Keywords
Neurobiophysics Neuromodulation Deep Brain Stimulation Wireless Modulation
Dear Editor,
Neurobiophysics is an interdisciplinary science and branch of biophysics, in which physical principles and techniques are used to manipulate the nervous system at physiological, cellular, and molecular levels (1, 2). Efforts have been made in this field to produce macroscopic outcomes, which usually are our everyday cognitive processes or, in some cases, pathologies, such as epilepsy, Alzheimer's disease, multiple sclerosis, etc. Non-invasive neuromodulation using physical approaches induces neuronal excitability that is important to decrease chronic pain and sensory dysfunctions. Developing non-invasive methods to allow the control of functions in the nervous system can potentially revolutionize regenerative medicine (3). To address this unmet need, we should research about artificial biological “switch” for the activation of neuron change affected by magnetic fields as a non-invasive modulator (Figure 1). There are two strategies that can be used to solve this problem: using magnetic biomaterials and magneto sensing proteins.
Wireless deep brain stimulation by magnetic fields via magnetic multifunctional nanoparticles and/or magnetosensitive proteins represents a novel non-invasive strategy for targeted control of the brain in neuromodulation therapies.
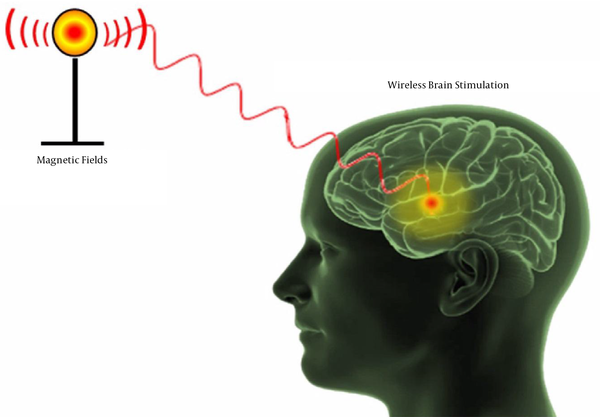
Strategies:
1- Using molecular dynamic simulation to identify the function of proteins in the nervous system in two states: healthy and injury or damages.
2- Designing magnetic nanoparticles or biological magnetoreceptors to remote control of neurons and deep brain stimulation by electromagnetic fields.
3- Classification of the nervous system injuries by computational biology and meta-analysis.
Focused areas:
1- Biophysics
2- Biomagnetism
3- Magnetogenetics
4- Biomaterials
5- Synthetic biology
6- Biomolecular engineering
7- Computational biology (structural biology and neuroinformatics)
8- Neurobiology
9- Neuromodulation
10- Deep brain stimulation
Engineered magnetic nanoparticles can be used in various environmental, biomedical, and clinical applications with unique properties (4). The effect of various magnetic fields on magnetic nanoparticles can, directly and indirectly, induce cellular stimulation. Heat, mechanical force, and production of free radicals are some of the direct effects of magnetic fields. One of the important roles of the indirect effect of magnetic fields on magnetic nanoparticles is the drug release control for local therapy (5, 6).
Cell stimulation using magnetic fields is a non-invasive approach to create a specific function that can penetrate to tissue depth and cells (7). It is used to stimulate deep areas of the brain for therapeutic purposes in various neurological disorders, including Alzheimer's disease, Parkinson's disease, vascular dementia, depressive disorder, etc. (8). Magnetic fields are divided into the static magnetic field, time-varying/dynamic magnetic field, or electromagnetic fields. The effects of magnetic fields depend on some parameters, such as source, intensity, frequency, as well as pulse sequence patterns (9). Magnetic fields affect cell function and physiological behaviors in two main ways: direct and unmediated effects, such as changing the rates of enzymatic and non-enzymatic reactions, the resting membrane potential, gene expression, and affecting the kinetics of radical pair recombination, etc., and the use of magnetoreceptors (10). Generally, magnetoreceptors are divided into two classes: artificial receptors and biomolecules (11, 12).
Ion channels as “messenger converter” are the best choice to control behaviors of cells and specifically, neurons. Using channels, like all cells, neurons are able to encode a variety of external stimuli into electrochemical messages so that the cell can respond to any changes in the environment (13). There are different types of channels distributed in each cell of the whole body that generate a concentration gradient of ions across biological membranes. When the cell is in the non-excited state, the electrical potential difference across the plasma membrane is called the resting membrane potential, which is varied in different cells. The resting membrane potential of a neuron is about -70 mV that it is caused by differences in the concentrations of ions inside and outside the cell. After cell stimulation, if cell membranes allow ions to cross and make membrane potential reach threshold, an action potential will be initiated. Indeed, the excitability of neurons is regulated by voltage-gated, ligand-gated, temperature-sensitive, pH-sensitive, and mechano-sensitive ion channels, etc. (14). Studies have demonstrated that mechano-sensitive and temperature-sensitive ion channels can be excited by magnetic fields (15). The firing of an action potential allows the cell to communicate with other cells through the release of neurotransmitters depending on calcium ion concentrations.
