Abstract
Background:
This study investigates the effects of MLR-1023 on cognitive deficiency and oxidative stress in a lipopolysaccharide (LPS)-induced mouse model.Objectives:
The role of PPAR-gamma receptors and the NO- cyclic GMP (cGMP)-KATP channel pathway were examined to identify the likely mechanisms.Methods:
The model was established by LPS injection. Behavioral assays included shuttle box and Y-maze tests. Superoxide dismutase (SOD) activity and malondialdehyde (MDA) levels were then measured in hippocampal tissue. To explore the probable mechanisms, the mice were pre-treated with agonists and antagonists 15 minutes before MLR-1023 (20 - 40 mg/kg, i.p.) administration.Results:
Lipopolysaccharide increased the initial latency (IL) time, while MLR-1023 (40 mg) reduced it. Administration of L-NAME, methylene blue, and glibenclamide with MLR-1023 increased IL duration, while L-arginine, sildenafil, and diazoxide reduced it. Lipopolysaccharide reduced the duration of step-through latency (STL), whereas MLR-1023 increased it. L-NAME, methylene blue, and glibenclamide with MLR-1023 reduced STL duration, but L-arginine, sildenafil, and diazoxide increased it. The spontaneous alternation index decreased with LPS, while MLR-1023 increased it. L-NAME, methylene blue, glibenclamide, and GW9662 decreased this index, whereas L-arginine, sildenafil, diazoxide, and pioglitazone increased it. Lipopolysaccharide increased MDA concentration, while MLR-1023 decreased it. L-NAME, methylene blue, and glibenclamide increased MDA concentration, while L-arginine, diazoxide, and pioglitazone decreased it. Lipopolysaccharide reduced SOD activity, which was improved by MLR-1023. L-NAME, methylene blue, glibenclamide, and GW9662 decreased SOD activity, while sildenafil and pioglitazone increased it.Conclusions:
MLR-1023 can improve LPS-induced learning-memory impairment and oxidative stress. The KATP/cGMP/NO pathway and PPARγ receptors are involved in this effect.Keywords
1. Background
Alzheimer’s disease (AD), a neurological disorder, is characterized by cognitive deficits, impaired learning and memory, and decreased psychological and behavioral performance (1). Additionally, research has shown that type 2 diabetes, a global health issue, significantly impacts cognitive functions, particularly memory, mental processing speed, and executive performance (2).
Although several studies indicate an association between type 2 diabetes (DM) and AD, the underlying mechanisms remain unclear (3). Due to this critical correlation, numerous investigations have been conducted on diabetic drugs to explore their potential neuroprotective effects. However, medications targeting diabetic neuroinflammation are still inadequate, necessitating further research (4). Cognitive dysfunction is a significant complication of diabetes mellitus, yet no clinically approved drug exists for this issue (5). Studies confirm the involvement of oxidative stress in the brains of AD patients, and oxidative stress appears to be a primary precursor of cognitive decline (6).
Several medications are available for managing AD. Neurotransmitters play a key role in the brain, and pathological changes in neurotransmitter function occur during the progression of AD. Drugs such as galantamine, memantine, donepezil, and others targeting neurotransmitters are used in AD treatment. Studies show that these medications can significantly improve cognitive deficits in patients (7). However, they are not completely successful, highlighting the need for more effective drugs to prevent or treat AD.
MLR-1023 (CP-26154, Tolimidone) is a Lyn-kinase activator known for its blood glucose-decreasing properties, making it a potential new treatment for type 2 diabetes (8). It has been reported that MLR-1023 enhances insulin receptor activity in DM, suggesting that MLR-1023 may work through insulin signaling (9). Lyn kinase is present in tissues such as the brain and adipose tissue and has been shown to play a novel role in the mesolimbic system, controlling mental tasks such as alcohol reward (10).
Since MLR-1023 can directly activate Lyn kinase, it offers a new therapeutic approach that promotes glucose-decreasing effects without affecting insulin secretion. Therefore, it may be beneficial in neurological disorders such as Alzheimer’s disease.
2. Objectives
This research project aims to investigate the effects of MLR-1023 on cognitive deficits and oxidative stress in an lipopolysaccharide (LPS)-induced mouse model of AD. The role of PPAR-gamma receptors and the NO-cyclic GMP (cGMP)-KATP channel pathway will also be examined to elucidate the likely mechanisms.
3. Methods
3.1. Animals
We used 90 NMRI male mice obtained from AJUMS (Jundishapur University of Medical Sciences, Ahvaz, Iran). These mice (weighing 25 - 30 g) had free access to food and water and were kept on a 12-hour light-dark cycle at a temperature of 22 ± 2°C and 80% humidity. The ethics committee of Dezful University of Medical Sciences approved this project (IR.DUMS.REC.1399.043), and all procedures were performed in accordance with the NIH guide for the care and use of laboratory animals (National Institutes of Health Publications No. 80-23, revised 1978) and the ARRIVE guidelines. To ensure reliable scientific data, we reduced the number of mice per group to 6 - 8 animals.
3.2. Drugs
All drugs used in this study are listed here: LPS, MLR-1023, L-NAME (NOS inhibitor), L-arginine (NO precursor), diazoxide (ATP-sensitive K+-channel opener), sildenafil (PDE-5 inhibitor), and glibenclamide (ATP-sensitive K+-channel inhibitor) were obtained from Sigma-Aldrich. Pioglitazone (PPARγ-agonist) was sourced from Osveh Company (Iran), and GW9662 (PPARγ-antagonist) was acquired from Tocris Bioscience (UK). Methylene blue (NOS/guanylyl cyclase blocker) was obtained from Merck. The drugs were diluted with saline, except for glibenclamide, pioglitazone, MLR-1023, and GW9662, which were dissolved in 1% dimethyl sulfoxide (DMSO) and then diluted up to 10 times their initial volume with saline. All drugs were administered via intraperitoneal (i.p.) injection at a dose of 10 mL/kg body weight. The dosages and routes of administration were based on previous studies (11, 12).
3.3. Behavioral Assay
The behavioral assays included the passive avoidance memory test (shuttle box) and the spatial memory test (Y-maze). After the behavioral tests, the mice were euthanized, and their brains were removed for analysis. Superoxide dismutase (SOD) enzyme activity and the amount of malondialdehyde (MDA) in hippocampal tissue samples were measured to evaluate oxidative stress.
3.3.1. Shuttle Box Test
The method suggested by Tamburella, with some modifications, was used for the passive avoidance memory assessment. The shuttle box apparatus, composed of two neighboring boxes of the same dimensions (27 × 14.5 × 14 cm), was used. The floor of the boxes had stainless steel bars (2 mm diameter, spaced 1 cm apart). One section was illuminated by a 5W lamp. The test was conducted over three days. On the first day, mice were allowed to freely explore the light or dark compartment for 10 minutes separately. On the second day, mice were placed in the bright section, and after 10 seconds, the door was opened. The latency time to enter the dark compartment was recorded as the cognitive phase (initial latency). Then, the door was closed, and a low-level electric shock (0.3 mA, 3 sec) was applied when all paws touched the floor grids. After 3 minutes, the mice were returned to their cages. On the third day, the mice were placed in the bright section again. The latency time to enter the dark section was recorded as step-through latency (STL), indicating the learning-memory parameter. The time cut-off was set at 300 seconds, and no shock was applied on this day (13).
