Abstract
Background:
Cumulative dose of ovary as a radiosensitive organ during abdominal and pelvic CT scan imaging is still a controversial challenge that requires practical dose reduction strategies. Although bismuth shields can reduce the dose in the right proportions, their use is controversial due to the reduced image quality.Objectives:
The aim of this study was to investigate the performance of a new combination of X-ray absorber structures that have less effect on image quality parameters.Methods:
First, various shields with different weight percentages of Cu and Bi were made, then the percentage of dose reduction and image quality were evaluated via phantoms. Finally, Shield with the least effect on image quality was evaluated for clinical evaluation on 20 patients.Results:
Shielding with thicknesses of 1T and 3T reduced the Entrance skin dose of ovarian by about 52% and 73%, respectively. Shields with 90% cu-10% Bi and 100% Bi structures had the least and most destructive effects on image quality, respectively, and also have the same image quality. The 10% Bi-90% Cu shield provided a 21% greater dose reduction than the bismuth shield. Also, this 1T thick shield did not create an artifact in the reconstructed images.Conclusions:
Shields are flexible, inexpensive, and user-friendly for ovarian shielding in abdominal and pelvic CT scans. Unlike bismuth shields, shields do not have the detrimental effects of image quality degradation.Keywords
1. Background
The use of computed tomography (CT) as a diagnostic imaging technology has increased dramatically over the past two decades. CT scans have a higher radiation dose than other diagnostic methods, as this method is responsible for more than 70% of the total doses of x-ray diagnostic procedures (1, 2).
Multi-detector computed tomography (MCDT) is an advanced technology that is faster than older generations of CT scanners. However, more doses are given to the patient (3) due to the unique diagnostic role of CT scan in a huge range of abdominal and pelvic diseases. This method is considered to be the most accurate technique for abdominal and pelvic exams after ultrasound (4). We should bear in mind that the radiation dose on the patient's body surface is maximal, and it decreases as it passes through the patient's body (5). The dose of the ovary in a normal CT scan Abdominal and pelvis were estimated in the range of 24.27 to 91.16 mGy via impact software with a standard deviation of 68.87 ± 16.81 (4).
Using a bismuth shield in CT imaging is an effective way to reduce the absorbed dose of ovaries (6, 7). Bismuth shield reduces the surface dose of the organ by absorbing low energy photons (8). Many studies have shown dose reduction efficiency of bismuth shields to be 26% to 57% (5, 6). In another study by Sancaktutar et al. (9), a dose reduction of approximately 90.2% was obtained using a bismuth shield for abdominal and pelvic CT scans. A dose of 50.5% was obtained with bismuth shields (6).
On the other hand, despite the advantages of bismuth shields, some studies have shown the destructive properties of these shields on image quality parameters such as SNR and CT number, as well as creating artifacts (7, 9-12).
2. Objectives
In this study, we designed and built shields that are perfectly suited for protecting the ovaries during CT scans of the abdomen and pelvis and do not have the disadvantages of common bismuth shield like reducing images quality and CT number shift that could disrupt the disease diagnose. Were clinically evaluated. Materials and methods, and then the results will be described below.
3. Methods
We used a tissue equivalent tissue phantom to measure the cumulative dose of ovarian position. This measurement was performed using thermoluminescence dosimeters. A CTDI acrylic phantom was used to measure noise and CT number changes. The effect of distance from the shield on image quality was investigated via the CTDI phantom to find the ideal distance. Image quality was also assessed using an anthropomorphic female phantom.
To evaluate the quality of the images, 20 female patients in the age range of 20 - 75 were divided into control and intervention groups. In the radiology department, after completing the consent forms, hardened shields were placed on the body of the ovary of women in the intervention group. A non-contrasting holder was made in which the shields were fixed, and under each shield was a 3 cm layer of noise-reducing foam between the patient's body and the shield. Routine abdominal and pelvic CT scans were performed in the control group of patients without any additional procedures.
All studies were performed using a Philips Brilliance 16-slice CT scanner that was effective in axial position and 120 kvp tube voltage and 162 mAs tube current and slice thickness of 0.5 cm.