Calcium is an important second messenger that regulates some of the signaling pathways and events in the cell, like gene expression, differentiation, proliferation, cell death, sensing, and fertilization, etc. Changes in the intracellular calcium through calcium-dependent channels (calcium influx) are a very fast and localized process near the cytosolic mouth of the channels. Indeed, some physiological processes depend on the uniform and localized calcium in cells (16, 17). Therefore, we can send different messages to the cell through the stimulation of different ion channels such as calcium by magnetic fields.
References
-
1.
Marino AA, Iliev IG. Neurobiophysics. digital Encyclopedia of Applied Physics; 2003.
-
2.
Kolomytkin OV, Marino AA. Neurobiophysics. digital Encyclopedia of Applied Physics; 2003.
-
3.
Biagioni MC, Sharma K, Migdadi HA, Cucca A. Non-invasive neuromodulation therapies for parkinson’s disease. Parkinson's Disease - Understanding Pathophysiology and Developing Therapeutic Strategies. 2018.
-
4.
Mohammed L, Gomaa HG, Ragab D, Zhu J. Magnetic nanoparticles for environmental and biomedical applications: A review. Particuology. 2017;30:1-14. https://doi.org/10.1016/j.partic.2016.06.001.
-
5.
Zablotskii V, Pastor JM, Larumbe S, Pérez-Landazábal JI, Recarte V, Gómez-Polo C, et al. High-field gradient permanent micromagnets for targeted drug delivery with magnetic nanoparticles. American Institute of Physics; 2010. p. 152-7.
-
6.
Zablotskii V, Polyakova T, Lunov O, Dejneka A. How a high-gradient magnetic field could affect cell life. Sci Rep. 2016;6:37407. [PubMed ID: 27857227]. [PubMed Central ID: PMC5114642]. https://doi.org/10.1038/srep37407.
-
7.
Zwolinska J, Gasior M, Sniezek E, Kwolek A. The use of magnetic fields in treatment of patients with rheumatoid arthritis. Review of the literature. Reumatologia. 2016;54(4):201-6. [PubMed ID: 27826175]. [PubMed Central ID: PMC5090029]. https://doi.org/10.5114/reum.2016.62475.
-
8.
Riancho J, Sanchez de la Torre JR, Paz-Fajardo L, Limia C, Santurtun A, Cifra M, et al. The role of magnetic fields in neurodegenerative diseases. Int J Biometeorol. 2021;65(1):107-17. [PubMed ID: 32198562]. https://doi.org/10.1007/s00484-020-01896-y.
-
9.
Humans I. Non-ionizing Radiation:Static and extremely low-frequency (ELF) electric and magnetic fields. IARC Monographs on the Evaluation of Carcinogenic Risks to Humans Volume 80. WHO, Cancer IAfRo; 2002.
-
10.
Zhang X, Yarema K, Xu A. Biological effects of static magnetic fields. Springer; 2017.
-
11.
Nordmann GC, Hochstoeger T, Keays DA. Magnetoreception-A sense without a receptor. PLoS Biol. 2017;15(10). e2003234. [PubMed ID: 29059181]. [PubMed Central ID: PMC5695626]. https://doi.org/10.1371/journal.pbio.2003234.
-
12.
Christiansen MG, Senko AW, Anikeeva P. Magnetic strategies for nervous system control. Annu Rev Neurosci. 2019;42:271-93. [PubMed ID: 30939100]. [PubMed Central ID: PMC6617523]. https://doi.org/10.1146/annurev-neuro-070918-050241.
-
13.
Barbic M. Possible magneto-mechanical and magneto-thermal mechanisms of ion channel activation in magnetogenetics. Elife. 2019;8. [PubMed ID: 31373554]. [PubMed Central ID: PMC6693891]. https://doi.org/10.7554/eLife.45807.
-
14.
Lamas JA, Rueda-Ruzafa L, Herrera-Perez S. Ion channels and thermosensitivity: TRP, TREK, or both? Int J Mol Sci. 2019;20(10). [PubMed ID: 31091651]. [PubMed Central ID: PMC6566417]. https://doi.org/10.3390/ijms20102371.
-
15.
Meister M. Physical limits to magnetogenetics. Elife. 2016;5. [PubMed ID: 27529126]. [PubMed Central ID: PMC5016093]. https://doi.org/10.7554/eLife.17210.
-
16.
Arredouani A, Yu F, Sun L, Machaca K. Regulation of store-operated Ca2+ entry during the cell cycle. J Cell Sci. 2010;123(Pt 13):2155-62. [PubMed ID: 20554894]. [PubMed Central ID: PMC2886739]. https://doi.org/10.1242/jcs.069690.
-
17.
Hodeify R, Yu F, Courjaret R, Nader N, Dib M, Sun L, et al. regulation and role of store-operated Ca(2+) entry in cellular proliferation. In: Kozak JA, Putney JJ, editors. Calcium Entry Channels in Non-Excitable Cells. Boca Raton (FL); 2018. p. 215-40.