Abstract
Background:
Alzheimer's disease (AD) is a progressive neurodegenerative disease leading to neuronal cell death and manifested by cognitive disorders and behavioral impairment. Mesenchymal stem cells (MSCs) are one of the most promising candidates to stimulate neuroregeneration and prevent disease progression. Optimization of MSC culturing protocols is a key strategy to increase the therapeutic potential of the secretome.Objectives:
Here, we investigated the effect of brain homogenate of a rat model of AD (BH-AD) on the enhancement of protein secretion in the secretome of periodontal ligament stem cells (PDLSCs) when cultured in a 3D environment. Moreover, the effect of this modified secretome was examined on neural cells to study the impact of the conditioned medium (CM) on stimulation of regeneration or immunomodulation in AD.Methods:
PDLSCs were isolated and characterized. Then, the spheroids of PDLSCs were generated in a modified 3D culture plate. PDLSCs-derived CM was prepared in the presence of BH-AD (PDLSCs-HCM) and the absence of it (PDLSCs-CM). The viability of C6 glioma cells was assessed after exposure to different concentrations of both CMs. Then, a proteomic analysis was performed on the CMs.Results:
Differentiation into adipocytes and high expression of MSCs markers verified the precise isolation of PDLSCs. The PDLSC spheroids were formed after 7 days of 3D culturing, and their viability was confirmed. The effect of CMs on C6 glioma cell viability showed that both CMs at low concentrations (> 20 mg/mL) had no cytotoxic effect on C6 neural cells. The results showed that PDLSCs-HCM contains higher concentrations of proteins compared to PDLSCs-CM, including Src-homology 2 domain (SH2)-containing PTPs (SHP-1) and muscle glycogen phosphorylase (PYGM) proteins. SHP-1 has a role in nerve regeneration, and PYGM is involved in glycogen metabolism.Conclusions:
The modified secretome derived from 3D cultured spheroids of PDLSCs treated by BH-AD as a reservoir of regenerating neural factors can serve as a potential source for AD treatment.Keywords
Alzheimer's Disease Periodontal Ligament Stem Cells Spheroid Secretome Nerve Regeneration
1. Background
Alzheimer's disease (AD) is a neurodegenerative disorder that causes dementia and deteriorates cognitive function. AD pathology is identified by the aggregation of β amyloid peptides around neural cells in the extracellular matrix, as well as intracellular neurofibrils cumulation due to hyperphosphorylation of tau protein in neurons. Moreover, the inflammatory response and oxidative stress are other hallmarks of AD that lead to synaptic loss and neuronal death in different brain areas, including the hippocampus and cerebral cortex (1-3). The World Alzheimer Report (2018) indicates that around 50 million people are living with dementia worldwide. AD has quickly become an epidemic, and the number of patients is expected to reach 152 million in 2050 (3). Currently, there is no efficacious therapy capable of improving AD pathology. The US Food and Drug Administration approved 3 categories of treatments for AD, including N-methyl-D-aspartate receptor antagonists, cholinesterase inhibitors, and combined drug therapy (memantine with donepezil) (3). Although these medications can relatively alleviate dementia symptoms in AD patients, they have no effect on AD pathogenesis and cannot prevent AD from progressing or prolong the patient’s survival (4). Therefore, developing new and innovative therapeutic approaches is essential.
Recent studies have demonstrated that mesenchymal stem cells (MSCs) show the ability to promote neuroregeneration and prevent AD progression (5). Different types of MSCs and their derivatives have been transplanted into the brain of a mouse model of AD and shown various effects (6). Among various MSCs, dental tissue-derived MSCs [such as dental pulp stem cells, periodontal ligament stem cells (PDLSCs), and gingival stem cells originating from neural crest] are more attractive. Removal procedures of these cells are minimally invasive with no ethical issues and excellent potential for neurogenic differentiation (7). Studies have shown that dental MSCs have immunomodulatory and regenerative impacts using in vivo models of various diseases, such as neurotrauma and multiple sclerosis (7). These stem cells secret a cocktail of bioactive neurotrophic and immunomodulatory factors in their culture medium, providing therapeutic potential for a variety of disorders (including neurodegenerative diseases such as AD) (5, 6).
Human PDLSCs (hPDLSCs) are an accessible cell source of undifferentiated cells with a doubling rate of 22 hours and low immunogenicity (8). The cells comprise 95% of MSCs and 5% of neural crest stem cells (9). The cells have the ability to express markers of MSCs, embryonic stem cells, and neural crest cells. PDLCs have the potential to differentiate into multiple cell fates, including neurogenic lineages (10-12). PDLSCs can exert an immunomodulatory effect via inflammatory cell recruitment (13, 14). PDLSCs have shown the regeneration potential of damaged neural tissues in AD induced by the deposition of phosphorylated tau protein. It was demonstrated that co-culturing of PDLSCs with okadaic acid-induced human neuroblastoma SH-SY5Y cell line (as in vitro models of AD) led to the cytoskeleton and cell shape recovery, as well as good cell viability reduced apoptosis levels (15).
In this study, we established a 3D spheroid culture of PDLSCs to optimize the MSC culture to increase their therapeutic potential (16-18). Treatment with MSC spheroids has been previously investigated, for instance, in bone regeneration with moderate success (19, 20), animal model of damaged endometrium with improved fertility (21), and inflammatory and ischemic models such as colitis and stroke (22, 23).
There are many studies in the field of cell grafts. However, low differentiation and survival have been shown in a mouse model of AD; therefore, it can be concluded that MSC-mediated recovery from AD may be regulated by paracrine mechanisms (6).
Broad spectra of secreted neurotrophic factors [including Brain-Derived Neurotrophic Factor (BDNF), Vascular Endothelial Growth Factor A (VEGFA), Neurotrophin-3 (NT-3), Nerve growth factor (NGF), and Glial cell line-derived neurotrophic factor (GDNF)] were discovered in hPDLSCs conditioned medium (hPDLSCs-CM). PDLSCs have a promising potential for paracrine-mediated therapy of neural damage (24). PDLSCs-CM is useful in promoting long-term neuroregeneration in spinal cord injury (25). Moreover, it can reduce the inflammatory responses in animal models of MS (26).
In pathological conditions, upon receiving signals from damaged sites, various components of extracellular membrane vesicles, such as nucleic acids, proteins, soluble cytokines, and lipids, are secreted to inhibit pro-inflammatory responses, suppress apoptosis, and stimulate the differentiation of tissue-specific precursor cells through paracrine signaling (7). It has been demonstrated that when MSCs are exposed to an injured environment, immense bioactive components are released in their secretome. The neuroregenerative effects of implanted MSCs are fully replicated by the secretome (27).
Here, we investigated the stimulative effect of the preconditioning of PDLSC spheroids with brain homogenate of a rat model of AD (BH-AD) to increase the therapeutic benefits of PDLSCs-CM in neuroregeneration and neuroprotection in AD. These findings showed immense translational potential due to providing evidence in favor of using paracrine-mediated therapy for neurodegenerative diseases. This study examined whether crosstalk between PDLSCs and BH-AD would have additive effects in releasing more efficient neuroreparative secretomes. This study might be able to introduce an innovative hypothesis to promote the efficiency of the paracrine mechanism for the treatment of AD. Furthermore, the use of PDLSCs can be a new goal in clinical research of neurodegenerative diseases and their CM (cell-free) therapy for AD instead of direct implantation of the cells with their various challenges related to their clinical application.
