Abstract
Background:
A major problem in the development of required chemical compounds in a variety of industries such as pharmaceutical, agricultural, paint and polymer industries is to easily access the chemical starting materials. One class of these materials is quinoline derivatives. Synthesis of these compounds, despite their simple chemical structures is relatively complicated and their synthesis design and purification are challenging. Quinoline derivatives were synthesized and explored for their anticancer, antitinephritic, antitumor, anti-inflammatory and analgesic activity, as antiallergetic agents for treating Alzheimer’s disease (AD). Possible formation of 5- and 7-substituted quinolines during the Skraup reaction using m-substituted anilines is well understood. However, there are conflicting reports on the composition of the products from certain reactions.Objectives:
As part of our research, we are interested in the development of synthetic strategies for preparation of 7-methyl-8-nitroquinoline selectively from a prepared mixture of 5- and 7-methyl quinoline as a key material to study its biological activities as well as to develop other corresponding quinoline derivatives for our research purpose.Materials and Methods:
Synthesis and characterization of 7-methylquinoline using the Skraup methodology was reported. A subsequent nitration reaction was also carried out using a mixture of 5- and 7-methylquinoline to obtain pure 7-methyl-8-nitroquinoline selectively. In this project, in the first step, a mixture of 7-methylquinolin and 5-methylquinolin was prepared from the reaction of m-toluidine and glycerol. In the next step, 7-methyl-8-nitroquinoline was selectively synthesized and subsequently characterized using conventional methods such as NMR, IR, GC-MS and elemental analysis.Results:
The compound 7-methyl-8-nitroquinolin was selectively synthesized in a two-step reaction with an excellent yield.Conclusions:
7-methyl-8-nitroquinolin as a key starting material was obtained through a two-step synthesis from m-toluidine. Skraup synthesis was employed to produce a mixture of 7- and 5-methylquinoline in a 2:1 ratio, which was then used for a nitration reaction using nitric acid and sulfuric acid without further purification. The final reaction selectively produced 7-methyl-8-nitroquinolin with an excellent yield.Keywords
Synthesis Key Starting Material Characterization Nitroquinoline
1. Background
Numerous recent reports have proved that quinoline ring containing compounds exhibit potent biological activities. Quinolines and their derivatives have been found to be important constituents of several biological active synthetic compounds (1-3) such as DNA-intercalating carriers (4) and those with DNA binding capability (5) and antitumor effects (6, 7). Quinoline derivatives were synthesized and explored for their analgesic activity (8) as antiallergetic agents (9) for treating Alzheimer’s disease (AD) (10), anticancer (11, 12), antitinephritic (13), antitumor (14), anti-inflammatory and other biological activities (15-17). Several quinoline derivatives were identified as potent inhibitors of the Pim-1 kinase which is involved in the control of cell growth, differentiation, proliferation and apoptosis (12).
Quinoline-containing antimalarial drugs, such as chloroquine, quinine and mefloquine, are mainstays of chemotherapy against malaria (18). Due to its unique pharmaceutical importance, a continuous development in the synthesis of new quinoline derivatives is a growing area of research.
Quinoline and its derivatives are well known for their antimalarial and antibacterial properties. A number of quinoline derivatives are known to possess antimicrobial, antitumor, antifungal, hypotensive, anti HIV, analgesics and anti-inflammatory activities.
Great attention is currently paid to the synthesis and study of the biological properties of quinoline derivatives by synthetic and medicinal chemists. Several classical methods for targeting the quinoline core, including the Skraup, Doebner-von Miller, Friedländer, Pfitzinger, Conrad-Limpach, and Combes syntheses (19, 20), have been developed for the preparation of quinolone-containing materials, ligands, and pharmaceutical agents (18). Most of these methods involve reactions which are often performed under unfavorably harsh conditions, mostly reactions under strong acidic or basic conditions or with high temperatures (17-22). Many quinoline derivatives are important starting materials for a wide range of applications such as drugs and industries (23-25).
A great deal of investigation has previously shown that the possible formation of 5- and 7-substituted quinolines during the well-known Skraup reaction with m-substituted anilines involves conflicting reports about the composition of the products from certain reactions (26-30).
