Abstract
Background:
This study presents a unique investigation into the relationship between the persistence of IgG antibody levels and reinfection in recovered coronavirus disease 2019 (COVID-19) patients. By shedding light on this aspect, we aim to address concerns about the risk of reinfection in unvaccinated patients, offering a fresh perspective in the current COVID-19 research landscape.Objectives:
This study takes a novel approach by focusing on the antibody response and reinfection in COVID-19 patients who were treated in outpatient settings for six months. This approach distinguishes our research within the broader context of COVID-19 studies.Methods:
Our comprehensive and rigorous prospective cohort study included 149 COVID-19 outpatients referred to Imam Ali Clinic in Dezfoul City, Khuzestan province, Iran. Data was meticulously collected from September 2021 to February 2022. All unvaccinated patients underwent RT-PCR testing, and their anti-SARS-CoV-2 IgM/IgG levels were measured at the onset of illness. Serum IgG levels were then measured again after three and six months using ELISA.Results:
All COVID-19 patients had their IgG antibody levels tested three times (on days two to four of the illness, at three months, and at six months). Significant differences in IgG antibody levels were observed between the vaccinated and unvaccinated groups during each period (P < 0.05). Additionally, there were substantial differences between the hypertension and non-hypertension groups (P = 0.032). Importantly, our findings reaffirm the impartiality of our research, as no significant differences were found between reinfection rates and various demographic or disease-related factors.Conclusions:
The findings of this study suggest that high levels of IgG antibodies in unvaccinated patients could potentially reduce the risk of reinfection. This insight could have important implications for public health strategies and the management of COVID-19 in unvaccinated populations, providing valuable information for decision-makers in the field.Keywords
1. Background
In December 2019, a respiratory disease outbreak caused by a novel coronavirus (2019-nCoV) began in Wuhan, China, eventually leading to a global pandemic (1). The virus was subsequently named SARS-CoV-2 by the International Committee of Taxonomy of Viruses (ICTV). It causes the disease known as coronavirus disease 2019 (COVID-19) (2). Coronaviruses (CoVs) are single-stranded positive-sense RNA viruses belonging to the Coronaviridae family and the order Nidovirales. They are widely distributed in humans and other mammals (3). The primary sources of the virus are asymptomatic carriers infected with 2019-nCoV (4). It is believed that 2019-nCoV can be transmitted through respiratory droplets, close contact, and the fecal-oral route (4-6). The incubation period of 2019-nCoV infection ranges from 1 to 14 days, typically between 3 to 7 days (7). The disease presents with clinical symptoms of viral pneumonia, with fever, fatigue, and dry cough as the primary symptoms (5, 8). In more severe cases, dyspnea may occur. Additionally, patients can develop acute respiratory distress syndrome, septic shock, difficulty compensating for metabolic acidosis, and coagulation dysfunction (9). Approximately 20% of cases are severe, with a mortality rate of around 3% (10).
Following exposure to infectious agents like viruses, the humoral immune system responds by producing specific antibodies to neutralize the virus and prevent further spread. Innate immune cells, such as macrophages, process the viral agents and present them to lymphocytes in specialized lymph nodes and the spleen. Initially, IgM antibodies are produced, followed by B lymphocytes differentiating into plasma cells, which primarily produce IgG or IgA antibodies as the immune response progresses (11). Studies have shown that in the early stages of the immune response to COVID-19, IgM antibody production is predominant, although the quantity produced is low, and the duration of production is short.
On the other hand, the production of IgG occurs with a delay, but it results in higher antibody levels due to the formation of immune memory. These IgG antibodies remain in the bloodstream for an extended period, even after the infection has resolved. The presence of IgM in a suspect's serum can indicate a recent infection, while detecting IgG in a person without clinical symptoms often suggests a past infection. Additionally, in patients who recover successfully, IgM levels usually decline over time (12). Typically, individuals infected with the coronavirus show positive antibody levels 10 - 21 days after infection, although in some cases, this may take longer (13). While the first infection with certain viruses can provide lifelong immunity, protective immunity against seasonal viruses is often short-lived. A key question in predicting the course of the COVID-19 pandemic is how long immune responses protect individuals from reinfection.
A review of the literature indicates that no study has previously examined the antibody response and reinfection rates in recovered COVID-19 patients in Iran.
2. Objectives
Therefore, this study aims to fill that gap by investigating the antibody response and reinfection rates in COVID-19 patients over six months in an outpatient setting.
