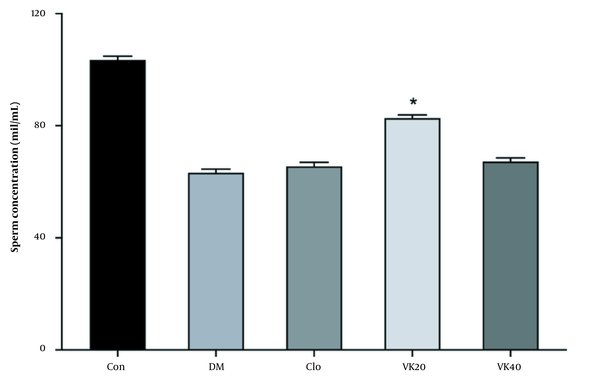
4.3. Histopathological Evaluation
Histological findings were evaluated for each group, Johnsen scoring, and histopathological evaluation are shown in Figure 4, respectively. In the control group, normal morphology was observed in the germinal epithelium in the seminiferous tubules and the interstitial area. In the DM group, the seminiferous epithelium was thinner when compared to the control group, and vacuolization occurred between seminiferous epithelium. Openings were seen in the interstitial area. The morphology of the clomiphene group was similar to the control group. Improvements in the germinal epithelial layer and an increase in its number were observed in the clomiphene and VK20 groups compared to the diabetic group. Morphologies in the Vincamin 40 mg/kg (VK40) group were similar to the seminal tubule morphology in the control group. However, it was observed that the number of tubules was higher in the VK20 group, showing normal germinal epithelial morphology, compared to the VK40 group.
A and B, Normal seminiferous tubule morphology (*) and normal germinal cell layers in the seminiferous tubule epithelium in the control group; C and D, Vacuolization in the seminiferous tubules (ïƒ) and a decrease in the number of germinal epithelial layers in the seminiferous tubules in the diabetes mellitus (DM) group; E and F, In the clomiphene group, morphology was similar to the control group in the seminiferous tubules (*) and normal germinal cell layers in the seminiferous tubule epithelium. Decreased openings in the interstitial area; G and H, Normal seminiferous tubule morphology (*) and normal germinal cell layers in the seminiferous tubule epithelium; I and J, Seminiferous tubule morphology (*) was similar to the control group and near-normal organization in the number of germinal epithelial layers (A, C, E, G, I: 20X; B, D, F, H, J: 40X).
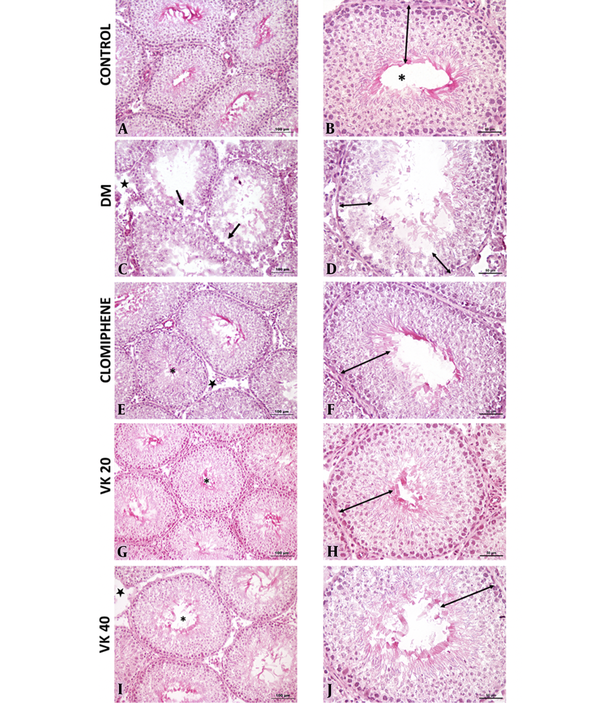
4.4. Immunohistochemical Analysis
The CLDN-11 expression, which was located at the blood-testis barrier between neighbor Sertoli cells, was imaged with the light microscope. The localization of CLDN-11 in each group showed in Figure 5.