Durce (27) reported that 7-methylquinoline (Figure 1) was the sole product from the reaction with m-toluidine which was later confirmed by Bradford, Elliot and Rowe (28) who reported in a detailed survey that ortho-/para-directing groups, such as the methyl group, gave only 7-substituted quinolines. A simultaneous report by Poutermann and Girardet (29) however, claimed that a mixture of 5- and 7-isomers was formed. Palmer (30) using gas chromatography showed that m-toluidine gave a mixture of 7-methyl- and 5-methylquinolines containing approximately 60% of the 7-isomer.
Although 7-methyl-8-nitroquinoline, itself, has a potential for biological activities, it will also have a great potential as a starting material for the development of a wide range of other biologically active compounds. As a part of our study, we were interested in multistep reduction and oxidation reactions of this compound which yields polypyridine compounds with a more efficient potential for biological activities such as anti-inflammatory agents and DNA intercalating compounds; such research has previously been undertaken by Barton and her research group (31-42).
There have been a number of functionalization reaction studies of quinolines including nitration reactions of 7-alkylquinolines (43, 44). In all cases, the corresponding 8-nitroquinoline was identified as the main product but the nature of the minor products formed remains uncertain. Capps (44) starting from impure 1 showed that an unidentified minor product was neither 7-methyl-5-nitro nor 5-methyl-5-nitroquinoline, which implied that nitration occurred in the pyridine nucleus. Here in, we report on the synthesis and characterization of 7-methylquinoline using the Skraup methodology. A subsequent nitration reaction was also carried out using a mixture of 5- and 7-methylquinoline to obtain pure 7-methyl-8-nitroquinoline selectively.
2. Objectives
In this study, we investigated the two-step synthesis of 7-methyl-8-nitroquinoline from m-toluidine in which conventional methods such as NMR spectroscopy, GC-MS spectrometry, IR spectroscopy and elemental analysis were used to characterize the products.
3. Materials and Methods
3.1. General Experimental
Reagent-grade solvents were used for the syntheses of compounds without further purification. 1H NMR and 13C NMR spectra were recorded on Varian UNITY-300 or Varian INOVA-500 spectrometers. 1H NMR and 13C NMR chemical shifts were referenced to residual solvent resonances (CDCl3, 7.25 and 77.01 ppm, respectively). Infrared spectra (400-4000 cm-1) were obtained using a Shimadzu 8201PC Series FTIR interfaced with an Intel 486 PC operating Shimadzu HyperIR software. Spectra were obtained using the diffuse reflectance method in solid KBr. GC-MS, LC-MS and Microanalyses were performed at Shahid Beheshti University of Medical Sciences. Glycerol and m-toluidine were obtained from Aldrich and used without further purification. All other starting materials were obtained commercially and used as received.
3.2. Syntheses
For the synthesis of 7-Methyl-8-nitro-quinolin, the two-step route shown in Figure 1 was employed.
Two-step Selective Synthesis of 7-Methyl-8-Nitro-quinoline From m-toluidine
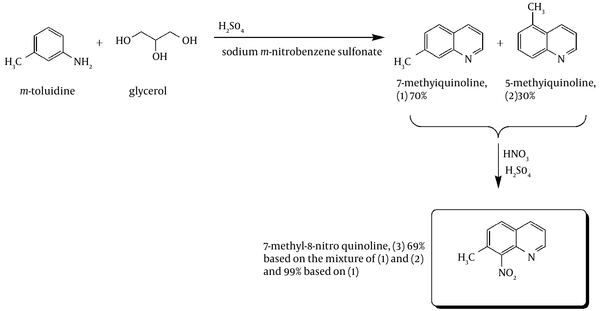
7-methylquinoline: In a round-bottom flask, m-nitrobenzene-sulfonate (135 g, 0.6 mol), glycerol (83.52 g, 0.92 mol), and m-toluidine (50.46 g, 0.47 mol) were mechanically stirred. To this mixture, 273.58 g (2.7 mol) of 98% H2SO4 solution and 61.5 g H2O were added. The addition was drop-wise and the H2SO4/H2O solution had been cooled in an ice bath before the addition. During the addition, the mixture was mechanically stirred and the exothermic reaction was controlled with an ice bath when needed. Once the addition was completed, the solution was heated to reflux (ca. 150°C) for 1 hour (caution: spontaneous exothermicity!). The solution was then cooled in an ice bath and diluted with 330 mL H2O and 43.08 g NaNO3 were added slowly. The solution was then warmed (~70°C) for about 20 minutes to decompose the excess m-toluidine. The solution was cooled again in an ice bath and 400 g of KOH was added slowly to increase the pH of the solution above 10 (pH > 10). The solution was then purified using steam distillation. The clear distillate was cooled, saturated with NaCl and kept in the refrigerator for a couple of nights. The formed brown crude oil was separated from the distillate using a separatory funnel. Then, the distillate was extracted with diethylether (3 × 200 mL). The organic phases were collected, dried with MgSO4, and evaporated. The resulting oil was then further purified using vacuum distillation: bp 91°C (3 mmHg) to give a 70% yield of 7-Methylquinoline (based on GC-MS and 1H NMR).