3.3.2. Y-maze Test
To examine short-term memory, we used a Y-maze with three arms (A, B, and C) of equal sizes (30 × 5 × 12 cm) with a 120° angle between the arms. Each mouse was tested for 8 minutes. First, each mouse was placed in one arm, and then we recorded the name of every arm the mouse entered. The number of entries per arm was also recorded. The alternation percentage (%) for each mouse was calculated by taking the ratio of the actual number of alternations to the possible number (defined as the total number of arm entries minus two) and multiplying by 100. The total number of arm entries was considered as an indicator of locomotor activity (14, 15).
3.3.3. Measurement of Hippocampal Tissue MDA Level and SOD Activity
The mice were euthanized after deep anesthesia, and their hippocampi were rapidly extracted. These tissues were rinsed with saline and stored at -80°C until analysis with ELISA kits. Each brain tissue sample was weighed and homogenized, then shaken for 90 minutes and centrifuged (at 4°C, 4000 × g, for 15 minutes). The supernatants were collected for analysis (16). ELISA kits for MDA and SOD were purchased from LDN Immunoassays (Germany), and the assays were conducted according to the manufacturer's guidelines.
3.4. Treatments
This project was an experimental laboratory study. As shown in Figure 1, the mouse model of Alzheimer's disease was induced by intraperitoneal injection of LPS. On the first day, all groups were injected with LPS (0.25 mg/kg, 0.1 mL/10 g body weight, dissolved in saline) daily for 7 consecutive days. The control group received a similar quantity of saline instead (15, 17). The first series of experiments aimed to determine the effective dose of MLR-1023 on behavioral assays (shuttle box and Y-maze tests). MLR-1023 at doses of 20, 30, and 40 mg/kg (i.p.) or 10 mL/kg solvent were administered for 14 consecutive days, 30 minutes prior to the tests.
Treatments road map

In the subsequent set of experiments, to investigate the potential role of the NO/cGMP/KATP pathway in the effects of MLR-1023, the mice were pre-treated with L-arginine (750 mg/kg, i.p.) or vehicle 30 minutes before the injection of MLR-1023 (40 mg/kg, i.p.). After 30 minutes, the tests were conducted. Additionally, we examined the effects of combined administration of MLR-1023 (40 mg/kg, i.p.) with L-NAME (10 mg/kg, i.p., a nonselective NO synthase inhibitor) (18). The role of cGMP was assessed by injecting methylene blue (20 mg/kg, i.p.) or vehicle 30 minutes before MLR-1023 (40 mg/kg, i.p.). We also evaluated the effect of combining an effective dose of MLR-1023 (40 mg/kg) with a sub-effective dose of sildenafil (5 mg/kg, i.p.) (19).
To assess the role of K+ channel gating, animals were pre-treated with glibenclamide (1 mg/kg, i.p.) and, 30 minutes later, they received MLR-1023 (40 mg/kg). Additionally, mice received diazoxide (5 mg/kg, i.p.) 30 minutes before MLR-1023 (40 mg/kg). Thirty minutes later, the behavioral tests were conducted (20).
To designate the potential role of PPARγ receptors, a PPARγ receptor agonist, pioglitazone (5 mg/kg, i.p.), was injected 30 minutes before MLR-1023 (40 mg/kg). Additionally, we administered GW9662 (2 mg/kg, i.p.) 30 minutes prior to the injection of MLR-1023 or solvent (18, 21).
3.5. Statistical Analysis
The data were presented as means ± SEM. Normality of the data was evaluated using the Kolmogorov-Smirnov test. All data followed a normal distribution. Statistical analysis was performed using SPSS version 22. One-way ANOVA with Tukey’s post hoc test (LSD in some cases) was used for intergroup comparisons. A P-value of less than 0.05 was considered significant.
4. Results
4.1. Effect of MLR-1023 on Passive Avoidance Task in Shuttle Box Test
Lipopolysaccharide injection significantly increased the initial latency (IL) time (P < 0.05) and decreased the STL time (P < 0.001) compared to the control group in the shuttle box test (Figure 2A). MLR-1023 significantly decreased IL (P < 0.05) and increased STL (P < 0.01) only at the dose of 40 mg/kg (Figure 2A). To evaluate the probable role of the NO/cGMP/KATP pathway and PPARγ receptors in the effects of MLR-1023, mice were pre-treated with the related agonists and antagonists mentioned below. These agonists and antagonists did not have a significant effect on IL and STL times when used alone.
Effect of administration of MLR-1023 (20, 30 and 40 mg/kg, i.p.) in the initial latency (IL) and step-through latency (STL) time in the shuttle box test (A); spontaneous alternation percentage in Y-maze test (B) versus control group in mice. Values are expressed as mean ± SEM. (n = 6). * P < 0.05 and *** P < 0.001 compared with the control group (one-way ANOVA followed by Tukey's test). # P < 0.05 and ## P < 0.01 compared with the lipopolysaccharide (LPS) + vehicle treated group (one-way ANOVA followed by Tukey's test).
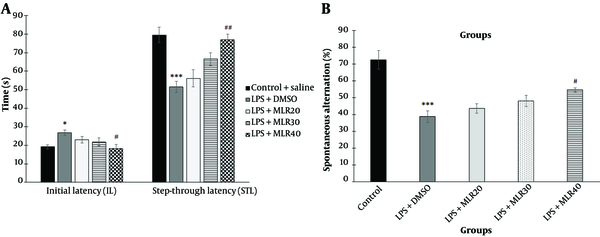
4.2. Involvement of NO in the Effect of MLR-1023 on Passive Avoidance Task in Shuttle box Test
Pre-treatment with L-NAME (10 mg/kg, i.p) significantly increased the IL (P < 0.01) and decreased the STL (P < 0.01) compared to the LPS + MLR-1023 (40 mg/kg, i.p.) treated group. In contrast, pre-treatment with L-arginine (750 mg/kg, i.p.) significantly decreased the IL (P < 0.05) and increased the STL (P < 0.05) compared to the LPS + MLR-1023 (40 mg/kg, i.p.) treated group (Figure 3A).
Involvement of NO/cyclic GMP (cGMP)/KATP channels pathway and PPARγ receptors in the effect of MLR-1023 on the initial latency (IL) and step-through latency (STL) in shuttle box test, are shown in panels A, B, C and D respectively. Values are expressed as mean ± SEM. (n = 6). * P < 0.05, ** P < 0.01 compared with the lipopolysaccharide (LPS) + MLR-1023 (40 mg/kg, i.p.) treated group, as determined by one-way ANOVA followed by Tukey’s (LSD in some cases) post hoc test.