2. Methods
2.1. Study Design and Ethics Approval
This research was carried out in accordance with the World Medical Association’s Code of Ethics (Declaration of Helsinki). Informed consent was obtained from all participants prior to sample collection. The Ethics Committee of Shahid Beheshti University of Medical Sciences approved this study (code: IR.SBMU.RETECH.REC.1400.601).
2.2. Isolation and Characterization of PDLSCs
Periodontal ligament biopsies were obtained from premolar teeth of young cases by scaling the roots. Teeth were healthy with no sign of periodontal disease, periapical lesion, caries, or gingivitis and were extracted with the aim of orthodontic therapies. PDLSCs were isolated by the explant culture of tooth samples. Periodontal tissues were minced and then incubated in a humid atmosphere (5% CO2) with a culture medium containing 10% fetal bovine serum (Biosera) and 1% of penicillin/streptomycin (Biosera) at 37°C. Cells were used in their third passage for all experiments. The expression of cell surface markers of PDLSCs was analyzed by flow cytometry. Cells (1 × 106) were incubated for 45 minutes at 4°C with specific antibodies conjugated with fluorescein isothiocyanate. Cells were stained using the following antibodies: anti-CD105, anti-CD90, anti-CD73, anti-CD44, anti-CD45, and anti-CD34. After incubation with each antibody, the cells were washed adequately and characterized with a flow cytometer (BD FACSCalibur Systems).
2.3. Evaluation of Differentiation Potential of PDLSCs
PDLSCs were differentiated in adipogenic and osteogenic differentiation mediums. For adipogenic differentiation, PDLSCs were cultured with low-glucose Dulbecco's Modified Eagle Medium (DMEM) supplemented with Fetal Bovine Serum (FBS) (10%), penicillin-streptomycin (1%), 3-isobutyl-1-methylxanthine (60 μM), insulin (10 μL/mL), indomethacin (0.5 mM), and dexamethasone (1 μM). Adipogenic and osteogenic induction was observed using a light/fluorescence microscope (a Nikon Eclipse Ti microscope equipped using a Nikon DS-Qi1Mc camera and NIS-Element’s software) (28).
2.4. 3D Cultured Spheroid Formation
PDLSCs were harvested at the third passage using 0.5% trypsin-EDTA, and 2 × 106 cells were sub-cultured in a complete cell culture medium in 8% agar-coated flasks (25 cm2) to prevent cell attachment to the surface of the flask and induce spheroid formation. Spheroids were fully formed after 7 days. Images of spheroids were obtained using an inverted microscope (Nikon Eclipse Ti microscope).
2.5. Live/Dead Staining
Cell viability of spheroids was investigated after 7 days via Live/Dead staining with acridine orange (AO, 5 mg/mL) and propidium iodide (PI, 3 mg/mL) using AO/PI staining solution (Nexcelom, CS2-0106). The PDLSC spheroids were centrifuged and resuspended in phosphate-buffered saline (PBS) containing 1 μL of PI and 2 μL of OA. The sample was incubated for 40 minutes in a humidified incubator (5% CO2) at 37°C. Then, the spheroids were washed with PBS to remove unbound dyes and imaged with a fluorescence microscope. The live cells were stained in green, and the dead cells were stained in red (29).
2.6. Preparation of BH-AD
To prepare brain homogenate, 8 male Sprague-Dawley rats (220-250g) were provided from the Animal House of Shahid Beheshti University of Medical Sciences, Tehran, Iran. The animals were kept in clean cages (5 rats per cage) with a 12-hour light-dark cycle at 25 ± 2°C and 50% ± 10% humidity. Animals had free access to tap water and standard food.
2.6.1. Stereotaxic Surgery and Injection of Streptozotocin
The rats were anesthetized by administration of ketamine/xylazine (100/10 mg/kg intraperitoneally) and subjected to stereotaxic surgery. A single-dose streptozotocin (STZ, 3 mg/kg) was injected bilaterally into the intracerebroventricular region of the brain of the rats to develop a model of AD (30, 31). After surgery, the animals were put in cages with free access to water and food. Two groups of rats were used in this study: STZ-induced rats and sham rats (subjected to stereotaxic surgery but without administration of STZ). There were 8 rats in each group.
2.6.2. Radial Arm Water Maze Test
After 21 days, spatial learning and memory in rats were investigated through a radial arm water maze (RAWM) test. The equipment was made of 6 arms and kept in a water tank with a 50 cm depth at 25°C. A behavioral test was performed in an air-regulated and sound-proof experimental room. Each rat was tested for 3 days. The first 2 days were for the training trials (10 trials per day), and the third day was for the probe trial. During each trial, the target platform was placed at the end of one of the 6 arms, and the rat was placed in another arm (starting arm). Visual cues were attached at the end of each arm, and each rat had a specific target platform with a randomized starting arm. Each rat had 60 seconds to transfer to the target arm. The latency to reach the platform and the number of entries to the non-target arms were assayed. After 2 days of training, the platform was “invisible” in all target arms. To begin the probe trial on the third day, each rat was located in the starting arm; then, the transfer latency to find the target arm, the number of entries to the goal arm, and the spent time in the goal arm were recorded. All trials were recorded by digital camera and analyzed via EthoVision tracking software (Noldus, the Netherlands) (32-34).
2.6.3. Collection of BH-AD
After behavioral tests, the brains of the rat models of AD were harvested and homogenized in DMEM F12 (100 mg/mL; Biosera) on ice. Then, the BH-AD was centrifuged at 4°C (12.000 rcf, 20 minutes), and the supernatant was collected. The supernatant was frozen in liquid nitrogen and kept at -80°C until use.
2.7. Preparation of PDLSCs-CM
Two different batches were used to collect CM, including CM derived from PDLSC spheroids cultured in general medium (PDLSCs-CM) and CM derived from PDLSC spheroids cultured in the presence of BH-AD (PDLSCs-HCM). Spheroids were cultured in a low FBS medium containing 0.5% FBS for 48 hours to secret PDLSCs-CM. Then, CM was collected, centrifuged for 3 minutes at 3000 g and 5 minutes at 1500 g, and stored at -80°C for 24 hours (Figure 1).
Schematic illustration of the process of the periodontal ligament stem cell conditioned medium collection
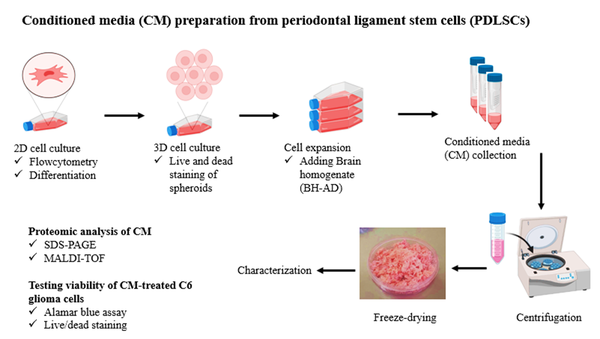
To collect PDLSCs-HCM, the spheroids were first cultured in a starvation medium for 30 minutes. Then, they were induced by a complete cell culture medium containing 10% BH-AD for 24 hours. Next, the culture medium was removed, and the preconditioned PDLSC spheroids were cultured in a low FBS medium containing 0.5% FBS for 48 hours. PDLSCs-HCM was collected and preserved at -80°C in a freezer. The frozen samples of PDLSCs- CM and HCM were freeze-dried for 24 hours and then stored at -20°C until use.
2.8. Cell Viability Analysis in the Presence of PDLSCs-CMs
To evaluate biocompatibility and cell viability in the presence of CMs, C6 glioma cells were cultured at a density of 8000 cells per well in 96-well plates. After 24 hours, culture media was removed, and different concentrations of both CMs were added to each well separately.