7-Methylquinoline: 1H NMR (500 MHz, CDCl3) δ 8.85 (d, 1 H), 8.07 (d, 1 H), 7.88 (s, 1 H), 7.68 (d, 1 H), 7.35 (m, 2 H), 2.55 (s, 3 H).
5-Methylquinoline: 1H NMR (500 MHz, CDCl3) δ 8.90 (d, 1 H), 8.28 (d, 1 H), 7.97 (d, 1 H), 7.58 (t, 1 H), 7.39 (m, 1 H), 7.29 (m, 1 H), 2.65 (s, 3 H).
7-Methyl-8-Nitro-quinoline: a solution of 28.5 mL of fuming HNO3 and 85.5 mL of 98% H2SO4 was added dropwise at -5°C, to a mechanically stirred mixture of 57.05 g (0.398 mol) of 7-methylquinoline and 142.5 mL of H2SO4. Once the addition was completed, the cool bath was removed and stirring was continued for 40 min. The solution was then poured over ice and vacuum filtered once the ice was completely dissolved. Additional cold water was added to the filtrate until no more precipitate appeared. The mixture was kept in the refrigerator overnight for precipitation completion. The precipitate was filtered, and then 95% EtOH (3 × 100 mL) was used to wash the solid. The solid was dried under vacuum to afford 51.80 g (69% based on the mixture, 99% based on 7-methylquinoline) of white powder. M.p. = 182-183°C. 1H NMR (CDCl3) δ 8.98 (dd, 1 H), 8.22 (dd, 1 H), 8.87 (d, 1 H), 7.49 (m, 2 H), 2.56 (s, 3 H). IR (KBr):υmax /cm-1 = 3077 w, 1630 w, 1597 msh, 1569 m, 1531 ssh, 1503 ssh, 1464 m, 1447 m, 1433 m, 1381 msh, 1356 msh, 1321 msh, 1229 w, 1202 w, 1145 wsh, 1067 w, 1040 w, 996 w, 959 w, 883 ssh, 844 ssh, 808 ssh, 740 w, 683 w, 647 msh. MS: m/z 188.06 ([M+H]+, 100%). Anal. Calc. for C10H8N2O2 (188.18): C 63.82, H 4.28, N 14.89%; found: C 64.47, H 4.65, N 14.98%.
4. Results
As shown in Figure 1, the first step of the procedure involves a Skraup reaction which is generally considered to proceed with an intermediate formation of the β-arylaminocarboxaldehyde or a Schiff base derived from it, then cyclisation to a tetrahydroquinoline followed by elimination of a water molecule and oxidation of the resulting dihydroquinoline using nitrobenzene salt (Figure 2) (45, 46). In the presence of a strong ortho-para directing group, -CH3, it is expected that the reaction would lead to 7-methylquinoline only (47). However, we observed, based on the 1 H NMR (Figure 3) and GC-MS studies (Figure 4), that the 5-methylquinoline was also formed in the reaction mixture as a minor product which can be interpreted through the cyclisation mechanistic step via an either sides of the NH2 group on the ring (Figure 2).