3. Methods
3.1. Patient Selection
This prospective cohort study was conducted on COVID-19 patients with a confirmed positive RT-PCR diagnostic test for the novel COVID-19 virus. The research included 149 COVID-19 patients who were referred to Imam Ali (a.s.) Clinic, exhibiting disease symptoms such as a positive PCR test or pulmonary involvement, as well as clinical symptoms including loss of smell and taste. The patients in this study were selected through simple random sampling.
3.2. Inclusion and Exclusion Criteria
The inclusion criteria for the study were outpatients who had previously been infected with the coronavirus, had recovered, and showed increased IgG and IgM antibody levels. Patients without a documented history of antibody levels were excluded from the study.
3.3. Study Design
Patients' information was initially reviewed based on the inclusion and exclusion criteria, and those who were eligible were invited to participate in the study. The purpose and method of cooperation were explained to the qualified volunteers, and written informed consent was obtained from all participants. At baseline, demographic data such as age, gender, Body Mass Index (BMI), blood group, education level, and correlations with diabetes, blood pressure, cardiovascular disease, and the distribution of IgG variable levels at different times, as well as COVID-19 infection status, were documented to identify factors that may affect the SARS-CoV-2 antibody response. Blood specimens were collected in sterile tubes, labeled with the patient's name, and processed in the laboratory of Imam Ali Clinic. Whole blood samples were allowed to clot, then centrifuged at 1000 × g for 10 minutes. The serum was separated and stored frozen at -20°C until analysis. Patients were monitored for six months, during which the antibody levels were measured three times, and their reinfection status was investigated. Antibody levels were determined using an ELISA reader (Stat Fax 2400) and Ideal Tashkhis kits.
3.4. Ethics Statement
The investigation was conducted in accordance with the Ethics Committee Guidelines of Dezfoul University of Medical Sciences (ethics code: IR.DUMS.REC.1400.036).
3.5. Statistical Analysis
All analyses were conducted using SPSS software version 22 (USA, IL, Chicago, SPSS Inc) for Windows. The Kolmogorov–Smirnov test was utilized to assess the normality of the data. Descriptive statistics, including frequency indices, percentages, means, standard deviations, and variances, were used to summarize the data. For normally distributed data, statistical analysis was carried out using chi-square tests, t-tests, and analysis of variance (ANOVA). In cases where the data did not follow a normal distribution, non-parametric tests, such as the chi-square, Mann-Whitney, and Wilcoxon tests, were employed. A type 1 error of 0.05 with a 95% confidence interval was considered in all tests.
4. Results
In this study, 149 participants were examined, of whom 86 (57.7%) were men and 63 (42.3%) were women. Among the 11 individuals who experienced reinfection, 4 (36%) were men, and 7 (63.6%) were women. The results indicated no significant relationship between gender and reinfection (P = 0.204).
The average age of all participants was 44 years, with an average of 43 years in the reinfection group and 44 years in the non-reinfection group. There was no significant relationship between age and reinfection (P = 0.994).
An important anthropometric measure, the BMI, was calculated by measuring the patients' weight and height. The average BMI of all patients was 24.8; in the reinfection group, it was 25.6, and in the non-reinfection group, it was 24.6. No significant relationship was found between BMI and reinfection (P = 0.338).
Out of the 149 patients examined, 49 (32.9%) had blood group A, 29 (19.5%) had blood group B, 26 (17.4%) had blood group AB, and 45 (30.2%) had blood group O. Among the 11 individuals who had reinfections, 3 (27.3%) had blood group A, 3 (27.3%) had blood group B, 1 (9.1%) had blood group AB, and 4 (36.4%) had blood group O. The results showed no significant relationship between blood group and the recurrence of the disease (P = 0.815) (Table 1).