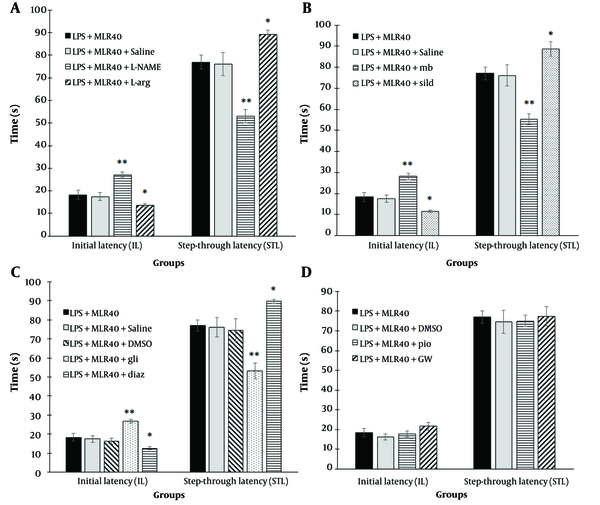
4.3. Involvement of cGMP in the Effect of MLR-1023 on Passive Avoidance Task in Shuttle Box Test
Pre-treatment with methylene blue (20 mg/kg, i.p.) significantly increased the IL (P < 0.01) and decreased the STL (P < 0.01) compared to the LPS + MLR-1023 (40 mg/kg, i.p.) treated group. In contrast, pre-treatment with sildenafil (5 mg/kg, i.p.) significantly decreased the IL (P < 0.05) and increased the STL (P < 0.05) compared to the LPS + MLR-1023 (40 mg/kg, i.p.) treated group (Figure 3B).
4.4. Involvement of KATP Channels in the Effect of MLR-1023 on Passive Avoidance Task in Shuttle Box Test
As shown in Figure 3C, pretreatment with glibenclamide (1 mg/kg, i.p., a KATP channel blocker) significantly increased the IL (P < 0.01) and decreased the STL (P < 0.01) compared to the LPS + MLR-1023 (40 mg/kg, i.p.) treated group. In contrast, pretreatment with diazoxide (10 mg/kg, i.p., a KATP channel opener) significantly decreased the IL (P < 0.05) and increased the STL (P < 0.05) compared to the LPS + MLR-1023 (40 mg/kg, i.p.) treated group.
4.5. Involvement of PPARγ Receptors in the Effect of MLR-1023 on Passive Avoidance Task in Shuttle Box Test
As shown in Figure 3D, pre-treatment with pioglitazone (5 mg/kg, i.p., a PPARγ receptor agonist) and GW9662 (2 mg/kg, i.p., a PPARγ receptor antagonist) did not have any significant effect in the shuttle box test.
4.6. Effect of MLR-1023 on Learning-Memory in Y-maze Test
There was no difference in total arm entries between groups. Lipopolysaccharide injection significantly decreased the spontaneous alternation percentage compared to the control group (P < 0.001) in the Y-maze test (Figure 2B). MLR-1023 significantly increased the spontaneous alternation percentage (P < 0.05) only at the dose of 40 mg/kg (Figure 2B). To assess the likely role of the NO-cGMP-KATP pathway and PPARγ receptors in the effects of MLR-1023, mice were pre-treated with related agonists and antagonists as mentioned below. These agonists and antagonists did not have a significant effect on the spontaneous alternation percentage when used alone.
4.7. Involvement of NO in the Effect of MLR-1023 on Learning-Memory in Y-maze Test
Pre-treatment with L-NAME (10 mg/kg, i.p.) significantly decreased the spontaneous alternation percentage (P < 0.05) compared to the LPS + MLR-1023 (40 mg/kg, i.p.) treated group. In contrast, pre-treatment with L-arginine (750 mg/kg, i.p.) significantly increased the spontaneous alternation percentage (P < 0.05) compared to the LPS + MLR-1023 (40 mg/kg, i.p.) treated group (Figure 4A).
Involvement of NO/cyclic GMP (cGMP)/KATP channels pathway and PPARγ receptors in the effect of MLR-1023 on learning-memory in Y-maze test, are shown in panels A, B, C and D respectively. Values are expressed as mean ± SEM. (n = 6). * P < 0.05, ** P < 0.01 compared with the lipopolysaccharide (LPS) + MLR-1023 (40 mg/kg, i.p.) treated group, as determined by one-way ANOVA followed by Tukey’s post hoc test.
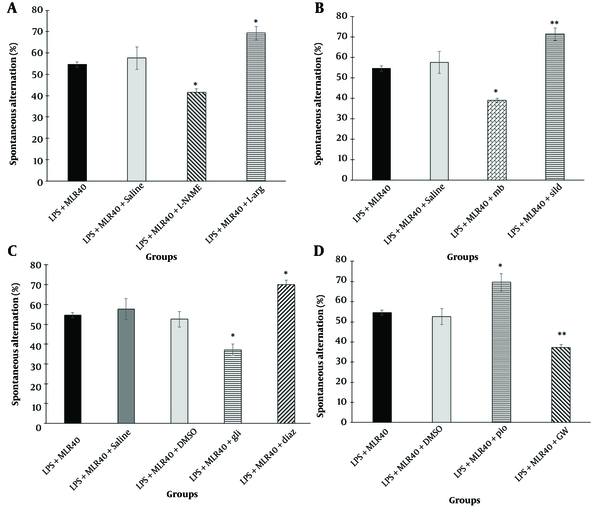
4.8. Involvement of cGMP in the Effect of MLR-1023 on Learning-Memory in Y-maze Test
Pre-treatment with methylene blue (20 mg/kg, i.p.) significantly decreased the spontaneous alternation percentage (P < 0.05) compared to the LPS + MLR-1023 (40 mg/kg, i.p.) treated group. Conversely, pre-treatment with sildenafil (5 mg/kg, i.p.) significantly increased the spontaneous alternation percentage (P < 0.01) compared to the LPS + MLR-1023 (40 mg/kg, i.p.) treated group (Figure 4B).
4.9. Involvement of KATP Channels in the Effect of MLR-1023 on Learning-Memory in Y-maze Test
As shown in Figure 4C, pre-treatment with glibenclamide (1 mg/kg, i.p., a KATP channel blocker) significantly decreased the spontaneous alternation percentage (P < 0.05) compared to the LPS+MLR-1023 (40 mg/kg, i.p.) treated group. Conversely, pre-treatment with diazoxide (10 mg/kg, i.p., a KATP channel opener) significantly increased the spontaneous alternation percentage (P < 0.05) compared to the LPS + MLR-1023 (40 mg/kg, i.p.) treated group.
4.10. Involvement of PPARγ Receptors in the Effect of MLR-1023 on Learning-Memory in Y-maze Test
As shown in Figure 4D, pre-treatment with pioglitazone (5 mg/kg, i.p., a PPARγ receptor agonist) significantly increased the spontaneous alternation percentage (P < 0.05) compared to the LPS + MLR-1023 (40 mg/kg, i.p.) treated group. In contrast, pre-treatment with GW9662 (2 mg/kg, i.p., a PPARγ receptor antagonist) significantly decreased the spontaneous alternation percentage (P < 0.01) compared to the LPS + MLR-1023 (40 mg/kg, i.p.) treated group.