Mechanism of Skraup Synthesis of 7- and 5-methylquinoline (45, 46)
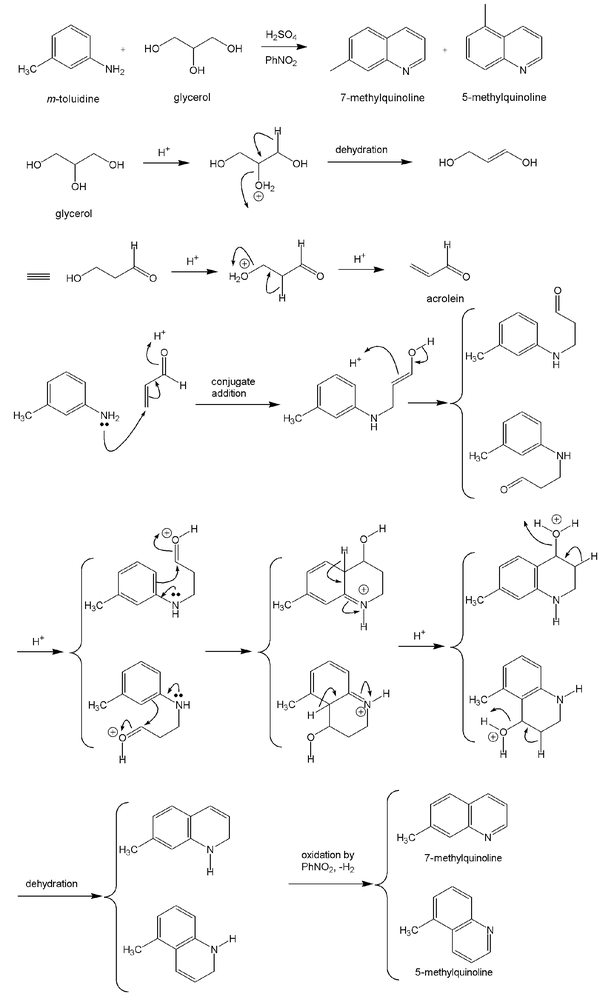
The chemical shifts of the proton signals associated with the protons of the methyl groups in the mixture of 7-methylquinoline and 5-methylquinoline in CDCl 3 have been assigned at 2.5454 and 2.6482 ppm, respectively (Figure 3). The peaks integration interpretation suggests that the products formed with a 2:1 ratio, respectively. The results were also consistent with the GC-MS analysis of the reaction mixture in chloroform solvent (Figure 4). As it is depicted in Figure 4, two peaks were observed in TIC Scan with peak integration of 100:50, for 7-methylquinoline and 5-methylquinoline, respectively. Therefore, the product was shown to consist of a mixture of 7-methylquinoline (70%) and 5-methylquinoline (30%).
1 H NMR Spectrum of the Reaction Mixture of 7- and 5-methylquinoline in CDCl 3 Solvent. The Spectrum Shows the Ratio of 2 : 1 in the Mixture.
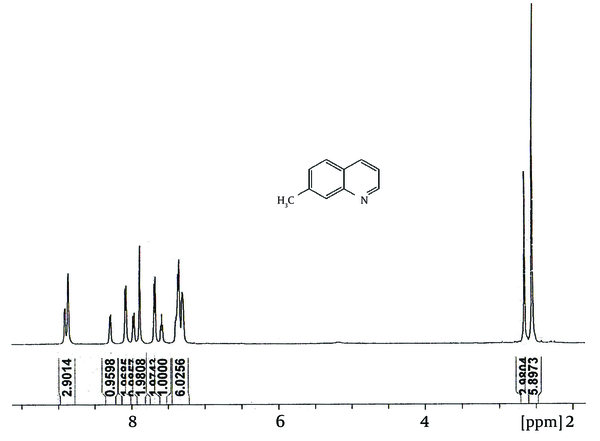
GC-MS Analysis of the Reaction Mixture of 7- and 5-methylquinoline in Chloroform Solvent. The Chromatogram Shows the Ratio of 2 : 1 in the Mixture.
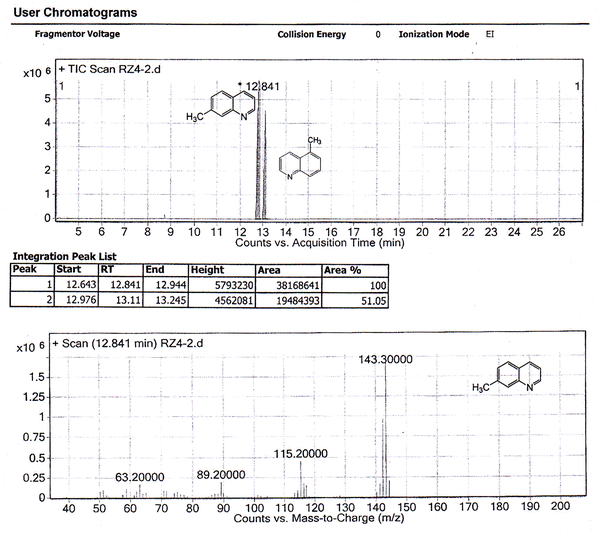
Unfortunately, isolation of the predominant 7-substituted isomer using a variety of methods such as column chromatography, crystallization or distillation techniques remained unsuccessful. It seemed probable, at the first instance, that the 7-methylquinoline used in the above studies contained appreciable amounts of the 5-methylquinoline, the nitration of which would have given rise to additional products (46).