Demographic Findings of the COVID-19 Patient Cohort in This Study a
Variables | Reinfection; (N = 11) | Non-reinfection; (N = 138) | Total; (N = 149) | P-Value |
---|---|---|---|---|
Age | 43 (33.0 - 53.0) | 44 (35.0 - 53.0) | 44 (33.0 - 55.0) | 0.994 |
Gender | 0.204 | |||
Male | 4 (36) | 82 (59.4) | 86 (57.7) | |
Female | 7 (63.6) | 56 (40.6) | 63 (42.3) | |
BMI | 24.8 (23.3 - 27.1) | 25.6 (24.5 - 27.1) | 24.6 (23.3 - 27.1) | 0.338 |
Blood group | 0.815 | |||
A | 3 (27.3) | 46 (33.3) | 49 (32.9) | |
B | 3 (27.3) | 26 (18.8) | 29 (19.5) | |
AB | 1 (9.1) | 25 (18.1) | 26 (17.4) | |
O | 4 (36.4) | 41 (29.7) | 45 (30.2) |
In this study, out of 149 participants, 9 individuals (6%) had diabetes, and all (100%) were in the non-reinfection group. The results indicate no significant relationship between diabetes and the likelihood of reinfection (P = 1.0). Additionally, 17 individuals (11.4%) had hypertension, and of the 11 individuals who experienced reinfection, only one person (9.1%) had hypertension. There was no significant relationship between hypertension and reinfection (P = 1.0). Furthermore, 9 participants (6%) had cardiovascular disease, and among those who experienced reinfection, one person (9.1%) had heart disease. The analysis showed no significant relationship between cardiovascular disease and reinfection (P = 0.508).
Moreover, 65 participants (43.6%) had pulmonary involvement. Of the 11 individuals with reinfection, 6 (54.5%) had lung involvement, while 59 individuals (42.8%) from the non-reinfection group had lung involvement. There was no significant relationship between pulmonary involvement and the likelihood of reinfection (P = 0.534).
Similarly, 101 participants (67.8%) had contact with high-risk and suspected individuals. Of the 11 individuals who experienced reinfection, 7 (63.6%) had contact with high-risk people. The results show no significant relationship between reinfection and contact with high-risk individuals (P = 0.747).
Additionally, 70 participants (47.5%) reported a decreased sense of smell. Among the 11 individuals with reinfection, 5 (45.5%) had a reduced sense of smell. No significant relationship was found between reduced sense of smell and reinfection (P = 1.0). Similarly, 67 participants (45%) reported a reduced sense of taste, and 5 individuals (45.5%) with reinfection experienced decreased taste. The analysis revealed no significant relationship between reduced sense of taste and reinfection (P = 1.0) (Table 2).
Correlation Between Diabetes, Blood Pressure, Cardiovascular Disease, Pulmonary Involvement, Decreased Sense of Smell and Taste and Re-Infection
Variables | Reinfection; (N = 11) | Non-reinfection | Total; (N= 149) | P-Value |
---|---|---|---|---|
Diabetes | 0 | 9 | 9 (6) | 1.0 |
Blood pressure disease | 1 (9.1) | 16 | 17 (11.4) | 1.0 |
Cardiovascular disease | 1 (9.1) | 8 | 9 (6) | 0.508 |
Pulmonary involvement | 6 (54.5) | 59 (42) | 65 (43.6) | 0.534 |
Contact with high-risk people | 7 (63.6) | 59 (42.8) | 101 (67.8) | 0.747 |
Decreased Sense | ||||
Smell | 5 (45.5) | 65 | 70 (47.5) | 1.0 |
Taste | 5 (45.5) | 62 | 67 (45) | 1.0 |
4.1. Average Distribution of IgG Between Diabetic and Non-diabetic Groups and Hypertension
The measurement of the average IgG at different times showed that the mean IgG level in diabetic individuals was 18.15 ± 6.29 (mean ± SD), whereas in non-diabetic individuals, it was 14.01 ± 7.23. The distribution of IgG levels between diabetic and non-diabetic individuals showed no significant difference (P = 0.055). Additionally, the IgG level in individuals with hypertension was 17.24 ± 5.83, while in those without a history of hypertension, it was 13.88 ± 7.31. The results indicate a significant difference in IgG distribution between individuals with hypertension and those without a history of hypertension (P = 0.032).
The average IgG was also measured in each blood group: For blood group A, the mean IgG was 14.10 ± 7.24; for blood group B, it was 13.51 ± 6.68; for blood group AB, it was 14 ± 6.73; and for blood group O, it was 15.08 ± 7.94. The results showed no significant relationship between the average IgG levels and different blood groups (P = 0.882) (Table 3).
Average Distribution of IgG Between Diabetic and Non-diabetic Groups, Hypertension and Non-hypertension, Different Blood Groups a
Variables | The Average IgG | P-Value |
---|---|---|
Diabetes | 0.055 | |
Yes | 18.15 ± 6.29 | |
No | 14.01 ± 7.23 | |
Hypertension | 0.032 | |
Yes | 17.24 ± 5.83 | |
No | 13.88 ± 7.31 | |
Blood groups | 0.882 | |
A | 14.10 ±7.24 | |
B | 13.51 ± 6.68 | |
AB | 14 ± 6.73 | |
O | 15.08 ± 7.94 |
4.2. Distribution of the IgG Variable Level at Different Times
The average IgG levels were measured at three different times: At the outset, three months later, and six months later. At the outset, the average IgG levels in the non-reinfection and reinfection groups were 6.5 and 3.5, respectively (P = 0.003). Three months later, the average IgG levels were 32.5 and 10.4 (P = 0.001), and six months later, they were 4.7 and 1.1 (P = 0.00001), respectively. These results indicate a significant difference in IgG levels between the non-reinfection and reinfection groups over time (Figure 1).