The cell viability was evaluated using the Alamar Blue (AB) assay. After 24, 48, and 72 hours of culturing, culture media containing CM was removed, and a medium with 10% AB dye (Sigma-Aldrich) was added to each well. The cells were incubated at 37°C for 24 hours, and the absorbance was measured using a microplate reader at 570 and 630 nm.
The cytotoxic effects of the CMs were also evaluated using Live/Dead assays with AO/PI cell viability kits. A Live/Dead assay was performed after 72 hours of incubation of C6 glioma cells with both CMs.
2.9. Detection of Secretome Proteins by 1D SDS-Polyacrylamide Gel Electrophoresis and Matrix Assisted Laser Desorption/Ionization- Time of Flight Mass Spectrometry (MALDI-TOF-MS)
Equal weights of lyophilized CMs were dissolved in equal amounts of DMEM F12. Proteins of 2 different samples were separated by 1D SDS-polyacrylamide gel electrophoresis (1D SDS-PAGE). Polyacrylamide gels were cast between 8 × 10 cm glass plates with a thickness of 1 mm. The polyacrylamide gel solution was poured between glass plates up to 2 cm from the upside. After polymerization, a 10-well comb was placed on the upside of the gel, and a stacking acrylamide gel solution (4% T) was added. To prepare samples, a boiling buffer containing 100-g/L SDS and 8-mol/L urea was added to the test samples in a 1: 1 volume ratio for 4 minutes and boiled for 5 minutes. Samples were centrifuged and loaded into the wells. Electrophoresis was performed at 30 mA/gel, 180 V, for 1.5 hours by a Tris/Glycine/SDS buffer at a pH of 8.3. Then, gels were stained with 2 dyes: Coomassie brilliant blue and silver nitrate (35). Different spots were cut from gels and digested for Matrix Assisted Laser Desorption/Ionization- Time of Flight (MALDI-TOF) analysis.
The MALDI-TOF technique was used to detect the protein content of HCM compared to CM, and matrix-assisted mass spectrometry (Applied Biosystems MALDI-TOF) was used. The digested samples from SDS-PAGE were spotted on a MALDI plate with alpha-cyano-4-hydroxy-cinnamic acid (CHCA) matrix solution in 50% acetonitrile (ACN) containing 0.1% trifluoroacetic acid (TFA) with a 1: 2 ratio. Then, they were air-dried and analyzed in the mass range of 800 - 4000 D. The interpretation of data was performed using Data Explorer version 4.0. The functional role of the detected proteins was evaluated through KEGG (Kyoto Encyclopedia of Genes and Genomes) pathway analysis, and gene ontology of biological processes was performed using the GeneCards database.
2.10. Statistical Analysis
We used GraphPad Prism 8 software, and data were statistically analyzed with a 1-way analysis of variance (ANOVA), followed by a Tukey post-test. Statistical significance was considered at *P < 0.05, **P < 0.01, ***P < 0.001, and ****P < 0.0001.
3. Results
3.1. PDLSCs Characterization
All isolated PDLSCs were adhered to the surface of the culture flask and illustrated a spindle-like morphology (Figure 2A). To investigate the differentiation potential, PDLSCs were incubated in the induction culture media for adipogenesis. The formation of cytoplasmic lipid vacuoles in red confirmed adipogenesis of isolated cells. Our results demonstrated that PDLSCs had the ability to differentiate into adipogenic lineage (Figure 2B). Flow cytometry analyses were performed in the third passage of the cells to determine the cell surface markers of PDLSCs. The results showed that hematopoietic-specific antigens, such as CD45 (98.9%) and CD34 (99%), were negative for the cells, and the expression of markers specific for MSCs, including CD44 (98.7%), CD90 (99.1%), CD105 (98.8%), and CD73 (99.5%), were positive for PDLSCs (Figure 3).
Periodontal ligament stem cells and their characterization. A, In the third passage (20×); B, Adipogenic differentiation. The lipid vacuoles were stained using oil red (20×).
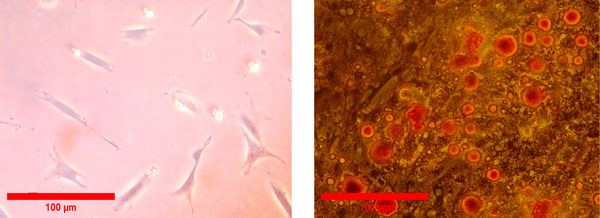
Flow cytometry of stem cells from human periodontal ligament
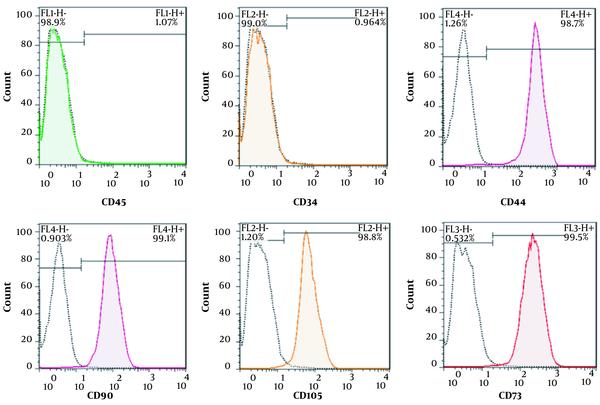
3.2. Morphology and Viability of Spheroids
The culture of PDLSCs in the agar-coated flasks led to the attachment of cells, and the spheroid formation initiated after about 24 hours. Spheroids with a diameter ranging between 250 and 300 μm were fully formed after 7 days. Live cells were identified by a strong green fluorescence signal, and a red fluorescence signal indicated the dead cells. The results confirmed the well-preserved viable cells on the seventh day of spheroid formation (Figure 4).
Spheroid formation after culturing periodontal ligament stem cells on an agar-coated flask and Live/Dead assay for the viability of cells. A, After 24 hours; B, After 48 hours; C, After 7 days with light microscopy (10×). Live/Dead staining of spheroids; D and E, After 5 days (20× and 10×, respectively); F, After culturing in 0.5% FBS medium with fluorescent microscopy (10×).
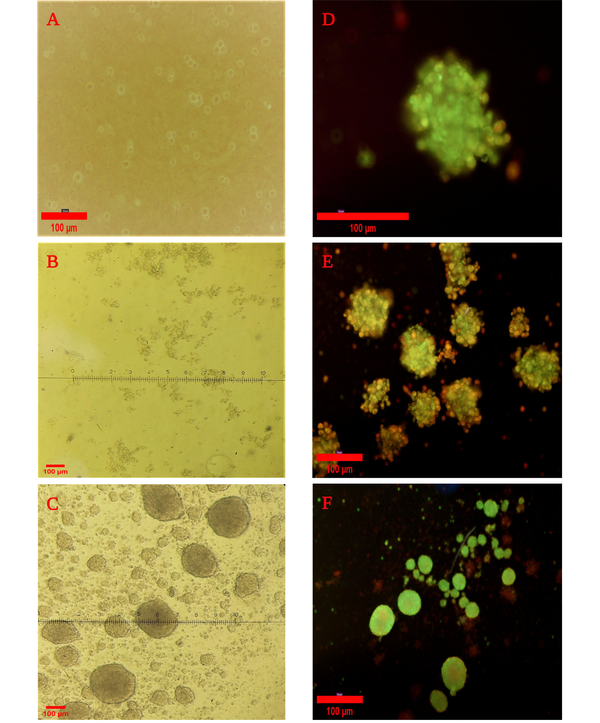
3.3. Modeling AD in Rats
We used STZ-induced dementia in rats as a model of AD. After 21 days, a RAWM test was carried out to examine the spatial memory of rats after receiving STZ. Then, the modeled rats were compared to the control rats to verify the effect of STZ. The results of the RAWM test are shown in Figure 5. In STZ-treated rats, the latency to locate the target arm was higher. The duration spent in the target arm and the number of entries to the target arm were less than the sham group. After establishing the models of AD, the brains of the rats were harvested and homogenized to use in PDLSCs-HCM preparation.