Nitration reaction of the mixture was, therefore, carried out using fuming nitric acid in concentrated sulfuric acid. The product which was obtained was then analyzed by NMR and MS techniques. The 1 H NMR and MS studies showed that only one product was obtained (Figure 5 and Figure 6). Product with a nitro group ortho to a methyl group may be identified from its MS by the facile loss of OH ion from the parent ion. The parent ion mass/charge ratio signal of the nitro product in GC-MS determination was 188.1, while the mass/charge ratio signal of 171.1 can be assigned as [M-OH] + which was consistent with the results reported previously for ortho-nitrotoluene (48).
1 H NMR Spectrum of 7-methyl-8-nitroquinoline in CDCl 3 Solvent
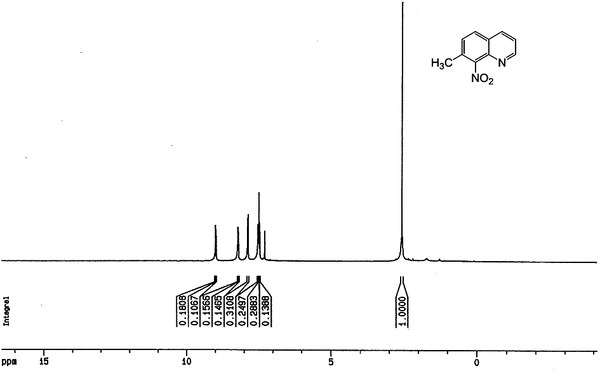
GC-MS Analysis of 7-methyl-8-nitroquinoline in Chloroform Solvent. The Chromatogram Shows the Neat Product Was Formed
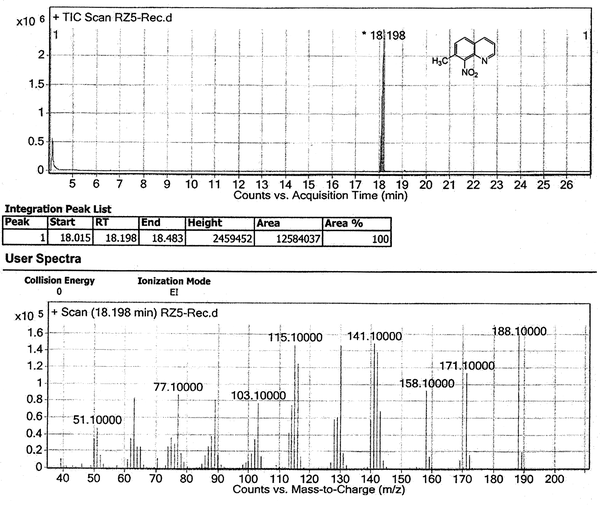
5. Discussion
In our study, nitration of the mixture of 7-methyl- and 5-methyl-quinoline obtained by Skraup synthesis, with fuming HNO3 and concentrated H2SO4 gave 7-methyl-8-nitroquinoline selectively as a white pure powder product; the identity of which was confirmed by the ejection of OH ion from the parent ion in the MS and by a lack of significant benzylic coupling proton in the NMR spectrum. Similar studies were performed by Long and Schofield (49) using a mixture of 7-ethyl- and 5-ethyl-quinoline, but in their study, they observed the formation of a minor 5-ethyl-8-nitroquinoline as a side product, by which the nitration products were contaminated and therefore, the yield of the total synthesis decreased significantly compared to our report. In our study, by contrast, a pure selective product was formed upon nitration of the mixture of 7-methyl- and 5-methyl-quinoline. The formation of other products was not observed either.
Nitration of the mixture of 7-methyl-quinoline results in exclusive formation of 8-isomer, which is the only position remaining in the carboxylic ring that is fully conjugated (ortho) with the methyl group, considering the lesser degree of character of the 6, 7-bond. Based on this interpretation, nitration of 5-methyl-quinoline is expected to occur at both positions 8 and 6, both positions being fully conjugated (para and ortho, respectively) with the methyl group. Surprisingly, these expected products were not formed during the nitration reaction of the mixture. The total yield of nitroderivative was 99% from 7-methylquinoline. This could be due to the result of the reaction conditions that we approached in our laboratory.