Immunolocalization of the claudin-11 in the testes of rats in each group. Immunostaining was seen positive with DAB brown along with blue counter stained with hematoxylin (original magnification X400).
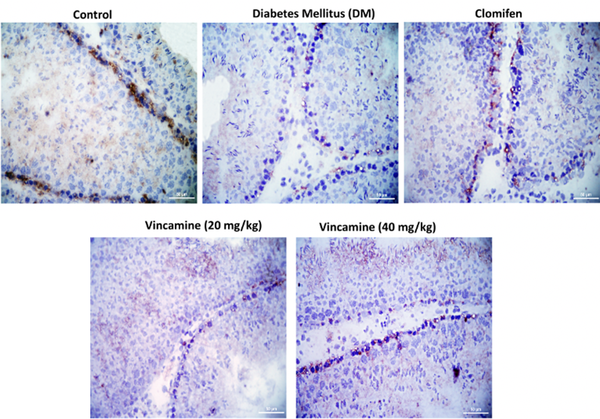
5. Discussion
While reproduction is essential for the long-term survival of humanity, fertility rates have decreased due to various lifestyle and environmental variables (2). Diabetes mellitus lowers testosterone levels while increasing gonadotropin levels. Both in vivo and in vitro evidence suggests that these alterations are linked to a steroidogenetic abnormality in Leydig cells. In addition, diabetes-related neuropathy can cause tonia of seminal vesicles, bladder, and urethra (20).
Natural components have been demonstrated to play an essential impact in the prevention of human illnesses in epidemiological investigations. Among them, vincamine has been reported to have therapeutic potential for cerebral metabolic and circulatory disorders (21). This paper was the first research about the potential reaction of vincamine on an alloxan-induced diabetic rat with spermatogenic dysfunction.
Clomiphene citrate is a non-steroidal fertility medicine that stimulates testosterone and sperm production when taken orally. Several studies reported that clopmiphene citrate treatment improved testosterone levels and spermatogenesis in infertile and hypogonadal men (22). Clomiphene could be applied to improve sperm parameters in clinical therapy by binding to the estrogen receptor and suppressing normal oestrogenic negative feedback on the hypothalamus-pituitary and hence spermatogenesis. This is in line with the data of this research, which found that clomiphene enhanced sperm parameters in diabetic rats. Thus, clomiphene exerted a beneficial controlled effect on the spermatogenic dysfunction observed in diabetes testes, as a result of the findings (23).
The findings of the present review seem to suggest that FBC concentrations were significantly reduced after administration of 20 mg/kg and 40 mg/kg of vincamine compared with the diabetic control group. Nandini and Naik showed that oral vincamine (20 and 30 mg/kg) treatment for one month resulted in a significant reduction in APG concentrations in diabetic rats, similar to the reduction observed in glibenclamide-treated animals (7). The results are in line with previous studies, which may indicate that vincamine’s ability to lower blood sugar levels in diabetic mice may help reduce the risk of abnormal spermatozoa and oxidative stress in sperm.
It can be said that the increase in semen concentration observed in the VK20 group may be related to the high levels of reproductive hormones such as testosterone, which directly support the spermatogenesis process. It seems that the increase observed in semen concentration in addition to testosterone level, may be due to increased testicular antioxidant defense and epididymal sperm maturation, which are effective in suppressing oxidative stress (24).
One of the primary causes of the onset of diabetic spermatogenic diseases is oxidative stress. As a result of the sexual germ cells being affected by oxidative stress, their differentiation and division are disrupted, reducing the number of spermatogonia, primary and secondary spermatocytes, spermatids, and mature spermatozoa in the basement membrane.