The effect of streptozotocin on learning and memory of streptozotocin-induced rats; A, The effects of streptozotocin on duration in the target arm in the probe trial in the radial arm water maze; B, The effects of streptozotocin on the number of entries to the target arm; C, The effects of streptozotocin on the latency to locate the target arm. Data are described as mean ± SEM (n = 8).
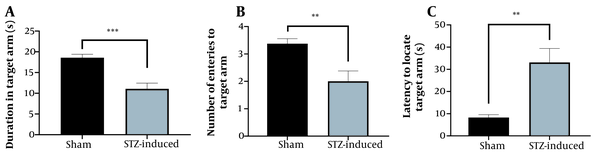
3.4. Cell Viability in the Presence of PDLSCs-CMs
PDLSCs-CM and PDLSCs-HCM were prepared, and the viability of the C6 cells after treatment with different concentrations of the CMs was examined by the AB assay and Live/Dead staining after 24, 48, and 72 hours. The results of light microscopy are presented in Figure 6. The graphs of the cell viability assay at different concentrations of CMs (Figure 6) showed that at 24 hours, the cell viability of the treated cells decreased at low and high concentrations of CMs. In lower concentrations, no significant difference was observed between the samples of PDLSCs-HCM-treated cells and negative controls after 48 and 72 hours of incubation (P < 0.05). The results of the Live/Dead assay showed that a significant increase in dead cells was observed in the cells exposed to very low and high concentrations of CMs after 72 hours (Figure 7).
Cell viability assay; A, Light microscopy of C6 cells treated with different concentrations of CMs [(a) control, (b) 5 mg/mL, (c) 20 mg/mL, and (d) 40 mg/mL]; B, Cell viability graphs at different time points at 24, 48, and 72 hours and various concentrations of periodontal ligament stem cell conditioned medium and Periodontal ligament stem cells-homogenate exposed cells derived conditioned media (PDLSCs-HCM).
![Cell viability assay; A, Light microscopy of C6 cells treated with different concentrations of CMs [(a) control, (b) 5 mg/mL, (c) 20 mg/mL, and (d) 40 mg/mL]; B, Cell viability graphs at different time points at 24, 48, and 72 hours and various concentrations of periodontal ligament stem cell conditioned medium and Periodontal ligament stem cells-homogenate exposed cells derived conditioned media (PDLSCs-HCM). Cell viability assay; A, Light microscopy of C6 cells treated with different concentrations of CMs [(a) control, (b) 5 mg/mL, (c) 20 mg/mL, and (d) 40 mg/mL]; B, Cell viability graphs at different time points at 24, 48, and 72 hours and various concentrations of periodontal ligament stem cell conditioned medium and Periodontal ligament stem cells-homogenate exposed cells derived conditioned media (PDLSCs-HCM).](https://services.brieflands.com/cdn/serve/3163e/8f3f5b828807cb173ac3641920bdc4732fc9f353/ijpr-21-1-133668-i003-preview.png)
Live/Dead staining of C6 glioma cells after 72 hours of treatment with different concentrations of periodontal ligament stem cell conditioned medium and Periodontal ligament stem cells-homogenate exposed cells derived conditioned media (PDLSCs-HCM). A, Live cells are green and dead cells are red (10× and 20×); B, percent of live and dead cells.
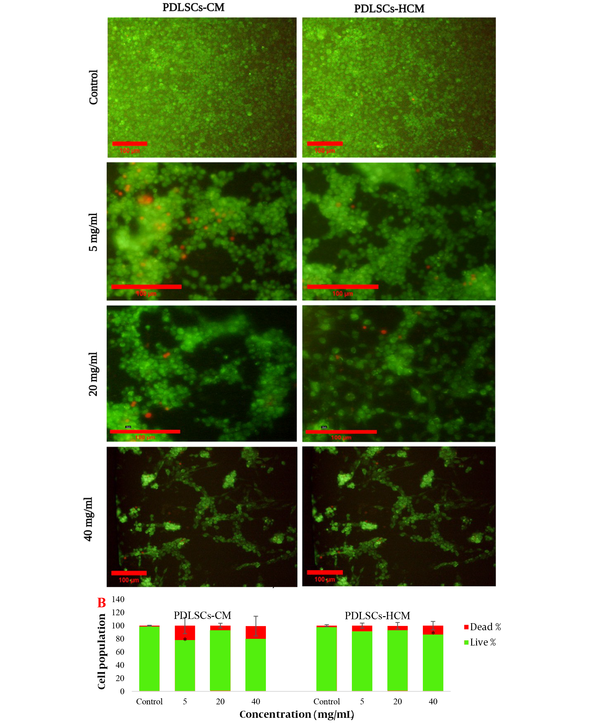
3.5. Proteomic Analysis
The proteins of both CMs were separated using the SDS-PAGE technique (Figure 8). The patterns of protein expression were compared between the 2 samples. The bands on the gel were cut and analyzed using MALDI-TOF-MS (Figure 9). BH-AD-treated cells showed more protein secretion in their CM compared to the non-treated cells. After analysis with MALDI-TOF/MS technique, 3 proteins, including protein tyrosine phosphatase non-receptor type 6 (PTPN6) or SHP-1, muscle glycogen phosphorylase (PYGM), and putative protein FAM90A20P, were particularly detected in PDLSCs-HCM.
Staining of SDS-polyacrylamide gel electrophoresis with 2 dyes: Coomassie blue and silver nitrate
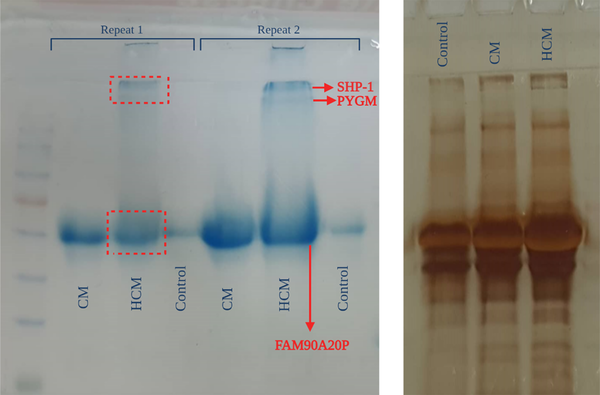
Spectrums of the matrix assisted laser desorption/ionization- time of flight mass spectrometry (MALDI-TOF-MS) for different bands on the gel
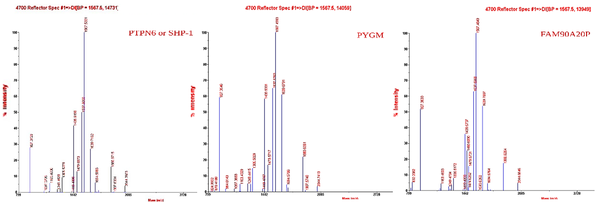
4. Discussion
MSCs can express neuroprotective factors, such as cytokines, chemokines, growth factors, adhesion molecules, angiogenic molecules, hormones, and enzymes. Most of these proteins and metabolites greatly contribute to immunomodulation and regenerative therapy (25). It has been demonstrated that the 3D spheroid culturing of MSCs can better simulate the body environment in vitro; therefore, MSCs secret different protein content in their secretome when cultured in 3D culture compared to 2D culture. The released secretome from 3D cultured spheroids can lead to further angiogenesis and wound healing (16, 36, 37), a higher anti-inflammatory effect (17, 38, 39), and richer expression of regenerative trophic factors, including stem cell factor (SCF), fibroblast growth factor 2 (FGF-2), the human cytokine I-309, platelet derived growth factor (PDGF-BB), and granulocyte-macrophage colony-stimulating factor (GM-CSF) (39).