4.11. Effect of MLR-1023 on the Brain Tissue Lipid Peroxidation
In this study, MDA, a lipid peroxidation marker, was measured in hippocampal tissue. Lipopolysaccharide injection significantly increased the levels of MDA in the brain tissue compared to the control group (P < 0.001). MLR-1023 significantly decreased the levels of MDA only at the dose of 40 mg/kg (P < 0.01) (Figure 5A). To investigate the possible involvement of the NO/cGMP/KATP pathway and PPARγ receptors, mice were pre-treated with related agonists and antagonists mentioned below. These agonists and antagonists did not have a significant effect on the levels of MDA when used alone.
Effect of MLR-1023 (20, 30 and 40 mg/kg, i.p.) on the levels of malondialdehyde (MDA) (A); and superoxide dismutase (SOD) enzyme activity (B) versus control group in the mice brain tissue. Values are expressed as mean ± SEM. (n = 6). *** P < 0.001 compared with the vehicle-treated control group (one-way ANOVA followed by Tukey's test). # P < 0.05, ## P < 0.01 compared with the lipopolysaccharide (LPS) + vehicle treated group (one-way ANOVA followed by Tukey's test).

4.12. Involvement of NO in the Effect of MLR-1023 on the Brain Tissue Lipid Peroxidation
Pre-treatment with L-NAME (10 mg/kg, i.p) increased (P < 0.05) the levels of MDA compared to the LPS + MLR-1023 (40 mg/kg, i.p.) treated group. In contrast, pre-treatment with L-arginine (750 mg/kg, i.p.) decreased (P < 0.05) the levels of MDA compared to the LPS + MLR-1023 (40 mg/kg, i.p.) treated group (Figure 6A).
Involvement NO/cyclic GMP (cGMP)/KATP channels pathway and PPARγ receptors in the effect of MLR-1023 on hippocampal tissue lipid peroxidation [levels of malondialdehyde (MDA)] are shown in panels A, B, C and D respectively. Values are expressed as mean ± SEM. (n = 6). * P < 0.05, ** P < 0.01 compared with the lipopolysaccharide (LPS) + MLR-1023 (40 mg/kg, i.p.) treated group, as determined by one-way ANOVA followed by Tukey’s post hoc test.
![Involvement NO/cyclic GMP (cGMP)/KATP channels pathway and PPARγ receptors in the effect of MLR-1023 on hippocampal tissue lipid peroxidation [levels of malondialdehyde (MDA)] are shown in panels A, B, C and D respectively. Values are expressed as mean ± SEM. (n = 6). * P < 0.05, ** P < 0.01 compared with the lipopolysaccharide (LPS) + MLR-1023 (40 mg/kg, i.p.) treated group, as determined by one-way ANOVA followed by Tukey’s post hoc test. Involvement NO/cyclic GMP (cGMP)/KATP channels pathway and PPARγ receptors in the effect of MLR-1023 on hippocampal tissue lipid peroxidation [levels of malondialdehyde (MDA)] are shown in panels A, B, C and D respectively. Values are expressed as mean ± SEM. (n = 6). * P < 0.05, ** P < 0.01 compared with the lipopolysaccharide (LPS) + MLR-1023 (40 mg/kg, i.p.) treated group, as determined by one-way ANOVA followed by Tukey’s post hoc test.](https://services.brieflands.com/cdn/serve/316a8/8c9cd3deaa9366735ae3c0ef08a73f9cb0a55aab/jai-4-2-149170-i006-preview.png)
4.13. Involvement of cGMP in the Effect of MLR-1023 on the Brain Tissue Lipid Peroxidation
As shown in Figure 6B, pre-treatment with methylene blue (20 mg/kg, i.p.) increased (P < 0.05) the levels of MDA compared to the LPS + MLR-1023 (40 mg/kg, i.p.) treated group. In contrast, pre-treatment with sildenafil (5 mg/kg, i.p.) did not have a significant effect on the levels of MDA.
4.14. Involvement of KATP Channels in the Effect of MLR-1023 on the Brain Tissue Lipid Peroxidation
As shown in Figure 6C, pre-treatment with glibenclamide (1 mg/kg, i.p., a KATP channel blocker) led to an increase (P < 0.01) in the levels of MDA compared to the LPS + MLR-1023 (40 mg/kg, i.p.) treated group. In contrast, pre-treatment with diazoxide (10 mg/kg, i.p., a KATP channel opener) led to a decrease (P < 0.05) in the levels of MDA compared to the LPS + MLR-1023 (40 mg/kg, i.p.) treated group.
4.15. Involvement of PPARγ Receptors in the Effect of MLR-1023 on the Brain Tissue Lipid Peroxidation
Pre-treatment with pioglitazone (5 mg/kg, i.p., a PPARγ receptor agonist) decreased the levels of MDA compared to the LPS+MLR-1023 (40 mg/kg, i.p.) treated group (P < 0.05). However, pre-treatment with GW9662 (2 mg/kg, i.p., a PPARγ receptor antagonist) did not have a significant effect on the levels of MDA (Figure 6D).
4.16. Effect of MLR-1023 on SOD Activity in the Brain Tissue
Changes in SOD enzyme activity (u/mL) were measured in hippocampal tissue as an antioxidant parameter. Lipopolysaccharide treatment significantly decreased SOD activity in brain tissue compared to the control group (P < 0.001). MLR-1023 significantly increased SOD activity only at the dose of 40 mg/kg (P < 0.05) (Figure 5B). To study the possible involvement of the NO-cGMP-KATP pathway and PPARγ receptors, mice were pre-treated with the related agonists and antagonists mentioned below. These agonists and antagonists did not have a significant effect on hippocampal tissue SOD activity when used alone.
4.17. Involvement of NO in the Effect of MLR-1023 on SOD Activity in the Brain Tissue
Pre-treatment with L-NAME (10 mg/kg, i.p.) decreased SOD activity compared to the LPS + MLR-1023 (40 mg/kg, i.p.) treated group (P < 0.05). In contrast, pre-treatment with L-arginine (750 mg/kg, i.p.) did not have a significant effect on hippocampal tissue SOD activity (Figure 7A).
Involvement of NO/cyclic GMP (cGMP)/KATP channels pathway and PPARγ receptors in the effect of MLR-1023 on hippocampal tissue superoxide dismutase (SOD) activity are shown in panels A, B, C and D respectively. Values are expressed as mean ± SEM. (n = 6). * P < 0.05 compared with the lipopolysaccharide (LPS) + MLR-1023 (40 mg/kg, i.p.) treated group, as determined by one-way ANOVA followed by Tukey’s post hoc test.