3.1. Shield Construction, Image Quality Evaluation, and Statistical Analysis
To reduce the patient dose without significantly reducing the SNR value, the photon energy must be intentionally modified so that low-energy beams can be removed from the spectrum, and high-energy beams can be maintained. To this end, physical protection may be a good solution. However, protective ingredients will play an important role in the success of this method. We found that Cu had all the properties and was selected as the base metal in the shield structure. Bismuth is a metal used as a shield in the past, while it cannot Modify the photon energy in a targeted way So that low energy photons remove from the spectrum and maintain high energy photons, which leads to image formation. Therefore, not a good choice. To test the accuracy and efficiency of this theory, different ovarian shields were made with different weight percentages of Cu and Bi (Table 1).
Different Combinations and Widths of the Constructed Shields
Name | Bi (%W) | Cu (%W) | Width |
---|---|---|---|
10% Bi-90% Cu | 10 | 90 | 1T-3T |
50% Bi-90% Cu | 50 | 50 | 1T-3T |
90% Bi-10% Cu | 90 | 10 | 1T-3T |
100% Bi | 100 | 0 | 1T-3T |
A mold made by 3D printing technology with a circular shape was used to shape the shields. RTV (room-temperature-vulcanizing silicone) was mixed well as an elastomeric intermediate and Cu and Bi powders using a mechanical stirrer. The shields were made in a circular shape with a diameter of 120 mm and a constant thickness of 5 mm except at marginal 5 mm.
The thickness edges decreases slightly to Avoid artifact due to the sharp edge of shields. For the preliminary evaluation of the new compounds, the effect of these shields on noise change and the CT number was investigated using CTDI phantom.
Quantitative image quality analyses performed via anthropomorphic phantoms by measuring noise and CT numbers shift at different ROIs. Different ROIs were evaluated. Image noise was measured using standard attenuation of attenuation values (per Hounsfield unit) in a homogeneous region. It was considered (Figure 1) that the CT number and standard deviation within each ROI were measured for ten consecutive sequences of exile images and then averaged to obtain an accurate value.
Selected different ROI, s in pelvis axial CT scan for noise and CT number shift calculation
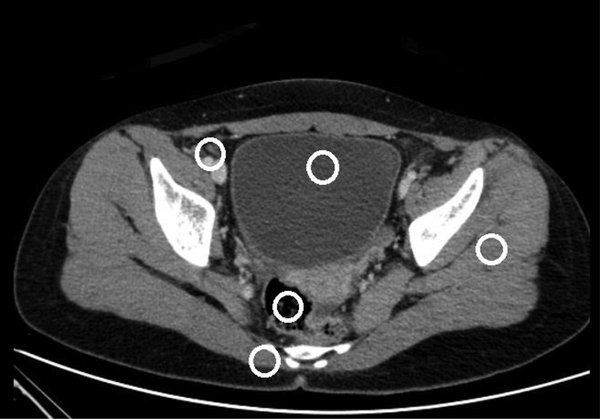
Differences between the groups were statistically determined to be less than 0.05 using paired student t-test and statistical significance. Kolmogorov-Smirnov test was performed to check the normality of the data. In the field of clinical trial, statistical differences between the two groups for image quality were assessed using the non-parametric Mann-Whitney test.
4. Results
4.1. Surface Dose Using Anthropomorphic Phantom
Table 2 shows the mean value and standard deviation of the skin input dose (ESD) measured for different shields with thicknesses of 1T and 3T. The mean of ovarian ESD during abdominal and pelvic CT scan was 22.02 ± 1.12 for non-shielded condition and for 1T thickness, the amount of ESD reduction for 10% Bi-90% Cu, 50% Bi-50% Cu, 90% Bi-10% Cu, and 100% Bi shields were shown to be 52.1%, 54.1%, 50.8%, and 52.8%, respectively.
Mean Entrance Skin Dose (ESD) of the Ovaries During Pelvis CT Scans with and Without Manufactured Shields with the Thickness of 1 T and 3 T
Shield Composition | ESD, mGy | Dose Reduction, % | |
---|---|---|---|
1T | No shielding | 22.2 ± 1.12 | - |
10% Bi-90% Cu | 10.55 ± 0.91 | 52.1 | |
50% Bi-50% Cu | 10.06 ± 0.77 | 54.1 | |
90% Bi-10% Cu | 10.82 ± 0.74 | 50.8 | |
100% Bi | 10.38 ± 0.98 | 52.8 | |
3T | 10% Bi-90% Cu | 5.8 ± 1.1 | 73.6 |
50% Bi-50% Cu | 5.85 ± 0.63 | 73.4 | |
90% Bi-10% Cu | 5.92 ± 0.87 | 73.1 | |
100% Bi | 6.14 ± 0.85 | 72.1 |
Also, for 3T thickness, ESD reduction rate for 10% Bi-90% Cu, 50% Bi-50% Cu, 90% Bi-10% Cu and 100% Bi shields equal to 73.6%, 73.4%, 73.1% and 72.1%, respectively were shown.