Spermatogenesis relies heavily on testosterone as the primary androgen, and its enhancement has a considerable impact on the number and quality of sperm (25). Reactive oxygen species plays an important role in the pathogenesis of diabetes-related male infertility. In certain pathological conditions, excessive accumulation of ROS can relatively induce autophagy (26). In this study, it was observed that testosterone levels in diabetic rats were much lower than in the control group. In addition, it seems that vincamine has been shown to significantly inhibit the reduction of serum testosterone levels in diabetic rats. It has been proven in previous studies that excessive production and accumulation of ROS in the testis causes autophagy (27). In the results obtained while investigating the causes of abnormal autophagy, apoptosis, decreased testosterone levels, abnormalities in sperm cell differentiation, and damage to the blood-testis barrier were observed in Sertoli cells (28).
Additionally, in vitro and in vivo investigations have shown that IL-1 and TNF- hindered Leydig cell activity, hence inhibiting testosterone synthesis produced by LH (29).
In the histopathological examination, significant testicular deterioration and a significant decrease in testicular lesion score were found in the diabetes control group in our study. It can be said that the testicular histological image and lesion score of the group treated with vincamine improved significantly. This shows us that controlling hyperglycemia and reducing testicular oxidative stress may have contributed to this improvement.
In conclusion, vincamine was reported to improve antidiabetic and testosterone in diabetic rats. These actions show us that they can be explained, at least in part, by its antioxidant and anti-inflammatory properties, which may be a valid target for the treatment or prevention of testicular reproductive damage.
References
-
1.
Maresch CC, Stute DC, Alves MG, Oliveira PF, de Kretser DM, Linn T. Diabetes-induced hyperglycemia impairs male reproductive function: a systematic review. Hum Reprod Update. 2018;24(1):86-105. [PubMed ID: 29136166]. https://doi.org/10.1093/humupd/dmx033.
-
2.
Cheng Y, Yang Z, Shi J, Yang J, Zhao J, He Y, et al. Total flavonoids of Epimedium ameliorates testicular damage in streptozotocin-induced diabetic rats by suppressing inflammation and oxidative stress. Environ Toxicol. 2020;35(2):268-76. [PubMed ID: 31696645]. https://doi.org/10.1002/tox.22864.
-
3.
Fayomi AP, Orwig KE. Spermatogonial stem cells and spermatogenesis in mice, monkeys and men. Stem Cell Res. 2018;29:207-14. [PubMed ID: 29730571]. [PubMed Central ID: PMC6010318]. https://doi.org/10.1016/j.scr.2018.04.009.
-
4.
Ohbo K, Yoshida S, Ohmura M, Ohneda O, Ogawa T, Tsuchiya H, et al. Identification and characterization of stem cells in prepubertal spermatogenesis in mice. Dev Biol. 2003;258(1):209-25. [PubMed ID: 12781694]. https://doi.org/10.1016/s0012-1606(03)00111-8.
-
5.
Delu A, Kiltz RJ, Kuznetsov VA, Trussell JC. Clomiphene citrate improved testosterone and sperm concentration in hypogonadal males. Syst Biol Reprod Med. 2020;66(6):364-9. [PubMed ID: 33043679]. https://doi.org/10.1080/19396368.2020.1822457.
-
6.
Ghosh S, Chowdhury S, Das AK, Sil PC. Taurine ameliorates oxidative stress induced inflammation and ER stress mediated testicular damage in STZ-induced diabetic Wistar rats. Food Chem Toxicol. 2019;124:64-80. [PubMed ID: 30496779]. https://doi.org/10.1016/j.fct.2018.11.055.
-
7.
Nandini HS, Naik PR. Antidiabetic, antihyperlipidemic and antioxidant effect of Vincamine, in streptozotocin-induced diabetic rats. Eur J Pharmacol. 2019;843:233-9. [PubMed ID: 30496743]. https://doi.org/10.1016/j.ejphar.2018.11.034.
-
8.
Al-Rashed S, Baker A, Ahmad SS, Syed A, Bahkali AH, Elgorban AM, et al. Vincamine, a safe natural alkaloid, represents a novel anticancer agent. Bioorg Chem. 2021;107:104626. [PubMed ID: 33450545]. https://doi.org/10.1016/j.bioorg.2021.104626.
-
9.