4.18. Involvement of cGMP in the Effect of MLR-1023 on SOD Activity in the Brain Tissue
As shown in Figure 7B, pre-treatment with methylene blue (20 mg/kg, i.p.) decreased SOD activity compared to the LPS + MLR-1023 (40 mg/kg, i.p.) treated group (P < 0.05). In contrast, pre-treatment with sildenafil (5 mg/kg, i.p.) increased SOD activity compared to the LPS + MLR-1023 (40 mg/kg, i.p.) treated group (P < 0.05).
4.19. Involvement of KATP Channels in the Effect of MLR-1023 on SOD Activity in the Brain Tissue
As shown in Figure 7C, pretreatment with glibenclamide (1 mg/kg, i.p., a KATP channel blocker) led to a decrease (P < 0.05) in SOD activity compared to the LPS + MLR-1023 (40 mg/kg, i.p.) treated group. In contrast, pre-treatment with diazoxide (10 mg/kg, i.p., a KATP channel opener) did not have a significant effect on hippocampal tissue SOD activity.
4.20. Involvement of PPARγ Receptors in the Effect of MLR-1023 on SOD Activity in the Brain Tissue
Pre-treatment with pioglitazone (5 mg/kg, i.p., a PPARγ receptor agonist) increased SOD activity compared to the LPS + MLR-1023 (40 mg/kg, i.p.) treated group (P < 0.05). However, pre-treatment with GW9662 (2 mg/kg, i.p., a PPARγ receptor antagonist) decreased SOD activity (P < 0.05) compared to the LPS + MLR-1023 (40 mg/kg, i.p.) treated group (Figure 7D).
5. Discussion
In this study, the effects of MLR-1023 on cognitive deficiency and oxidative stress in an LPS-induced mouse model of AD were examined. To investigate the possible involvement of the NO/cGMP/KATP channels pathway and PPARγ receptors in the effects of MLR-1023, mice were pre-treated with related agonists and antagonists that did not have a significant effect when used alone.
It has been shown that LPS induces inflammation that can lead to memory impairment and hippocampal-related disabilities (22). Our results indicated that LPS injection increased the initial IL, reflecting cognitive impairment, and decreased the STL time, reflecting learning-memory impairment, in the passive avoidance task in the shuttle box test. MLR-1023 decreased IL and increased STL at the dose of 40 mg/kg. Thus, we can conclude that MLR-1023 can improve cognitive deficiency and learning-memory impairment induced by LPS.
NO, as a key neurotransmitter, is involved in neurodegenerative disorders such as cognitive impairments (23). It has been reported that the NO-cGMP pathway is involved in some spatial and recognition memory impairments (24). In this study, pre-treatment with L-NAME increased IL and decreased STL, indicating that L-NAME reduced the improving effect of MLR-1023. In contrast, pre-treatment with L-arginine decreased IL and increased STL, showing that L-arginine potentiated the improving effect of MLR-1023. Therefore, it can be inferred that the L-arginine-NO pathway may be involved in the improving effect of MLR-1023 on cognitive deficiency and learning-memory impairments induced by LPS in the passive avoidance task in the shuttle box test.
Previous studies confirm that the NO/cGMP signaling pathway is involved in the learning-memory process. NO plays a crucial role in long-term potentiation and synaptic plasticity, which are essential for learning and memory activities. These evidences suggest that NO could provide a therapeutic opportunity in AD via modulation of the relevant NOS expression (23). In this regard, we observed that pre-treatment with methylene blue increased IL and decreased STL, indicating that methylene blue reduced the improving effect of MLR-1023. In contrast, sildenafil decreased IL and increased STL, enhancing the improving effect of MLR-1023. Thus, guanylate cyclase (GC) and cGMP may play a role in the improving effect of MLR-1023 on cognitive deficiency and learning-memory impairments induced by LPS in the shuttle box test.
KATP channels regulate many cellular functions by connecting metabolic function and cell membrane electrical functions. Opening KATP channels can decrease brain damage, improve learning and memory, and provide a neuroprotective role by stimulating synaptic connections (25). In this study, pretreatment with glibenclamide (a KATP channel blocker) increased the IL and decreased the STL, indicating that it weakens the improving effect of MLR-1023. In contrast, diazoxide (a KATP channel opener) decreased the IL and increased the STL, enhancing the improving effect of MLR-1023. Thus, KATP channels may play a role in the improving effect of MLR-1023 on cognitive deficiency and learning-memory impairments induced by LPS in the shuttle box test.
Studies have shown that dysfunction of PPAR-γ receptors during neurodevelopment can cause memory impairment, whereas PPAR-γ activation is a potential therapeutic approach for these problems (26). However, our results showed that pretreatment with pioglitazone (a PPARγ receptor agonist) and GW9662 (a PPARγ receptor antagonist) did not have any significant effect on the passive avoidance task in the shuttle box test. Thus, PPARγ receptors did not play a role in the improving effect of MLR-1023 on cognitive deficiency and learning-memory impairments induced by LPS. These agonists and antagonists did not significantly affect IL and STL time when used alone.
Lipopolysaccharide can cause reactive oxygen species (ROS)-mediated rat hippocampal neuroinflammation, leading to memory loss (27). In our study, LPS injection significantly decreased the spontaneous alternation percentage compared to the control group in the Y-maze test, indicating learning-memory impairment. Treatment with MLR-1023 increased the spontaneous alternation percentage, showing the improving effect of MLR-1023 on learning-memory impairments induced by LPS. There was no difference in total arm entries between groups, indicating that the mice did not have motion impairment.
Several studies reveal that activation of neuronal nitric oxide synthase leads to increased NO levels, which activate guanylate cyclase, in turn increasing cGMP levels. A deficiency of this NO-cGMP pathway reduces the ability to learn a Y-maze task. Thus, the NO-cGMP pathway modulates the ability of rats to learn the Y-maze task, and cognitive ability in the Y-maze test may be improved by enhancing this pathway (28). Similarly, our results showed that pretreatment with L-NAME decreased the spontaneous alternation percentage compared to the LPS + MLR-1023 treated group, decreasing the improving effect of MLR-1023 on learning-memory impairments induced by LPS. In contrast, L-arginine increased the spontaneous alternation percentage, showing it enhanced the improving effect of MLR-1023 in the Y-maze test. Therefore, we can infer that the L-arginine-NO pathway may be involved in the improving effect of MLR-1023 on learning-memory impairments induced by LPS. Furthermore, pretreatment with methylene blue decreased the spontaneous alternation percentage, decreasing the improving effect of MLR-1023 on learning-memory impairments induced by LPS. On the other hand, sildenafil increased the spontaneous alternation percentage, potentiating the improving effect of MLR-1023 in the Y-maze test. Thus, GC and cGMP may be involved in the improving effect of MLR-1023 on learning-memory impairments induced by LPS. Supporting this, previous studies have proposed that extracellular cGMP modulates the ability to learn Y-maze behavior (29).