For all manufactured shields, the dose reduction rate was statistically significant (P < 0.05). By increasing the thickness of the shields from 1T to 3T, a greater surface dose reduction rate (about 20%) was obtained.
4.2. Image Quality Analysis Using CTDI Phantom and Anthropomorphic Phantom
The shields were placed on a uniform phantom to evaluate the quality of the image during the CT scan. Some ROIs were considered at the center of the phantom at different distances from the shield. The amount of CT number and noise were measured for each ROI in 10 consecutive slices, then the change in CT number and increase in noise were calculated comparing to the non-shielded mode (dose reference mode). Increasing the weight percentage of Cu in the structure of the shields reduces the noise and the amount of CT number shift in the images. 10% Bi-90% Cu Shield has the least effect on image quality in terms of noise and CT number shift, which decreases rapidly with increasing distance between shield and ROI.
4.3. CT Number Shift and Noise Increasing
The mean CT number and image noise on the anterior pelvic region were 11.9 and 6.8 HU, respectively. The use of 10% Bi-90% Cu, 50% Bi-50% Cu, 90% Bi-10% Cu, and 100% Bi shields with 1T thickness, Anterior pelvic image noise 0.8 (11%), 1.2, respectively (Increased by 17%), 9.1 (27%) and 7.2 (40%). Mean CT of anterior pelvic CT for 1T thickness of 10% Bi-90% Cu, 50% Bi-50% Cu, 90% Bi-10% Cu, and 100% Bi shields increased 5.8, 14.4, 17.7, and 19.2 HU, respectively.
All composed shields except (10% Bi-90% Cu) “ideal shield” affected significantly in noise quantity and CT number shifting amount of all studied ROIs. The effects of the ideal shield with a thickness of 1T had no statistically significant effect on bladder noise (P = 0.08) and rectal (P = 0.07). Also, this shield did not significantly change the CT number of the gluteus maximus (P = 0.53), iliac vessels (0.06) and rectal (P = 0.53). The effects of 3T thickness ideal shield had no significant effect on rectal noise (P = 0.06) and iliac artery CT number (P = 0.13). In addition, the effect of the ideal 3T thickness shield on image quality was similar to that of the 1T bismuth shield. Thus, the ideal 3T shield produced 21% more dose reduction properties than the 1T bismuth shield in the same image quality.
The results showed that the ideal shield with a structure of 10% Bi-90% Cu shows the least effect on image quality for CT number shift and noise.
5. Discussion
The previous studies employed two techniques to calculate dose reduction: (ORGAN DOSE MEASURMENT or entrance skin dose (ESD) measurements), which in the present study, the second method was used. According to Dose measurements the use of shields made for ovaries with a thickness of 1T can significantly reduce the amount of ESD (50.8% - 54.1%). The dose reduction properties of 1T thick shields were approximately equal to the expected amount. The results of our dose reduction were consistent with the results of Hopper et al. (6), Alonso et al. (3), and Einstein et al. (11) studies, and the only difference with the mentioned studies could be related to imaging techniques. Performance of 1T constructed shields was better than study shields of Hohl et al. (13), Lambert and Gould (14); Yilmaz et al. (15), Gatuzzo (16), and Wang et al. (17).
Since the manufactured shields were effective, they can be used in clinical treatments. The efficiency of these shields increased significantly with increasing thickness from 1T to 3T.
5.1. Effect on Noise and CT Number Shift: An Anthropomorphic Phantom Study
We used the CTDI phantom for the initial evaluation of the shields. The results showed that with increasing the percentage of Cu, image noise and CT number shifting significantly decreased. Increasing the amount of Cu in shields from 0% to 90% leads to a 105% reduction in image noise dose and 21% in changing the CT number at a distance of 3 cm from the shield.