Atta MS, Almadaly EA, El-Far AH, Saleh RM, Assar DH, Al Jaouni SK, et al. Thymoquinone Defeats Diabetes-Induced Testicular Damage in Rats Targeting Antioxidant, Inflammatory and Aromatase Expression. Int J Mol Sci. 2017;18(5). [PubMed ID: 28448463]. [PubMed Central ID: PMC5454832]. https://doi.org/10.3390/ijms18050919.
-
10.
Arkali G, Aksakal M, Kaya SO. Protective effects of carvacrol against diabetes-induced reproductive damage in male rats: Modulation of Nrf2/HO-1 signalling pathway and inhibition of Nf-kB-mediated testicular apoptosis and inflammation. Andrologia. 2021;53(2). e13899. [PubMed ID: 33242925]. https://doi.org/10.1111/and.13899.
-
11.
Rodriguez-Diaz R, Dando R, Huang YA, Berggren PO, Roper SD, Caicedo A. Real-time detection of acetylcholine release from the human endocrine pancreas. Nat Protoc. 2012;7(6):1015-23. [PubMed ID: 22555241]. [PubMed Central ID: PMC3538842]. https://doi.org/10.1038/nprot.2012.040.
-
12.
Otter S, Lammert E. Exciting Times for Pancreatic Islets: Glutamate Signaling in Endocrine Cells. Trends Endocrinol Metab. 2016;27(3):177-88. [PubMed ID: 26740469]. https://doi.org/10.1016/j.tem.2015.12.004.
-
13.
Cheng CY, Mruk DD. The blood-testis barrier and its implications for male contraception. Pharmacol Rev. 2012;64(1):16-64. [PubMed ID: 22039149]. [PubMed Central ID: PMC3250082]. https://doi.org/10.1124/pr.110.002790.
-
14.
Korejo NA, Wei Q, Zheng K, Mao D, Korejo RA, Shah AH, et al. Contemporaneous effects of diabetes mellitus and hypothyroidism on spermatogenesis and immunolocalization of Claudin-11 inside the seminiferous tubules of mice. BMC Dev Biol. 2018;18(1):15. [PubMed ID: 29940839]. [PubMed Central ID: PMC6019809]. https://doi.org/10.1186/s12861-018-0174-4.
-
15.
Jiang YP, Ye RJ, Yang JM, Liu N, Zhang WJ, Ma L, et al. Protective effects of Salidroside on spermatogenesis in streptozotocin induced type-1 diabetic male mice by inhibiting oxidative stress mediated blood-testis barrier damage. Chem Biol Interact. 2020;315:108869. [PubMed ID: 31682803]. https://doi.org/10.1016/j.cbi.2019.108869.
-
16.
Yashwant Kumar A, Nandakumar K, Handral M, Talwar S, Dhayabaran D. Hypoglycaemic and anti-diabetic activity of stem bark extracts Erythrina indica in normal and alloxan-induced diabetic rats. Saudi Pharm J. 2011;19(1):35-42. [PubMed ID: 23960740]. [PubMed Central ID: PMC3745190]. https://doi.org/10.1016/j.jsps.2010.10.001.
-
17.
Oghbaei H, Alipour MR, Hamidian G, Ahmadi M, Ghorbanzadeh V, Keyhanmanesh R. Two months sodium nitrate supplementation alleviates testicular injury in streptozotocin-induced diabetic male rats. Exp Physiol. 2018;103(12):1603-17. [PubMed ID: 30088840]. https://doi.org/10.1113/EP087198.
-
18.
Johnsen SG. Testicular biopsy score count--a method for registration of spermatogenesis in human testes: normal values and results in 335 hypogonadal males. Hormones. 1970;1(1):2-25. [PubMed ID: 5527187]. https://doi.org/10.1159/000178170.
-
19.
Ellenburg JL, Kolettis P, Drwiega JC, Posey AM, Goldberg M, Mehrad M, et al. Formalin Versus Bouin Solution for Testis Biopsies: Which Is the Better Fixative? Clin Pathol. 2020;13:2632010X19897262. [PubMed ID: 31922127]. [PubMed Central ID: PMC6940599]. https://doi.org/10.1177/2632010X19897262.