We observed that pretreatment with glibenclamide (a KATP channel blocker) decreased the spontaneous alternation percentage, thus diminishing the improving effect of MLR-1023 on learning-memory impairments induced by LPS. Similarly, some previous findings indicate that glibenclamide enhances spontaneous alternation performance, suggesting that ATP-sensitive potassium channels may play a role in cognitive performance (30). Conversely, pretreatment with diazoxide (a KATP channel opener) increased the spontaneous alternation percentage, enhancing the improving effect of MLR-1023 in the Y-maze test. Overall, it appears that KATP channels may be involved in the improving effect of MLR-1023 on learning-memory impairments induced by LPS.
It has been reported that PPARγ receptors play an important role in the Y-maze task analysis. As pioglitazone, a PPARγ agonist, can improve type 2 diabetes-related cognitive insufficiencies, it can be concluded that pioglitazone may be considered a potential agent for managing cognitive deficiencies (31). In our study, pioglitazone increased the spontaneous alternation percentage, thus enhancing the improving effect of MLR-1023 in the Y-maze test. However, pretreatment with GW9662 (a PPARγ receptor antagonist) decreased the spontaneous alternation percentage, indicating a reduction in the improving effect of MLR-1023 on learning-memory impairments induced by LPS. Therefore, PPARγ receptors may play a role in the improving effect of MLR-1023 on learning-memory impairments induced by LPS. These agonists and antagonists did not have a significant effect on the spontaneous alternation percentage when used alone.
A study has shown that antioxidant capacity, such as SOD activity, decreased in the brain of LPS-treated rats, while lipid peroxidation (MDA) and cytokine production increased (32). In our study, LPS injection significantly increased the levels of MDA, a lipid peroxidation marker, in hippocampal tissue. Treatment with MLR-1023 decreased the levels of MDA, demonstrating an improving effect on lipid peroxidation induced by LPS in hippocampal tissue.
Our study showed that pretreatment with L-NAME increased the levels of MDA, reducing the improving effect of MLR-1023 on lipid peroxidation induced by LPS. Conversely, pretreatment with L-arginine decreased the levels of MDA, enhancing the improving effect of MLR-1023 on lipid peroxidation induced by LPS. Thus, we infer that the L-arginine-NO pathway may have a possible involvement in the improving effect of MLR-1023 on lipid peroxidation induced by LPS in hippocampal tissue. Additionally, pretreatment with methylene blue increased the levels of MDA, indicating a reduction in the improving effect of MLR-1023 on LPS-induced lipid peroxidation. However, pretreatment with sildenafil did not have a significant effect on the levels of MDA. Nevertheless, GC and cGMP may have a possible role in the improving effect of MLR-1023 on LPS-induced lipid peroxidation in hippocampal tissue. One of the notable pathological changes in AD patients is lipid peroxidation. Furthermore, malondialdehyde, as an end product of peroxidation, is also increased in the brain of patients with AD (33).
Studies have shown that KATP channel blockage by glibenclamide reduces the beneficial effects of simvastatin on MDA content. Therefore, the opening of KATP channels plays a crucial role in protection against lipid peroxidation (34). In this project, pretreatment with glibenclamide increased MDA levels, diminishing the improving effect of MLR-1023 on LPS-induced lipid peroxidation. In contrast, pretreatment with diazoxide (a KATP channel opener) decreased MDA levels, enhancing the effect of MLR-1023. Thus, KATP channels may have a potential role in the positive effect of MLR-1023 on LPS-induced lipid peroxidation in hippocampal tissue.
Our results indicate that pretreatment with pioglitazone (a PPARγ receptor agonist) decreased MDA levels, enhancing the improving effect of MLR-1023 on LPS-induced lipid peroxidation. However, pretreatment with GW9662 (a PPARγ receptor antagonist) did not significantly affect MDA levels. Therefore, PPARγ receptors may have a potential role in the beneficial effect of MLR-1023 on LPS-induced lipid peroxidation in hippocampal tissue. These agonists and antagonists did not significantly affect MDA levels when used alone. However, another investigation shows that PPARγ receptors can induce the accumulation of lipid droplets, indicating the molecular regulatory mechanism of PPARγ on lipid metabolism (35).
Results from a study revealed that LPS increased MDA levels and decreased SOD activity. IL-1β knockdown in the hippocampus improved oxidative stress and neuroinflammation induced by LPS, reducing memory insufficiencies and depression-like behaviors. This suggests that IL-1β is necessary for the oxidative and neuroinflammatory responses produced by LPS (36). In our study, LPS decreased SOD enzyme activity (u/mL) in hippocampal tissue, indicating a reduction in antioxidative capacity and oxidative stress. Treatment with MLR-1023 increased SOD activity, demonstrating that MLR-1023 can improve LPS-induced impairment in SOD activity in hippocampal tissue.
Our results revealed that pretreatment with L-NAME decreased SOD activity, reducing the beneficial effect of MLR-1023 on LPS-induced impairment in SOD activity. In contrast, pretreatment with L-arginine did not significantly affect SOD activity, suggesting that the L-arginine-NO pathway may play a potential role in the improving effect of MLR-1023 on LPS-induced impairment in the antioxidative capacity of hippocampal tissue. Moreover, pretreatment with methylene blue decreased SOD activity, reducing the improving effect of MLR-1023 on LPS-induced impairment in SOD activity. However, pretreatment with sildenafil increased SOD activity, enhancing the effect of MLR-1023 on LPS-induced impairment in SOD activity. Thus, GC and cGMP may be involved in the improving effect of MLR-1023 on LPS-induced impairment in the hippocampal tissue antioxidative capacity.
Pretreatment with glibenclamide (a KATP channel blocker) decreased SOD activity, reducing the improving effect of MLR-1023 on LPS-induced impairment in SOD activity. Conversely, pretreatment with diazoxide (a KATP channel opener) did not significantly affect hippocampal tissue SOD activity, suggesting that KATP channels may play a potential role in the improving effect of MLR-1023 on LPS-induced impairment in hippocampal tissue antioxidative capacity.
Pretreatment with pioglitazone (a PPARγ receptor agonist) increased SOD activity, enhancing the effect of MLR-1023 on LPS-induced impairment in SOD activity. However, pretreatment with GW9662 (a PPARγ receptor antagonist) decreased SOD activity, reducing the improving effect of MLR-1023 on LPS-induced impairment in SOD activity. Consequently, PPARγ receptors may play a role in the beneficial effect of MLR-1023 on LPS-induced impairment in hippocampal tissue antioxidative capacity. These agonists and antagonists did not significantly affect hippocampal tissue SOD activity when used alone.
In conclusion, MLR-1023 can improve learning-memory impairments and oxidative stress induced by LPS in a mice model. The KATP/cGMP/NO cell signaling pathway and PPARγ receptors may be involved in this effect.
Acknowledgements
References
-
1.
Liu PP, Xie Y, Meng XY, Kang JS. History and progress of hypotheses and clinical trials for Alzheimer's disease. Signal Transduct Target Ther. 2019;4:29. [PubMed ID: 31637009]. [PubMed Central ID: PMC6799833]. https://doi.org/10.1038/s41392-019-0063-8.
-
2.