Moreover, the manipulation of the culturing condition of MSCs is a beneficial strategy to improve the therapeutic potential of MSCs through the paracrine mechanism. Furthermore, it has been demonstrated that MSCs can release richer secretomes when exposed to an injured environment caused by a disease (27).
In the current study, the PDLSCs were cultured in a 3D environment. Moreover, the effect of brain homogenate taken from STZ-induced AD-like disease in rats was studied on promoting the protein content of the PDLSC secretome in a 3D cultured spheroid form. The spheroids were exposed to the brain homogenate, and the proteins of their CM were evaluated. This strategy was proposed to improve the efficacy of the PDLSCs-derived secretome for the treatment of AD.
In this study, PDLSCs were isolated and characterized by adipogenic differentiation and flow cytometry. The adipogenic differentiation potential of the isolated cells was verified. Also, the results of flow cytometry showed stemness of the cells with negative hematopoietic-specific antigens and positive markers specific for MSCs. We clearly demonstrated that the 3D cultured spheroids of isolated PDLSCs were fully formed after 7 days of culturing in a non-adherent environment, and the viability of the cells in 3D cultured spheroids was confirmed at different time points of the spheroid formation. Our results contrast with the results reported by Jeong et al. showing that the viability of PDLSCs in 3D culturing decreased compared to traditional 2D culturing (40). Besides, based on the culturing method, the spheroid morphology was preserved for 3 - 21 days, and the internal structure was homogeneous and biologically stable for at least 30 days (41, 42).
In this study, we cultured PDLSC spheroids in 2 different conditions, including in a normal culture medium and the presence of BH-AD. BH-AD was collected from AD models with verified AD-like behavior using a RAWM test. It was demonstrated that the duration in the target arm was less in the control group (STZ-induced rats) than in the sham group. Moreover, the treated rats entered the target arm less often and had latency to locate at the target arm. The results showed a significant difference in learning and memory between the control (STZ-treated rats) and sham groups. It was confirmed that an AD model was established; therefore, we used their brain homogenate to prepare HCM.
We collected CMs in both culturing conditions and investigated the viability of the C6 glioma cells after exposure to different concentrations of both collected CMs through Live/Dead and AB assays. The results of these assays after 24, 48, and 72 hours of incubation showed that the cellular viability was significantly maintained in the presence of low concentrations of CMs (> 20 mg/mL). The results revealed that cell viability in both groups was not dependent on time and concentration, except in the 40-mg/mL concentrations that significantly decreased compared to the control group at all time points (Figure 6). Also, in the presence of 20-mg/mL PDLSCs-CM, the cell viability significantly decreased after 72 hours of incubation compared to the control group. After 48 and 72 hours, the treated cells were adapted to CMs; however, they showed various reactions to different concentrations of CMs.
Our findings are in agreement with the findings of Bari et al, showing that the MSCs-derived secretome had no cytotoxic effect on human chondrocytes and fibroblasts at concentrations lower than 25 mg/mL (43). The Live/Dead assay showed a significant difference in the number of viable cells during the 72-hour culture at very low and high concentrations of CMs.
In this study, a proteomic analysis was performed to determine the protein profiles of the PDLSCs-derived secretome in 2 culturing conditions in the presence and absence of BH-AD. The results confirmed that when PDLSCs were exposed to culture media supplemented with different components (especially proteins from disease-affected environments), protein expression profile of the exposed cells was altered (Figure 8). PDLSCs treated with BH-AD produced more protein compared to non-treated cells. Three proteins were detected in PDLSCs-HCM, including PTPN6 or SHP-1, muscle PYGM, and putative protein FAM90A20P.
PTPN6 or SHP-1 is a multifunctional signaling protein involved in the development of the central nervous system (CNS). The cortex, cerebellum, and cervical spinal cord have high expression of this protein. SHP-1 is also involved in astrocyte proliferation and differentiation, as well as oligodendrocyte maturation, differentiation, and survival.
SHP-1 deficiency causes a decrease in astrocytes and microglia cell population in CNS and impaired myelination as well (44). This protein was shown to be overexpressed in the CM of PDLSC spheroids treated with BH-AD (HCM). SHP-1 has been identified to be associated with synaptic vesicles and interact with synaptophysin, implying that it plays a role in neurotransmission. It is also involved in neuronal signaling cascades of coupled Ig-like receptor-B with SHP1 that promotes axon regeneration (44, 45).
It has been shown that hippocalcin (a calcium-binding protein involved in neural activity) induces neuronal differentiation by activating the protein kinase C (PKC)/phospholipase D1 (PLD1) cascade, subsequently leading to SHP-1 activation. SHP-1 dephosphorylates signal transducer and activator of transcription 3 (STAT3,Y705) and prevents astrocytic differentiation (46). Neuroinflammation and increasing oxidative stress are the hallmarks of AD (47). SHP-1 has been shown to affect the function of inflammatory cells by inducing the survival of macrophages, a process that is independent of growth factors, such as colony-stimulating factor-1. Moreover, SHP-1 can decrease inflammation via the regulation of chemokine gene expression and the modulation of interferon regulatory factor-1. In addition, SHP-1 may be responsible for the support of photoreceptors and the protection against retinal degeneration by preventing the production of NO (48). PTPN6 or SHP-1 is involved in the differentiation of hematopoietic progenitor cells, positive regulation of cell proliferation, and negative regulation of humoral immune response mediated by circulating immunoglobulin. It is also involved in protein dephosphorylation and G protein-coupled receptor signaling pathway (Table 1).
Gene Ontology and KEGG Pathway Analysis of Detected Proteins
Protein Name | Gene Ontology-Biological Process | KEGG Pathway | Disease |
---|---|---|---|
PTPN6 or SHP-1 | Involved in hematopoietic progenitor cell differentiation, negative regulation of humoral immune response mediated by circulating immunoglobulin, protein dephosphorylation, G protein-coupled receptor signaling pathway, and positive regulation of cell population proliferation | Adherent junction, JAK-STAT signaling pathway, natural killer cell-mediated cytotoxicity, T cell receptor signaling pathway, B cell receptor signaling pathway, proteoglycans in cancer, PD-L1 expression, and PD-1 checkpoint pathway in cancer | Cd45 deficiency, polycythemia, polycythemia vera, subcorneal pustular dermatosis, myeloproliferative, and neoplasm |
PYGM | Involved in the carbohydrate metabolic process, glycogen metabolic process, glycogen catabolic process, and metabolic process | - | Glycogen storage disease V, glycogen storage disease Vii, muscular atrophy, and myoglobinuria |
We also detected PYGM in PDLSCs-HCM. Genes of both the liver and muscle isozymes of the PYGM enzyme are expressed in the human brain (49). PYGM has a major role in the carbohydrate/glycogen metabolic process and glycogen catabolic process. The rate-limiting stage of glycogen mobilization is catalyzed by this important enzyme in the glycogen metabolism process (50). In a study, a molecular analysis showed that the expression levels of glycogenesis genes, such as phosphoglucomutase 1, hexokinase, glucose transporter 1, glycogen synthase 1, and PYGM, were regulated by hypoxia-inducible factor-1, phosphoinositide 3-kinase/protein kinase B also known as AKT, and glycogen synthase kinase-3β; therefore, they regulated the glycogen metabolism of stem cells during hypoxic preconditioning (51).