-
20.
La Vignera S, Condorelli R, Vicari E, D'Agata R, Calogero AE. Diabetes mellitus and sperm parameters. J Androl. 2012;33(2):145-53. [PubMed ID: 21474785]. https://doi.org/10.2164/jandrol.111.013193.
-
21.
Han J, Qu Q, Qiao J, Zhang J. Vincamine Alleviates Amyloid-beta 25-35 Peptides-induced Cytotoxicity in PC12 Cells. Pharmacogn Mag. 2017;13(49):123-8. [PubMed ID: 28216895]. [PubMed Central ID: PMC5307895]. https://doi.org/10.4103/0973-1296.196309.
-
22.
Wahab OA, Princely AC, Oluwadamilare AA, Ore-Oluwapo DO, Blessing AO, Alfred EF. Clomiphene citrate ameliorated lead acetate-induced reproductive toxicity in male Wistar rats. JBRA Assist Reprod. 2019;23(4):336-43. [PubMed ID: 31173495]. [PubMed Central ID: PMC6798595]. https://doi.org/10.5935/1518-0557.20190038.
-
23.
Chenniappan K, Murugan K. Therapeutic and fertility restoration effects of Ionidium suffruticosum on sub-fertile male albino Wistar rats: effects on testis and caudal spermatozoa. Pharm Biol. 2017;55(1):946-57. [PubMed ID: 28183234]. [PubMed Central ID: PMC6130764]. https://doi.org/10.1080/13880209.2016.1278453.
-
24.
Ashidi JS, Owagboriaye FO, Yaya FB, Payne DE, Lawal OI, Owa SO. Assessment of reproductive function in male albino rat fed dietary meal supplemented with Mucuna pruriens seed powder. Heliyon. 2019;5(10). e02716. [PubMed ID: 31720466]. [PubMed Central ID: PMC6838900]. https://doi.org/10.1016/j.heliyon.2019.e02716.
-
25.
Haghmorad D, Mahmoudi MB, Haghighi P, Alidadiani P, Shahvazian E, Tavasolian P, et al. Improvement of fertility parameters with Tribulus Terrestris and Anacyclus Pyrethrum treatment in male rats. Int Braz J Urol. 2019;45(5):1043-54. [PubMed ID: 31626524]. [PubMed Central ID: PMC6844349]. https://doi.org/10.1590/S1677-5538.IBJU.2018.0843.
-
26.
Tian Y, Song W, Xu D, Chen X, Li X, Zhao Y. Autophagy Induced by ROS Aggravates Testis Oxidative Damage in Diabetes via Breaking the Feedforward Loop Linking p62 and Nrf2. Oxid Med Cell Longev. 2020;2020:7156579. [PubMed ID: 32509151]. [PubMed Central ID: PMC7254092]. https://doi.org/10.1155/2020/7156579.
-
27.
Huang W, Cao Z, Zhang J, Ji Q, Li Y. Aflatoxin B(1) promotes autophagy associated with oxidative stress-related PI3K/AKT/mTOR signaling pathway in mice testis. Environ Pollut. 2019;255(Pt 2):113317. [PubMed ID: 31610502]. https://doi.org/10.1016/j.envpol.2019.113317.
-
28.
Shang Y, Wang H, Jia P, Zhao H, Liu C, Liu W, et al. Autophagy regulates spermatid differentiation via degradation of PDLIM1. Autophagy. 2016;12(9):1575-92. [PubMed ID: 27310465]. [PubMed Central ID: PMC5082779]. https://doi.org/10.1080/15548627.2016.1192750.
-
29.
Nasiri K, Akbari A, Nimrouzi M, Ruyvaran M, Mohamadian A. Safflower seed oil improves steroidogenesis and spermatogenesis in rats with type II diabetes mellitus by modulating the genes expression involved in steroidogenesis, inflammation and oxidative stress. J Ethnopharmacol. 2021;275:114139. [PubMed ID: 33894286]. https://doi.org/10.1016/j.jep.2021.114139.