Biessels GJ, Despa F. Cognitive decline and dementia in diabetes mellitus: mechanisms and clinical implications. Nat Rev Endocrinol. 2018;14(10):591-604. [PubMed ID: 30022099]. [PubMed Central ID: PMC6397437]. https://doi.org/10.1038/s41574-018-0048-7.
-
3.
Zhang W, Lu J, Qing Z, Zhang X, Zhao H, Bi Y, et al. Effects of Subcortical Atrophy and Alzheimer's Pathology on Cognition in Elderly Type 2 Diabetes: The Alzheimer's Disease Neuroimaging Initiative Study. Front Aging Neurosci. 2022;14:781938. [PubMed ID: 35173604]. [PubMed Central ID: PMC8841716]. https://doi.org/10.3389/fnagi.2022.781938.
-
4.
Fang XX, Wang H, Song HL, Wang J, Zhang ZJ. Neuroinflammation Involved in Diabetes-Related Pain and Itch. Front Pharmacol. 2022;13:921612. [PubMed ID: 35795572]. [PubMed Central ID: PMC9251344]. https://doi.org/10.3389/fphar.2022.921612.
-
5.
Shi J, Yin Q, Zhang L, Wu Y, Yi P, Guo M, et al. Zi Shen Wan Fang Attenuates Neuroinflammation and Cognitive Function Via Remodeling the Gut Microbiota in Diabetes-Induced Cognitive Impairment Mice. Front Pharmacol. 2022;13:898360. [PubMed ID: 35910371]. [PubMed Central ID: PMC9335489]. https://doi.org/10.3389/fphar.2022.898360.
-
6.
Hassan HM, Elnagar MR, Abdelrazik E, Mahdi MR, Hamza E, Elattar EM, et al. Neuroprotective effect of naringin against cerebellar changes in Alzheimer's disease through modulation of autophagy, oxidative stress and tau expression: An experimental study. Front Neuroanat. 2022;16:1012422. [PubMed ID: 36312298]. [PubMed Central ID: PMC9615142]. https://doi.org/10.3389/fnana.2022.1012422.
-
7.
Yang Z, Zou Y, Wang L. Neurotransmitters in Prevention and Treatment of Alzheimer's Disease. Int J Mol Sci. 2023;24(4). [PubMed ID: 36835251]. [PubMed Central ID: PMC9966535]. https://doi.org/10.3390/ijms24043841.
-
8.
Saporito MS, Ochman AR, Lipinski CA, Handler JA, Reaume AG. MLR-1023 is a potent and selective allosteric activator of Lyn kinase in vitro that improves glucose tolerance in vivo. J Pharmacol Exp Ther. 2012;342(1):15-22. [PubMed ID: 22473614]. https://doi.org/10.1124/jpet.112.192096.
-
9.
Ochman AR, Lipinski CA, Handler JA, Reaume AG, Saporito MS. The Lyn kinase activator MLR-1023 is a novel insulin receptor potentiator that elicits a rapid-onset and durable improvement in glucose homeostasis in animal models of type 2 diabetes. J Pharmacol Exp Ther. 2012;342(1):23-32. [PubMed ID: 22431203]. https://doi.org/10.1124/jpet.112.192187.
-
10.
Gibb SL, Jeanblanc J, Barak S, Yowell QV, Yaka R, Ron D. Lyn kinase regulates mesolimbic dopamine release: implication for alcohol reward. J Neurosci. 2011;31(6):2180-7. [PubMed ID: 21307254]. [PubMed Central ID: PMC6633047]. https://doi.org/10.1523/JNEUROSCI.5540-10.2011.
-
11.
Dolatshahi M, Davoudi S, Paridar Y, Naserzadeh R, Ghorbanzadeh B. Pharmacological evidence for the involvement of the opioid system in the antidepressant-like effect of simvastatin in mice: Without tolerance and withdrawal syndrome. Neurosci Lett. 2020;714:134578. [PubMed ID: 31669314]. https://doi.org/10.1016/j.neulet.2019.134578.
-
12.
Ghorbanzadeh B, Behmanesh MA, Mahmoudinejad R, Zamaniyan M, Ekhtiar S, Paridar Y. The effect of montelukast, a leukotriene receptor antagonist, on the acetic acid-induced model of colitis in rats: Involvement of NO-cGMP-K(ATP) channels pathway. Front Pharmacol. 2022;13:1011141. [PubMed ID: 36225573]. [PubMed Central ID: PMC9549743]. https://doi.org/10.3389/fphar.2022.1011141.
-
13.
Dolatshahi M, Farbood Y, Sarkaki A, Mansouri SMT, Khodadadi A. Ellagic acid improves hyperalgesia and cognitive deficiency in 6-hydroxidopamine induced rat model of Parkinson's disease. Iran J Basic Med Sci. 2015;18(1):38-46. [PubMed ID: 25810874]. [PubMed Central ID: PMC4366741].
-
14.
Shi SH, Zhao X, Liu AJ, Liu B, Li H, Wu B, et al. Protective effect of n-butanol extract from Alpinia oxyphylla on learning and memory impairments. Physiol Behav. 2015;139:13-20. [PubMed ID: 25446210]. https://doi.org/10.1016/j.physbeh.2014.11.016.
-
15.
Wang Y, Wang M, Fan K, Li T, Yan T, Wu B, et al. Protective effects of Alpinae Oxyphyllae Fructus extracts on lipopolysaccharide-induced animal model of Alzheimer's disease. J Ethnopharmacol. 2018;217:98-106. [PubMed ID: 29447949]. https://doi.org/10.1016/j.jep.2018.02.015.
-
16.
Farbood Y, Sarkaki A, Dolatshahi M, Mansouri SMT, Khodadadi A. Ellagic Acid Protects the Brain Against 6-Hydroxydopamine Induced Neuroinflammation in a Rat Model of Parkinson's Disease. Basic Clin Neurosci. 2015;6(2):83-9. [PubMed ID: 27307952]. [PubMed Central ID: PMC4636882].
-
17.
Chen F, Ghosh A, Wu F, Tang S, Hu M, Sun H, et al. Preventive effect of genetic knockdown and pharmacological blockade of CysLT(1)R on lipopolysaccharide (LPS)-induced memory deficit and neurotoxicity in vivo. Brain Behav Immun. 2017;60:255-69. [PubMed ID: 27810377]. https://doi.org/10.1016/j.bbi.2016.10.021.
-
18.
Shahsavarian A, Javadi S, Jahanabadi S, Khoshnoodi M, Shamsaee J, Shafaroodi H, et al. Antidepressant-like effect of atorvastatin in the forced swimming test in mice: the role of PPAR-gamma receptor and nitric oxide pathway. Eur J Pharmacol. 2014;745:52-8. [PubMed ID: 25446923]. https://doi.org/10.1016/j.ejphar.2014.10.004.
-
19.