As an important energy supply of neurons, lactate is required to integrate the glutamatergic neurotransmission and glucose consumption processes and is provided by glycogenolysis. In the brain, PYGM plays a key role in the process of glycogenolysis and the decomposition of stored glycogen. The alpha-1,4 glycosidic bond of glycogen was catalyzed by the phosphorolytic cleavage activity of this enzyme and led to releasing of glucose-1- phosphate. Pinacho et al. reported that in schizophrenia, the protein expression level of PYGM and its activity regulator protein [ras-related C3 botulinum toxin substrate 1 (RAC1)] both decreased in the astrocytes. In astrocytes, the interaction between PYGM and RAC1 may be similar to their interaction in T cells (52).
In schizophrenia, a disorder in a metabolic pathway in astrocytes that involves PYGM could lead to a transitory local energy shortage in the dorsolateral prefrontal cortex (53). According to another study, brain PYGM decreased in the lesioned hemisphere of the teleost fish model (54). A deficit of the glucose metabolic process was seen in a pathological state of AD (55); therefore, exogenous PYGM from HCM could sustain energy requirements.
The putative protein FAM90A20P is one of the proteins observed in PDLSCs-CM. It is a pseudogene, and its role should be investigated.
4.1. Conclusion
The findings of the current study support the excellent potential of CM derived from 3D-cultured PDLSCs treated with homogenate of the AD rat brain (BH-AD) as a modified secretome for neural regeneration. The CM was enriched with SHP-1 as an inducer of neural differentiation, which is involved in anti-inflammatory processes. In addition, PYGM (as a glycogenolysis enzyme) overexpressed in this modified secretome. Since it participates in the glucose metabolic process, it can be involved in neural regeneration due to supporting energy requirements in the AD brain.
Acknowledgements
References
-
1.
Wang Z, Peng W, Zhang C, Sheng C, Huang W, Wang Y, et al. Effects of stem cell transplantation on cognitive decline in animal models of Alzheimer's disease: A systematic review and meta-analysis. Sci Rep. 2015;5:12134. [PubMed ID: 26159750]. [PubMed Central ID: PMC4498325]. https://doi.org/10.1038/srep12134.
-
2.
Ahmed Nel M, Murakami M, Hirose Y, Nakashima M. Therapeutic Potential of Dental Pulp Stem Cell Secretome for Alzheimer's Disease Treatment: An In Vitro Study. Stem Cells Int. 2016;2016:8102478. [PubMed ID: 27403169]. [PubMed Central ID: PMC4923581]. https://doi.org/10.1155/2016/8102478.
-
3.
Liu XY, Yang LP, Zhao L. Stem cell therapy for Alzheimer's disease. World J Stem Cells. 2020;12(8):787-802. [PubMed ID: 32952859]. [PubMed Central ID: PMC7477654]. https://doi.org/10.4252/wjsc.v12.i8.787.
-
4.
Zhang XM, Ouyang YJ, Yu BQ, Li W, Yu MY, Li JY, et al. Therapeutic potential of dental pulp stem cell transplantation in a rat model of Alzheimer's disease. Neural Regen Res. 2021;16(5):893-8. [PubMed ID: 33229725]. [PubMed Central ID: PMC8178760]. https://doi.org/10.4103/1673-5374.297088.
-
5.
Hernández AE, García E. Mesenchymal Stem Cell Therapy for Alzheimer's Disease. Stem Cells Int. 2021;2021:7834421. [PubMed ID: 34512767]. [PubMed Central ID: PMC8426054]. https://doi.org/10.1155/2021/7834421.
-
6.
Mita T, Furukawa-Hibi Y, Takeuchi H, Hattori H, Yamada K, Hibi H, et al. Conditioned medium from the stem cells of human dental pulp improves cognitive function in a mouse model of Alzheimer's disease. Behav Brain Res. 2015;293:189-97. [PubMed ID: 26210934]. https://doi.org/10.1016/j.bbr.2015.07.043.
-
7.
Soundara Rajan T, Giacoppo S, Diomede F, Bramanti P, Trubiani O, Mazzon E. Human periodontal ligament stem cells secretome from multiple sclerosis patients suppresses NALP3 inflammasome activation in experimental autoimmune encephalomyelitis. Int J Immunopathol Pharmacol. 2017;30(3):238-52. [PubMed ID: 28764573]. [PubMed Central ID: PMC5815262]. https://doi.org/10.1177/0394632017722332.
-
8.
Fortino VR, Chen RS, Pelaez D, Cheung HS. Neurogenesis of neural crest-derived periodontal ligament stem cells by EGF and bFGF. J Cell Physiol. 2014;229(4):479-88. [PubMed ID: 24105823]. [PubMed Central ID: PMC4292882]. https://doi.org/10.1002/jcp.24468.
-
9.
Ng TK, Huang L, Cao D, Yip YW, Tsang WM, Yam GH, et al. Cigarette smoking hinders human periodontal ligament-derived stem cell proliferation, migration and differentiation potentials. Sci Rep. 2015;5:7828. [PubMed ID: 25591783]. [PubMed Central ID: PMC5379007]. https://doi.org/10.1038/srep07828.
-
10.
Coura GS, Garcez RC, de Aguiar CB, Alvarez-Silva M, Magini RS, Trentin AG. Human periodontal ligament: a niche of neural crest stem cells. J Periodontal Res. 2008;43(5):531-6. [PubMed ID: 18624954]. https://doi.org/10.1111/j.1600-0765.2007.01065.x.
-
11.
Huang CY, Pelaez D, Dominguez-Bendala J, Garcia-Godoy F, Cheung HS. Plasticity of stem cells derived from adult periodontal ligament. Regen Med. 2009;4(6):809-21. [PubMed ID: 19903001]. https://doi.org/10.2217/rme.09.55.
-
12.
Song JS, Kim SO, Kim SH, Choi HJ, Son HK, Jung HS, et al. In vitro and in vivo characteristics of stem cells derived from the periodontal ligament of human deciduous and permanent teeth. Tissue Eng Part A. 2012;18(19-20):2040-51. [PubMed ID: 22571499]. https://doi.org/10.1089/ten.TEA.2011.0318.
-
13.
Iwata T, Yamato M, Zhang Z, Mukobata S, Washio K, Ando T, et al. Validation of human periodontal ligament-derived cells as a reliable source for cytotherapeutic use. J Clin Periodontol. 2010;37(12):1088-99. [PubMed ID: 20618549]. https://doi.org/10.1111/j.1600-051X.2010.01597.x.
-
14.
Washio K, Iwata T, Mizutani M, Ando T, Yamato M, Okano T, et al. Assessment of cell sheets derived from human periodontal ligament cells: a pre-clinical study. Cell Tissue Res. 2010;341(3):397-404. [PubMed ID: 20632035]. https://doi.org/10.1007/s00441-010-1009-1.
-
15.