The relationship of IgG levels between the two groups that had re-infection and those that did not at different times.
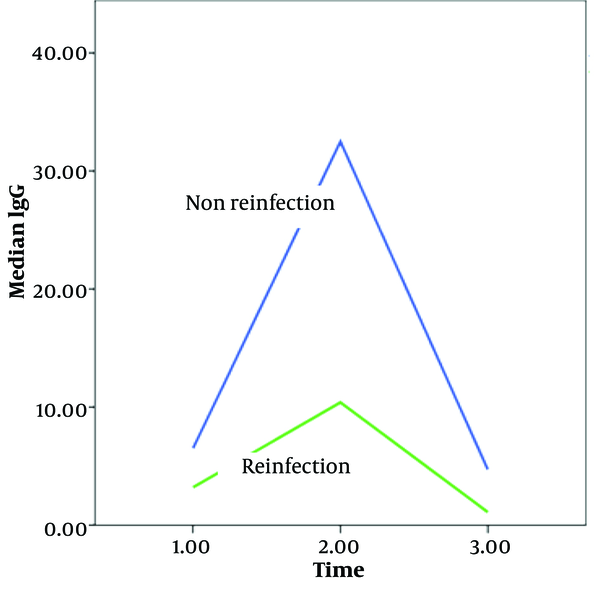
5. Discussion
In recent years, COVID-19 has emerged as a major global health concern. Like many other viral diseases, increasing humoral immunity is critical for combating this virus. The persistence of antibodies following infection is key to evaluating humoral immunity and assessing the risk of reinfection. Research indicates that long-term immunity is more likely if antibody levels rise and remain elevated after a viral infection, underscoring the importance of vaccination.
However, in some COVID-19 patients, a rapid decline in antibody levels and reinfection have been observed. Therefore, the present study aimed to investigate the relationship between relapse and IgG levels, along with various factors such as demographic characteristics, including gender, age, BMI, and blood groups, as well as underlying conditions like diabetes, hypertension, and cardiovascular disease in 149 outpatients who had previously contracted the virus over a six-month period.
In this study, 57.7% of participants were men and 42.3% were women. The mean age of those who experienced reinfection was 43 years, compared to 44 years in the non-reinfection group, with no statistically significant difference. In a similar study involving 326 patients, Zheng et al. (14) examined humoral responses between male and female patients during recovery and found no significant difference in antibody titers between the two groups (P ≥ 0.05). These findings suggest that age and gender are likely unrelated to the duration of antibody titers.
Body Mass Index is often used to assess the risk of various diseases. This study also examined BMI and found no significant difference between patients with and without reinfection. Similarly, a study by Kooistra et al. involving 35,506 ICU patients in the Netherlands found no significant relationship between obesity and mortality (15).
Regarding blood type, unlike a study by Rouhanizadeh et al. that reported individuals with blood type O had a 30% lower risk of contracting COVID-19 compared to other blood types, the present study found no significant difference in this respect. Given the conflicting results in the literature, further research is needed to investigate the role of blood type in COVID-19 infection risk across different populations (16).
Additionally, previous studies revealed that 43.6% of all subjects had pulmonary involvement, with only six individuals falling into the recurrent infection group. No significant relationship was found between pulmonary involvement and recurrent infection in these patients. It is important to note that pulmonary involvement in these cases was not severe; the patients were treated on an outpatient basis, which may have resulted in a lower antibody response. Consequently, these individuals may have been reinfected after a short time due to insufficient antibody levels.
Observational and retrospective studies conducted near the Wuhan area have indicated that hypertension is the most common comorbidity in COVID-19 patients, with prevalence rates ranging from 15% to 30% (17). In the current study, the relationship between underlying conditions such as diabetes, hypertension, and cardiovascular disease with relapse was examined. The findings showed no significant relationship between these conditions and reinfection. However, studies have demonstrated that poor control of these diseases can increase susceptibility to reinfection. For example, uncontrolled high blood pressure has been identified as a potential risk factor for contracting COVID-19. Various organizations have stressed the importance of blood pressure control to reduce the overall disease burden, even if it does not directly impact susceptibility to SARS-CoV-2 infection. Nevertheless, previous studies have shown that hypertensive patients using angiotensin-converting enzyme inhibitors (ACEI) experience more severe hypertension (18).