Ludka FK, Zomkowski AD, Cunha MP, Dal-Cim T, Zeni AL, Rodrigues AL, et al. Acute atorvastatin treatment exerts antidepressant-like effect in mice via the L-arginine-nitric oxide-cyclic guanosine monophosphate pathway and increases BDNF levels. Eur Neuropsychopharmacol. 2013;23(5):400-12. [PubMed ID: 22682406]. https://doi.org/10.1016/j.euroneuro.2012.05.005.
-
20.
Ostadhadi S, Akbarian R, Norouzi-Javidan A, Nikoui V, Zolfaghari S, Chamanara M, et al. Possible involvement of ATP-sensitive potassium channels in the antidepressant-like effects of gabapentin in mouse forced swimming test. Can J Physiol Pharmacol. 2017;95(7):795-802. [PubMed ID: 28177665]. https://doi.org/10.1139/cjpp-2016-0292.
-
21.
Budni J, Gadotti VM, Kaster MP, Santos AR, Rodrigues AL. Role of different types of potassium channels in the antidepressant-like effect of agmatine in the mouse forced swimming test. Eur J Pharmacol. 2007;575(1-3):87-93. [PubMed ID: 17761162]. https://doi.org/10.1016/j.ejphar.2007.08.010.
-
22.
Schirmbeck GH, Seady M, Froes FT, Taday J, Da Re C, Souza JM, et al. Long-term LPS systemic administration leads to memory impairment and disturbance in astrocytic homeostasis. Neurotoxicology. 2023;99:322-31. [PubMed ID: 38006911]. https://doi.org/10.1016/j.neuro.2023.11.009.
-
23.
Dubey H, Gulati K, Ray A. Alzheimer's Disease: A Contextual Link with Nitric Oxide Synthase. Curr Mol Med. 2020;20(7):505-15. [PubMed ID: 31782366]. https://doi.org/10.2174/1566524019666191129103117.
-
24.
Orzelska-Gorka J, Bernat P, Tutka P, Listos J, Kedzierska E, Fidecka S, et al. Modification of NO-cGMP Pathway Differentially Affects Diazepam- and Flunitrazepam-Induced Spatial and Recognition Memory Impairments in Rodents. Neurotox Res. 2020;37(4):1036-46. [PubMed ID: 31792805]. [PubMed Central ID: PMC7085477]. https://doi.org/10.1007/s12640-019-00110-1.
-
25.
Yahil S, Wozniak DF, Yan Z, Mennerick S, Remedi MS. Cognitive deficits and impaired hippocampal long-term potentiation in K(ATP)-induced DEND syndrome. Proc Natl Acad Sci U S A. 2021;118(45). [PubMed ID: 34732576]. [PubMed Central ID: PMC8609313]. https://doi.org/10.1073/pnas.2109721118.
-
26.
Garcia-Baos A, Pastor A, Gallego-Landin I, de la Torre R, Sanz F, Valverde O. The role of PPAR-gamma in memory deficits induced by prenatal and lactation alcohol exposure in mice. Mol Psychiatry. 2023;28(8):3373-83. [PubMed ID: 37491462]. https://doi.org/10.1038/s41380-023-02191-z.
-
27.
Khan MS, Muhammad T, Ikram M, Kim MO. Dietary Supplementation of the Antioxidant Curcumin Halts Systemic LPS-Induced Neuroinflammation-Associated Neurodegeneration and Memory/Synaptic Impairment via the JNK/NF-kappaB/Akt Signaling Pathway in Adult Rats. Oxid Med Cell Longev. 2019;2019:7860650. [PubMed ID: 31827700]. [PubMed Central ID: PMC6885271]. https://doi.org/10.1155/2019/7860650.
-
28.
Llansola M, Hernandez-Viadel M, Erceg S, Montoliu C, Felipo V. Increasing the function of the glutamate-nitric oxide-cyclic guanosine monophosphate pathway increases the ability to learn a Y-maze task. J Neurosci Res. 2009;87(10):2351-5. [PubMed ID: 19326454]. https://doi.org/10.1002/jnr.22064.
-
29.
Cabrera-Pastor A, Malaguarnera M, Taoro-Gonzalez L, Llansola M, Felipo V. Extracellular cGMP Modulates Learning Biphasically by Modulating Glycine Receptors, CaMKII and Glutamate-Nitric Oxide-cGMP Pathway. Sci Rep. 2016;6:33124. [PubMed ID: 27634333]. [PubMed Central ID: PMC5025658]. https://doi.org/10.1038/srep33124.
-
30.
Stefani MR, Nicholson GM, Gold PE. ATP-sensitive potassium channel blockade enhances spontaneous alternation performance in the rat: a potential mechanism for glucose-mediated memory enhancement. Neuroscience. 1999;93(2):557-63. [PubMed ID: 10465439]. https://doi.org/10.1016/s0306-4522(99)00128-1.
-
31.
Jiang LY, Tang SS, Wang XY, Liu LP, Long Y, Hu M, et al. PPARgamma agonist pioglitazone reverses memory impairment and biochemical changes in a mouse model of type 2 diabetes mellitus. CNS Neurosci Ther. 2012;18(8):659-66. [PubMed ID: 22620268]. [PubMed Central ID: PMC6493499]. https://doi.org/10.1111/j.1755-5949.2012.00341.x.
-
32.
Heidarzadeh M, Amininasab M, Rezayat SM, Mousavi SE. Investigation of Antioxidant and Anti-inflammatory Properties of Berberine Nanomicelles: In vitro and In vivo Studies. Curr Drug Deliv. 2024;21(9):1273-83. [PubMed ID: 37815182]. https://doi.org/10.2174/0115672018258030230920035222.
-
33.
Christen Y. Oxidative stress and Alzheimer disease. Am J Clin Nutr. 2000;71(2):621S-9S. [PubMed ID: 10681270]. https://doi.org/10.1093/ajcn/71.2.621s.
-
34.
Dowlatshahi K, Ajami M, Pazoki-Toroudi H, Hajimiresmaiel SJ. ATP-dependent potassium channels are implicated in simvastatin pretreatment-induced inhibition of apoptotic cell death after renal ischemia/reperfusion injury. Med J Islam Repub Iran. 2015;29:191. [PubMed ID: 26157709]. [PubMed Central ID: PMC4476217].
-
35.
Hao K, Wang J, Yu H, Chen L, Zeng W, Wang Z, et al. Peroxisome Proliferator-Activated Receptor gamma Regulates Lipid Metabolism in Sheep Trophoblast Cells through mTOR Pathway-Mediated Autophagy. PPAR Res. 2023;2023:6422804. [PubMed ID: 38020065]. [PubMed Central ID: PMC10651342]. https://doi.org/10.1155/2023/6422804.
-
36.
Li M, Li C, Yu H, Cai X, Shen X, Sun X, et al. Lentivirus-mediated interleukin-1beta (IL-1beta) knock-down in the hippocampus alleviates lipopolysaccharide (LPS)-induced memory deficits and anxiety- and depression-like behaviors in mice. J Neuroinflammation. 2017;14(1):190. [PubMed ID: 28931410]. [PubMed Central ID: PMC5607621]. https://doi.org/10.1186/s12974-017-0964-9.