Wang D, Wang Y, Tian W, Pan J. Advances of tooth-derived stem cells in neural diseases treatments and nerve tissue regeneration. Cell Prolif. 2019;52(3). e12572. [PubMed ID: 30714230]. [PubMed Central ID: PMC6536383]. https://doi.org/10.1111/cpr.12572.
-
16.
Santos JM, Camões SP, Filipe E, Cipriano M, Barcia RN, Filipe M, et al. Three-dimensional spheroid cell culture of umbilical cord tissue-derived mesenchymal stromal cells leads to enhanced paracrine induction of wound healing. Stem Cell Res Ther. 2015;6(1):90. [PubMed ID: 25956381]. [PubMed Central ID: PMC4448539]. https://doi.org/10.1186/s13287-015-0082-5.
-
17.
Cesarz Z, Tamama K. Spheroid Culture of Mesenchymal Stem Cells. Stem Cells Int. 2016;2016:9176357. [PubMed ID: 26649054]. [PubMed Central ID: PMC4663368]. https://doi.org/10.1155/2016/9176357.
-
18.
Frith JE, Thomson B, Genever PG. Dynamic three-dimensional culture methods enhance mesenchymal stem cell properties and increase therapeutic potential. Tissue Eng Part C Methods. 2010;16(4):735-49. [PubMed ID: 19811095]. https://doi.org/10.1089/ten.TEC.2009.0432.
-
19.
Suenaga H, Furukawa KS, Suzuki Y, Takato T, Ushida T. Bone regeneration in calvarial defects in a rat model by implantation of human bone marrow-derived mesenchymal stromal cell spheroids. J Mater Sci Mater Med. 2015;26(11):254. [PubMed ID: 26449444]. [PubMed Central ID: PMC4598349]. https://doi.org/10.1007/s10856-015-5591-3.
-
20.
Yamaguchi Y, Ohno J, Sato A, Kido H, Fukushima T. Mesenchymal stem cell spheroids exhibit enhanced in-vitro and in-vivo osteoregenerative potential. BMC Biotechnol. 2014;14:105. [PubMed ID: 25479895]. [PubMed Central ID: PMC4299781]. https://doi.org/10.1186/s12896-014-0105-9.
-
21.
Domnina A, Novikova P, Obidina J, Fridlyanskaya I, Alekseenko L, Kozhukharova I, et al. Human mesenchymal stem cells in spheroids improve fertility in model animals with damaged endometrium. Stem Cell Res Ther. 2018;9(1):50. [PubMed ID: 29482664]. [PubMed Central ID: PMC5828181]. https://doi.org/10.1186/s13287-018-0801-9.
-
22.
Guo L, Ge J, Zhou Y, Wang S, Zhao RC, Wu Y. Three-dimensional spheroid-cultured mesenchymal stem cells devoid of embolism attenuate brain stroke injury after intra-arterial injection. Stem Cells Dev. 2014;23(9):978-89. [PubMed ID: 24341685]. [PubMed Central ID: PMC3997073]. https://doi.org/10.1089/scd.2013.0338.
-
23.
Molendijk I, Barnhoorn MC, de Jonge-Muller ES, Mieremet-Ooms MA, van der Reijden JJ, van der Helm D, et al. Intraluminal Injection of Mesenchymal Stromal Cells in Spheroids Attenuates Experimental Colitis. J Crohns Colitis. 2016;10(8):953-64. [PubMed ID: 26896084]. https://doi.org/10.1093/ecco-jcc/jjw047.
-
24.
Yamaza T, Kentaro A, Chen C, Liu Y, Shi Y, Gronthos S, et al. Immunomodulatory properties of stem cells from human exfoliated deciduous teeth. Stem Cell Res Ther. 2010;1(1):5. [PubMed ID: 20504286]. [PubMed Central ID: PMC2873699]. https://doi.org/10.1186/scrt5.
-
25.
Man RC, Sulaiman N, Idrus RBH, Ariffin SHZ, Wahab RMA, Yazid MD. Insights into the Effects of the Dental Stem Cell Secretome on Nerve Regeneration: Towards Cell-Free Treatment. Stem Cells Int. 2019;2019:4596150. [PubMed ID: 31772587]. [PubMed Central ID: PMC6855004]. https://doi.org/10.1155/2019/4596150.
-
26.
Rajan TS, Giacoppo S, Diomede F, Ballerini P, Paolantonio M, Marchisio M, et al. The secretome of periodontal ligament stem cells from MS patients protects against EAE. Sci Rep. 2016;6:38743. [PubMed ID: 27924938]. [PubMed Central ID: PMC5141419]. https://doi.org/10.1038/srep38743.
-
27.
Santamaria G, Brandi E, Vitola P, Grandi F, Ferrara G, Pischiutta F, et al. Intranasal delivery of mesenchymal stem cell secretome repairs the brain of Alzheimer's mice. Cell Death Differ. 2021;28(1):203-18. [PubMed ID: 32704089]. [PubMed Central ID: PMC7852675]. https://doi.org/10.1038/s41418-020-0592-2.
-
28.
Trubiani O, Piattelli A, Gatta V, Marchisio M, Diomede F, D'Aurora M, et al. Assessment of an efficient xeno-free culture system of human periodontal ligament stem cells. Tissue Eng Part C Methods. 2015;21(1):52-64. [PubMed ID: 24787358]. [PubMed Central ID: PMC4290797]. https://doi.org/10.1089/ten.TEC.2014.0024.
-
29.
Kummrow A, Frankowski M, Bock N, Werner C, Dziekan T, Neukammer J. Quantitative assessment of cell viability based on flow cytometry and microscopy. Cytometry A. 2013;83(2):197-204. [PubMed ID: 23081720]. https://doi.org/10.1002/cyto.a.22213.
-
30.
Kamat PK. Streptozotocin induced Alzheimer's disease like changes and the underlying neural degeneration and regeneration mechanism. Neural Regen Res. 2015;10(7):1050-2. [PubMed ID: 26330820]. [PubMed Central ID: PMC4541228]. https://doi.org/10.4103/1673-5374.160076.
-
31.
Sodhi RK, Singh N. All-trans retinoic acid rescues memory deficits and neuropathological changes in mouse model of streptozotocin-induced dementia of Alzheimer's type. Prog Neuropsychopharmacol Biol Psychiatry. 2013;40:38-46. [PubMed ID: 23044340]. https://doi.org/10.1016/j.pnpbp.2012.09.012.
-
32.
Khoramjouy M, Naderi N, Kobarfard F, Heidarli E, Faizi M. An Intensified Acrolein Exposure Can Affect Memory and Cognition in Rat. Neurotox Res. 2021;39(2):277-91. [PubMed ID: 32876917]. https://doi.org/10.1007/s12640-020-00278-x.
-
33.
Hosseini SM, Golaghaei A, Nassireslami E, Naderi N, Pourbadie HG, Rahimzadegan M, et al. Neuroprotective effects of lipopolysaccharide and naltrexone co‑preconditioning in the photothrombotic model of unilateral selective hippocampal ischemia in rat. Acta Neurobiol Exp (Wars). 2019;79(1):73-85. [PubMed ID: 31038486].
-
34.
Chaby LE, Sheriff MJ, Hirrlinger AM, Lim J, Fetherston TB, Braithwaite VA. Does Chronic Unpredictable Stress during Adolescence Affect Spatial Cognition in Adulthood? PLoS One. 2015;10(11). e0141908. [PubMed ID: 26580066]. [PubMed Central ID: PMC4651310]. https://doi.org/10.1371/journal.pone.0141908.
-
35.