The results showed that the shield with a combination of 10% Bi-90% Cu will have the least detrimental effect on image quality. Both the made-up shield and the bismuth shield had a significant CT noise in direct contact with the phantom surface. However, as the distance between the shields and the phantom level increased, the noise and CT number increased rapidly. For the bismuth shield, the noise was reduced from 2296% to 85% by creating a distance of 5 cm relative to the direct contact with the phantom surface. Also, the CT number shifted from 161 to 33 HU.
By moving the ideal shields from contact to a distance of 5 cm from its surface via the phantom surface, the noise was reduced from 522% to 25%, and the CT number change from 59 to 16 HU. These shields produced no noise and only resulted in a 6 HU change in CT numbers at a distance of 9 cm.
5.2. The Effect on Noise and CT Number: A “Phantom and Patient” Study
All shields were evaluated using a human-like phantom. The shields were placed on the ovarian region at a distance of 3 cm. In the ROI of the gluteus intermedius region, the noise and CT number changes were 11% and 5.8 HU for the ideal shield, respectively, and 40% and 19.2 HU for the bismuth shield, respectively. The ideal shield noise levels in the ROI of the bladder, rectum, and iliac arteries were 2%, 0.7%, and 9%, respectively. The ideal shield did not change the Cat number of the iliac artery and only resulted in a change of 2 HU in the CT number in the bladder area and 1 HU in the rectal area. The results of the study on the anthropomorphic phantom, like the results of the CTDI phantom study, proved that the ideal 1T-thick shield had no detrimental effect on image quality. Figure 2 shows a noise comparison diagram of the ideal shields and the bismuth shield at different ROIs.
Comparison of 10% Bi-90% Cu and 100% Bi shields on Images noise for different ROI
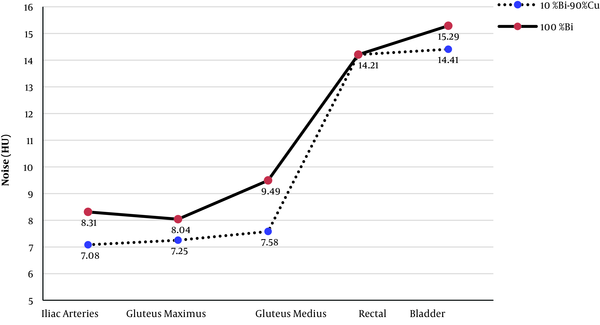
The effects of 1T thickness ideal shield on image quality were not significant in many areas. The ideal 3T thickness shield had the same effects as the 1T shield. However, the dose reduction in 3T shields was 21% higher than in 1T shield. Therefore, by using the ideal 3T shield instead of the conventional 1T bismuth shield, a dose reduction of 21% can be achieved with the same amount of image quality. The ideal 1T shield can also be used for better image quality. Figure 3 shows the effect of the two ideal and bismuth shields on the CT number of the ROI of the gluteus maximus and medius.
Comparison of 10% Bi-90% Cu and 100% Bi on CT number shift for gluteus maximus and gluteus intermedius regions.
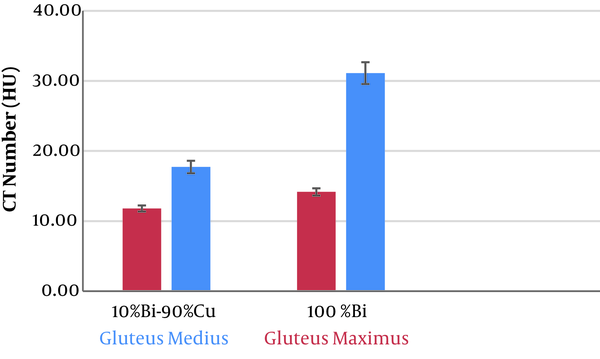
In the patient study, based on the radiologist report in Figure 4, the Ideal 1T shield did not have a detrimental effect on image quality, and the results of the clinical trial were consistent with studies on anthropomorphic phantoms.
examples of pelvic CT images without and with 1T shields thicknesses. A, 100% Bi; B, 90% Bi-10% Cu; C, 50% Bi-50% Cu; D, 10% Bi-90% Cu; E, without shielding.