In a review of hospital records from 280 COVID-19 patients, Aslanbeigi et al. reported that hypertension is associated with poor prognosis and higher mortality rates among COVID-19 patients, underscoring the need for optimal blood pressure management in these cases. They also emphasized the importance of early care and education for elderly patients with hypertension and other comorbidities (19).
In this study, the relationship between diabetes, hypertension, and IgG levels was also explored, and a significant increase in IgG levels was observed in patients with these conditions. These results highlight the importance of specific treatment strategies for hypertension in COVID-19 patients.
Zheng et al. conducted a study on 172 inpatients with COVID-19 in a Wuhan hospital, focusing on the humoral immune response over six months. Their research demonstrated that antibody titers remained high for over six months in recovered patients, suggesting that humoral immunity plays a dynamic role in acquired immunity (14).
In the present study, the antibody levels of participants were measured three times over a six-month period. The average IgG titer in the non-reinfected group was significantly higher than in the reinfected group, and this difference was statistically significant.
5.1. Conclusions
These results suggest that high antibody levels can provide immunity and support the effectiveness of vaccination in protecting against COVID-19. Additionally, the findings indicate that elevated IgG levels in unvaccinated patients may lower the risk of reinfection. Therefore, based on these results, vaccination can play a crucial role in preventing infection and reducing the spread of the disease.
Acknowledgements
References
-
1.
Lai CC, Shih TP, Ko WC, Tang HJ, Hsueh PR. Severe acute respiratory syndrome coronavirus 2 (SARS-CoV-2) and coronavirus disease-2019 (COVID-19): The epidemic and the challenges. Int J Antimicrob Agents. 2020;55(3):105924. [PubMed ID: 32081636]. [PubMed Central ID: PMC7127800]. https://doi.org/10.1016/j.ijantimicag.2020.105924.
-
2.
World Health O. Laboratory testing for coronavirus disease 2019 (COVID-19) in suspected human cases: interim guidance, 2 March 2020. Geneva: World Health Organization; 2020. Report No.: WHO/COVID-19/laboratory/2020.4.
-
3.
Zhu N, Zhang D, Wang W, Li X, Yang B, Song J, et al. A Novel Coronavirus from Patients with Pneumonia in China, 2019. N Engl J Med. 2020;382(8):727-33. [PubMed ID: 31978945]. [PubMed Central ID: PMC7092803]. https://doi.org/10.1056/NEJMoa2001017.
-
4.
Chan JF, Yuan S, Kok KH, To KK, Chu H, Yang J, et al. A familial cluster of pneumonia associated with the 2019 novel coronavirus indicating person-to-person transmission: a study of a family cluster. Lancet. 2020;395(10223):514-23. [PubMed ID: 31986261]. [PubMed Central ID: PMC7159286]. https://doi.org/10.1016/s0140-6736(20)30154-9.
-
5.
Chen ZM, Fu JF, Shu Q, Chen YH, Hua CZ, Li FB, et al. Diagnosis and treatment recommendations for pediatric respiratory infection caused by the 2019 novel coronavirus. World J Pediatr. 2020;16(3):240-6. [PubMed ID: 32026148]. [PubMed Central ID: PMC7091166]. https://doi.org/10.1007/s12519-020-00345-5.
-
6.
Lei J, Li J, Li X, Qi X. CT Imaging of the 2019 Novel Coronavirus (2019-nCoV) Pneumonia. Radiol. 2020;295(1):18. [PubMed ID: 32003646]. [PubMed Central ID: PMC7194019]. https://doi.org/10.1148/radiol.2020200236.
-
7.
Shen K, Yang Y, Wang T, Zhao D, Jiang Y, Jin R, et al. Diagnosis, treatment, and prevention of 2019 novel coronavirus infection in children: experts' consensus statement. World J Pediatr. 2020;16(3):223-31. [PubMed ID: 32034659]. [PubMed Central ID: PMC7090771]. https://doi.org/10.1007/s12519-020-00343-7.
-
8.