Ly L. Development of selective electrophoresis for proteins and peptides within proteomes [dissertation]. Sydney, Australia: The University of New South Wales; 2008.
-
36.
Bhang SH, Lee S, Shin JY, Lee TJ, Kim BS. Transplantation of cord blood mesenchymal stem cells as spheroids enhances vascularization. Tissue Eng Part A. 2012;18(19-20):2138-47. [PubMed ID: 22559333]. [PubMed Central ID: PMC3463282]. https://doi.org/10.1089/ten.TEA.2011.0640.
-
37.
Laschke MW, Schank TE, Scheuer C, Kleer S, Schuler S, Metzger W, et al. Three-dimensional spheroids of adipose-derived mesenchymal stem cells are potent initiators of blood vessel formation in porous polyurethane scaffolds. Acta Biomater. 2013;9(6):6876-84. [PubMed ID: 23415749]. https://doi.org/10.1016/j.actbio.2013.02.013.
-
38.
Bartosh TJ, Ylöstalo JH, Mohammadipoor A, Bazhanov N, Coble K, Claypool K, et al. Aggregation of human mesenchymal stromal cells (MSCs) into 3D spheroids enhances their antiinflammatory properties. Proc Natl Acad Sci U S A. 2010;107(31):13724-9. [PubMed ID: 20643923]. [PubMed Central ID: PMC2922230]. https://doi.org/10.1073/pnas.1008117107.
-
39.
Miranda JP, Camões SP, Gaspar MM, Rodrigues JS, Carvalheiro M, Bárcia RN, et al. The Secretome Derived From 3D-Cultured Umbilical Cord Tissue MSCs Counteracts Manifestations Typifying Rheumatoid Arthritis. Front Immunol. 2019;10:18. [PubMed ID: 30804924]. [PubMed Central ID: PMC6370626]. https://doi.org/10.3389/fimmu.2019.00018.
-
40.
Jeong YY, Kim MS, Lee KE, Nam OH, Jang J, Choi S, et al. Comparison of 2- and 3-Dimensional Cultured Periodontal Ligament Stem Cells; a Pilot Study. Appl Sci. 2021;11(3):1083. https://doi.org/10.3390/app11031083.
-
41.
Bellotti C, Duchi S, Bevilacqua A, Lucarelli E, Piccinini F. Long term morphological characterization of mesenchymal stromal cells 3D spheroids built with a rapid method based on entry-level equipment. Cytotechnology. 2016;68(6):2479-90. [PubMed ID: 27023795]. [PubMed Central ID: PMC5101319]. https://doi.org/10.1007/s10616-016-9969-y.
-
42.
Sart S, Tsai AC, Li Y, Ma T. Three-dimensional aggregates of mesenchymal stem cells: cellular mechanisms, biological properties, and applications. Tissue Eng Part B Rev. 2014;20(5):365-80. [PubMed ID: 24168395]. [PubMed Central ID: PMC4185975]. https://doi.org/10.1089/ten.TEB.2013.0537.
-
43.
Bari E, Perteghella S, Di Silvestre D, Sorlini M, Catenacci L, Sorrenti M, et al. Pilot Production of Mesenchymal Stem/Stromal Freeze-Dried Secretome for Cell-Free Regenerative Nanomedicine: A Validated GMP-Compliant Process. Cells. 2018;7(11). [PubMed ID: 30380806]. [PubMed Central ID: PMC6262564]. https://doi.org/10.3390/cells7110190.
-
44.
Paul S, Lombroso PJ. Receptor and nonreceptor protein tyrosine phosphatases in the nervous system. Cell Mol Life Sci. 2003;60(11):2465-82. [PubMed ID: 14625689]. https://doi.org/10.1007/s00018-003-3123-7.
-
45.
Hendriks WJ, Elson A, Harroch S, Pulido R, Stoker A, den Hertog J. Protein tyrosine phosphatases in health and disease. Febs j. 2013;280(2):708-30. [PubMed ID: 22938156]. https://doi.org/10.1111/febs.12000.
-
46.
Park SY, Yoon SN, Kang MJ, Lee Y, Jung SJ, Han JS. Hippocalcin Promotes Neuronal Differentiation and Inhibits Astrocytic Differentiation in Neural Stem Cells. Stem Cell Reports. 2017;8(1):95-111. [PubMed ID: 28017654]. [PubMed Central ID: PMC5233403]. https://doi.org/10.1016/j.stemcr.2016.11.009.
-
47.
Agostinho P, Cunha RA, Oliveira C. Neuroinflammation, oxidative stress and the pathogenesis of Alzheimer's disease. Curr Pharm Des. 2010;16(25):2766-78. [PubMed ID: 20698820]. https://doi.org/10.2174/138161210793176572.
-
48.
Chong ZZ, Maiese K. The Src homology 2 domain tyrosine phosphatases SHP-1 and SHP-2: diversified control of cell growth, inflammation, and injury. Histol Histopathol. 2007;22(11):1251-67. [PubMed ID: 17647198]. [PubMed Central ID: PMC2515712]. https://doi.org/10.14670/hh-22.1251.
-
49.
Gelinas RP, Froman BE, McElroy F, Tait RC, Gorin FA. Human brain glycogen phosphorylase: characterization of fetal cDNA and genomic sequences. Brain Res Mol Brain Res. 1989;6(2-3):177-85. [PubMed ID: 2615594]. https://doi.org/10.1016/0169-328x(89)90052-1.
-
50.
Mathieu C, Li de la Sierra-Gallay I, Duval R, Xu X, Cocaign A, Léger T, et al. Insights into Brain Glycogen Metabolism: THE STRUCTURE OF HUMAN BRAIN GLYCOGEN PHOSPHORYLASE. J Biol Chem. 2016;291(35):18072-83. [PubMed ID: 27402852]. [PubMed Central ID: PMC5000057]. https://doi.org/10.1074/jbc.M116.738898.
-
51.
Zhu H, Sun A, Zou Y, Ge J. Inducible metabolic adaptation promotes mesenchymal stem cell therapy for ischemia: a hypoxia-induced and glycogen-based energy prestorage strategy. Arterioscler Thromb Vasc Biol. 2014;34(4):870-6. [PubMed ID: 24558105]. https://doi.org/10.1161/atvbaha.114.303194.
-
52.
Pinacho R, Vila E, Prades R, Tarragó T, Castro E, Ferrer I, et al. The glial phosphorylase of glycogen isoform is reduced in the dorsolateral prefrontal cortex in chronic schizophrenia. Schizophr Res. 2016;177(1-3):37-43. [PubMed ID: 27156240]. https://doi.org/10.1016/j.schres.2016.04.024.
-
53.
Migocka-Patrzałek M, Elias M. Muscle Glycogen Phosphorylase and Its Functional Partners in Health and Disease. Cells. 2021;10(4). [PubMed ID: 33924466]. [PubMed Central ID: PMC8070155]. https://doi.org/10.3390/cells10040883.
-
54.
Ilieş I, Zupanc MM, Zupanc GK. Proteome analysis reveals protein candidates involved in early stages of brain regeneration of teleost fish. Neuroscience. 2012;219:302-13. [PubMed ID: 22659563]. https://doi.org/10.1016/j.neuroscience.2012.05.028.
-
55.
Chen Z, Zhong C. Decoding Alzheimer's disease from perturbed cerebral glucose metabolism: implications for diagnostic and therapeutic strategies. Prog Neurobiol. 2013;108:21-43. [PubMed ID: 23850509]. https://doi.org/10.1016/j.pneurobio.2013.06.004.