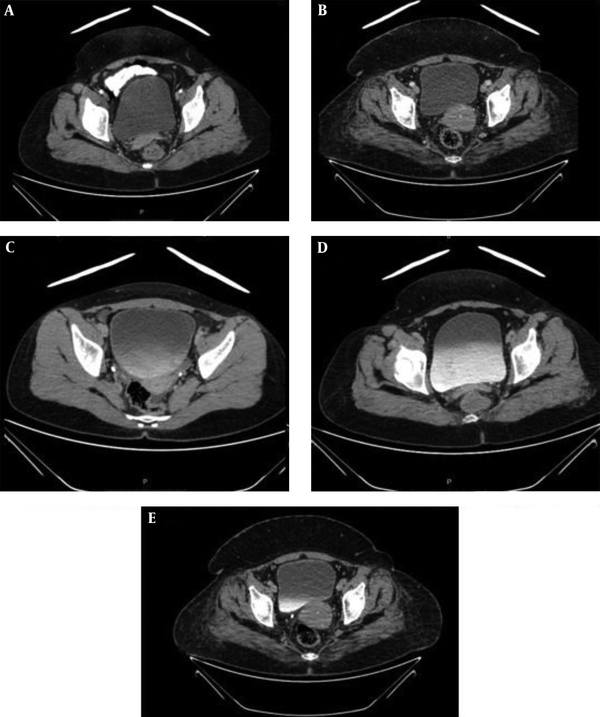
5.3. Patient Radiation Exposure Wasting
As we know, radiation wasting, which means removing high energy photons from the radiation spectra, leads to a decrease in SNR, and a decrease in SNR has a linear relationship with an increase in noise. Therefore, a slight increase in image noise means that the amount of radiation wasting is not noticeable.
The results of this study showed that the amount of ideal shield noise is much less than the bismuth shield. Also, the amount of ideal shield noise in most ROI was not statistically significant (P value < 0.05). It can be concluded that ideal shields have solved the problem of radiation loss.
5.4. Conclusions
The present study aimed to design and build a new shield to reduce the dose received by the ovaries in abdominal and pelvic CT scans without adversely affecting image quality. For this purpose, several shields were hardened using different combinations of Cu and Bi, and their performance was compared with conventional bismuth shields. The results of studies on the uniform phantom of CTDI and anthropomorphic phantom showed that the shield with 10% Bi-90% Cu structure had the least effect on image quality. A new 1T-thick shield reduced the entry dose of ovarian skin by 50%, similar to the bismuth 1T shield, while the effects of the ideal shield on image noise and CT change were calculated a lot less than the bismuth shield. Also, the ideal shield reduced the radiation dose by 21% more than the bismuth shield with the same thickness of 1T in the same image quality.
In the anthropomorphic phantom study, the largest change in CT was observed for the ideal shield less than 6 HU.
Also, the maximum noise generated from the reference dose mode was less than 11%. According to the radiologist, the results of the clinical trial were consistent with the results of phantom studies. On the other hand, no artifacts were observed in the reconstructed images for the Ideal 1T Shield.
References
-
1.
Smith-Bindman R, Miglioretti DL, Johnson E, Lee C, Feigelson HS, Flynn M, et al. Use of diagnostic imaging studies and associated radiation exposure for patients enrolled in large integrated health care systems, 1996-2010. JAMA. 2012;307(22):2400-9. [PubMed ID: 22692172]. [PubMed Central ID: PMC3859870]. https://doi.org/10.1001/jama.2012.5960.
-
2.
Pola A, Corbella D, Righini A, Torresin A, Colombo PE, Vismara L, et al. Computed tomography use in a large Italian region: trend analysis 2004-2014 of emergency and outpatient CT examinations in children and adults. Eur Radiol. 2018;28(6):2308-18. [PubMed ID: 29318431]. https://doi.org/10.1007/s00330-017-5225-x.
-
3.
Alonso TC, Mourao AP, Santana PC, da Silva TA. Assessment of breast absorbed doses during thoracic computed tomography scan to evaluate the effectiveness of bismuth shielding. Appl Radiat Isot. 2016;117:55-7. [PubMed ID: 27033028]. https://doi.org/10.1016/j.apradiso.2016.03.018.
-
4.
Amirnia A, Mehnati P, Jabbari N. Estimation of absorbed dose of radiosensitive organs and effective sose in patients underwent abdominopelvic spiral CT scan using impact CT patient dosimetry. Tehran Univ Med J. 2017;75(2):103-12.
-
5.
Hopper KD, Neuman JD, King SH, Kunselman AR. Radioprotection to the eye during CT scanning. AJNR Am J Neuroradiol. 2001;22(6):1194-8. [PubMed ID: 11415918].