Chung M, Bernheim A, Mei X, Zhang N, Huang M, Zeng X, et al. CT Imaging Features of 2019 Novel Coronavirus (2019-nCoV). Radiol. 2020;295(1):202-7. [PubMed ID: 32017661]. [PubMed Central ID: PMC7194022]. https://doi.org/10.1148/radiol.2020200230.
-
9.
Pan Y, Guan H, Zhou S, Wang Y, Li Q, Zhu T, et al. Initial CT findings and temporal changes in patients with the novel coronavirus pneumonia (2019-nCoV): a study of 63 patients in Wuhan, China. Eur Radiol. 2020;30(6):3306-9. [PubMed ID: 32055945]. [PubMed Central ID: PMC7087663]. https://doi.org/10.1007/s00330-020-06731-x.
-
10.
Chang L, Yan Y, Wang L. Coronavirus Disease 2019: Coronaviruses and Blood Safety. Transfus Med Rev. 2020;34(2):75-80. [PubMed ID: 32107119]. [PubMed Central ID: PMC7135848]. https://doi.org/10.1016/j.tmrv.2020.02.003.
-
11.
Liu X, Wang J, Xu X, Liao G, Chen Y, Hu CH. Patterns of IgG and IgM antibody response in COVID-19 patients. Emerg Microbes Infect. 2020;9(1):1269-74. [PubMed ID: 32515684]. [PubMed Central ID: PMC7448841]. https://doi.org/10.1080/22221751.2020.1773324.
-
12.
Zeng F, Dai C, Cai P, Wang J, Xu L, Li J, et al. A comparison study of SARS-CoV-2 IgG antibody between male and female COVID-19 patients: A possible reason underlying different outcome between sex. J Med Virol. 2020;92(10):2050-4. [PubMed ID: 32383183]. [PubMed Central ID: PMC7267228]. https://doi.org/10.1002/jmv.25989.
-
13.
Jia X, Zhang P, Tian Y, Wang J, Zeng H, Wang J, et al. Clinical Significance of an IgM and IgG Test for Diagnosis of Highly Suspected COVID-19. Front Med (Lausanne). 2021;8:569266. [PubMed ID: 33912572]. [PubMed Central ID: PMC8071939]. https://doi.org/10.3389/fmed.2021.569266.
-
14.
Zheng Y, Zhang Q, Ali A, Li K, Shao N, Zhou X, et al. Sustainability of SARS-CoV-2 Induced Humoral Immune Responses in COVID-19 Patients from Hospitalization to Convalescence Over Six Months. Virol Sin. 2021;36(5):869-78. [PubMed ID: 33661489]. [PubMed Central ID: PMC7931792]. https://doi.org/10.1007/s12250-021-00360-4.
-
15.
Kooistra EJ, Brinkman S, van der Voort PHJ, de Keizer NF, Dongelmans DA, Kox M, et al. Body Mass Index and Mortality in Coronavirus Disease 2019 and Other Diseases: A Cohort Study in 35,506 ICU Patients. Crit Care Med. 2022;50(1):e1-e10. [PubMed ID: 34374504]. [PubMed Central ID: PMC8670082]. https://doi.org/10.1097/CCM.0000000000005216.
-
16.
Rouhanizadeh H, Mousavi SA, Yazdani-Charati J, Pourali F, Saeedi M, Ajami A, et al. [Association between COVID-19 Infection and ABO Blood Types in Mazandaran Province, Iran: A Cross-Sectional Study]. J Mazandaran Univ Med Sci. 2021;31(197):35-43. FA.
-
17.
Zeng Z, Sha T, Zhang Y, Wu F, Hu H, Li H, et al. Hypertension in patients hospitalized with COVID-19 in Wuhan, China: a single-center retrospective observational study. MedRxiv. 2020;Preprint. https://doi.org/10.1101/2020.04.06.20054825.
-
18.
Xie J, Tong Z, Guan X, Du B, Qiu H. Clinical Characteristics of Patients Who Died of Coronavirus Disease 2019 in China. JAMA Netw Open. 2020;3(4). e205619. [PubMed ID: 32275319]. [PubMed Central ID: PMC7148440]. https://doi.org/10.1001/jamanetworkopen.2020.5619.
-
19.
Aslanbeigi F, Rahimi H, Malekipour A, Pahlevani H, Najafizadeh M, Ehteram H, et al. Association between hypertension and clinical outcomes in COVID-19 patients: a case-controlled study. Ann Med Surg (Lond). 2023;85(7):3258-63. [PubMed ID: 37427160]. [PubMed Central ID: PMC10328661]. https://doi.org/10.1097/MS9.0000000000000391.