-
6.
Hopper KD, King SH, Lobell ME, TenHave TR, Weaver JS. The breast: in-plane x-ray protection during diagnostic thoracic CT--shielding with bismuth radioprotective garments. Radiology. 1997;205(3):853-8. [PubMed ID: 9393547]. https://doi.org/10.1148/radiology.205.3.9393547.
-
7.
Lawrence S, Seeram E. The Current Use and Effectiveness of Bismuth Shielding in Computed Tomography: A Systematic Review. Radiol Open J. 2017;2(1):7-16. https://doi.org/10.17140/roj-2-113.
-
8.
Akhlaghi P, Miri-Hakimabad H, Rafat-Motavalli L. Effects of shielding the radiosensitive superficial organs of ORNL pediatric phantoms on dose reduction in computed tomography. J Med Phys. 2014;39(4):238-46. [PubMed ID: 25525312]. [PubMed Central ID: PMC4258732]. https://doi.org/10.4103/0971-6203.144490.
-
9.
Sancaktutar AA, Bozkurt Y, Onder H, Soylemez H, Atar M, Penbegul N, et al. A new practical model of testes shield: the effectiveness during abdominopelvic computed tomography. J Androl. 2012;33(5):984-9. [PubMed ID: 22207708]. https://doi.org/10.2164/jandrol.111.015560.
-
10.
Mathieu KB, Cody DD. Partial arc beam filtration: a novel approach to reducing CT breast radiation dose. AJR Am J Roentgenol. 2013;200(3):601-7. [PubMed ID: 23436850]. [PubMed Central ID: PMC3855393]. https://doi.org/10.2214/AJR.12.8771.
-
11.
Einstein AJ, Elliston CD, Groves DW, Cheng B, Wolff SD, Pearson GD, et al. Effect of bismuth breast shielding on radiation dose and image quality in coronary CT angiography. J Nucl Cardiol. 2012;19(1):100-8. [PubMed ID: 22068687]. [PubMed Central ID: PMC3266996]. https://doi.org/10.1007/s12350-011-9473-x.
-
12.
Revel MP, Fitton I, Audureau E, Benzakoun J, Lederlin M, Chabi ML, et al. Breast dose reduction options during thoracic CT: influence of breast thickness. AJR Am J Roentgenol. 2015;204(4):W421-8. [PubMed ID: 25794091]. https://doi.org/10.2214/AJR.14.13255.
-
13.
Hohl C, Wildberger JE, Suss C, Thomas C, Muhlenbruch G, Schmidt T, et al. Radiation dose reduction to breast and thyroid during MDCT: effectiveness of an in-plane bismuth shield. Acta Radiol. 2006;47(6):562-7. [PubMed ID: 16875333]. https://doi.org/10.1080/02841850600702150.
-
14.
Lambert JW, Gould RG. Evaluation of a Net Dose-Reducing Organ-Based Tube Current Modulation Technique: Comparison With Standard Dose and Bismuth-Shielded Acquisitions. AJR Am J Roentgenol. 2016;206(6):1233-40. [PubMed ID: 27058461]. https://doi.org/10.2214/AJR.15.15778.
-
15.
Yilmaz MH, Albayram S, Yasar D, Ozer H, Adaletli I, Selcuk D, et al. Female breast radiation exposure during thorax multidetector computed tomography and the effectiveness of bismuth breast shield to reduce breast radiation dose. J Comput Assist Tomogr. 2007;31(1):138-42. [PubMed ID: 17259846]. https://doi.org/10.1097/01.rct.0000235070.50055.e6.
-
16.
Catuzzo P, Aimonetto S, Fanelli G, Marchisio P, Meloni T, Mistretta L, et al. Dose reduction in multislice CT by means of bismuth shields: results of in vivo measurements and computed evaluation. Radiol Med. 2010;115(1):152-69. [PubMed ID: 20012921]. https://doi.org/10.1007/s11547-009-0469-4.
-
17.
Wang J, Duan X, Christner JA, Leng S, Yu L, McCollough CH. Radiation dose reduction to the breast in thoracic CT: comparison of bismuth shielding, organ-based tube current modulation, and use of a globally decreased tube current. Med Phys. 2011;38(11):6084-92. [PubMed ID: 22047373]. https://doi.org/10.1118/1.3651489.