Conclusions: A highly efficient selective two-step straightforward synthesis of 7-methyl-8-nitroquinoline from m-toluidine was reported. The synthetic strategy was mediating of two steps. In the first step, the Skraup synthesis of quinolines was employed to produce oil products of 7- and 5-methylquinoline mixtures which were characterized using NMR and MS methods, while the final step was to produce 7-methyl-8-nitroquinoline using mixture of fuming HNO3/conc. H2SO4. The final product was characterized using NMR, MS, IR, and elemental analysis, and its melting point was recorded. The total yield of nitroderivative was 99% from 7-methylquinoline.
Acknowledgements
References
-
1.
El-Subbagh HI, Abu-Zaid SM, Mahran MA, Badria FA, Al-Obaid AM. Synthesis and biological evaluation of certain alpha,beta-unsaturated ketones and their corresponding fused pyridines as antiviral and cytotoxic agents. J Med Chem. 2000;43(15):2915-21. [PubMed ID: 10956199].
-
2.
Watson AA, Fleet GW, Asano N, Molyneux RJ, Nash RJ. Polyhydroxylated alkaloids natural occurrence and therapeutic applications. Phytochemistry. 2001;56(3):265-95. [PubMed ID: 11243453].
-
3.
Jerom BR, Spencer KH. N-(4-Heterocyclic-N-(4-piperidinyl) amides. Eur Pat Appl EP. 1988;277794.
-
4.
Chen Y, Chen I, Tzeng C, Wang T. Synthesis and Cytotoxicity Evaluation of Certain α‐Methylidene‐γ‐butyrolactones Bearing Coumarin, Flavone, Xanthone, Carbazole, and Dibenzofuran Moieties. Helvetica Chimica Acta. 2000;83(5):989-94.
-
5.
Atwell GJ, Baguley BC, Denny WA. Potential antitumor agents. 57. 2-Phenylquinoline-8-carboxamides as "minimal" DNA-intercalating antitumor agents with in vivo solid tumor activity. J Med Chem. 1989;32(2):396-401. [PubMed ID: 2913299].
-
6.
Kuo SC, Lee HZ, Juang JP, Lin YT, Wu TS, Chang JJ, et al. Synthesis and cytotoxicity of 1,6,7,8-substituted 2-(4'-substituted phenyl)-4-quinolones and related compounds: identification as antimitotic agents interacting with tubulin. J Med Chem. 1993;36(9):1146-56. [PubMed ID: 8387598].
-
7.
Xia Y, Yang ZY, Xia P, Bastow KF, Tachibana Y, Kuo SC, et al. Antitumor agents. 181. Synthesis and biological evaluation of 6,7,2',3',4'-substituted-1,2,3,4-tetrahydro-2-phenyl-4-quinolones as a new class of antimitotic antitumor agents. J Med Chem. 1998;41(7):1155-62. [PubMed ID: 9544215]. https://doi.org/10.1021/jm9707479.
-
8.
Roma G, Grossi G, Di Braccio M, Piras D, Ballabeni V, Tognolini M, et al. 1,8-Naphthyridines VII. New substituted 5-amino[1,2,4]triazolo[4,3-a][1,8]naphthyridine-6-carboxamides and their isosteric analogues, exhibiting notable anti-inflammatory and/or analgesic activities, but no acute gastrolesivity. Eur J Med Chem. 2008;43(8):1665-80. [PubMed ID: 18045747]. https://doi.org/10.1016/j.ejmech.2007.10.010.
-
9.
Reddy GV, Kanth SR, Maitraie D, Narsaiah B, Rao PS, Kishore KH, et al. Design, synthesis, structure-activity relationship and antibacterial activity series of novel imidazo fused quinolone carboxamides. Eur J Med Chem. 2009;44(4):1570-8. [PubMed ID: 18775585]. https://doi.org/10.1016/j.ejmech.2008.07.024.
-
10.
Tomasoli I, Pudlo M, de Los Rios C, Soriano E, Colmena I, Gandia L, et al. Eur J Med Chem. 2011;46:1-10.
-
11.
Zhang J, Ke X, Tu C, Lin J, Ding J, Lin L, et al. Novel Cu(II)-quinoline carboxamide complexes: structural characterization, cytotoxicity and reactivity towards 5'-GMP. Biometals. 2003;16(3):485-96. [PubMed ID: 12680713].
-
12.
Marchalant Y, Baranger K, Wenk GL, Khrestchatisky M, Rivera S. Can the benefits of cannabinoid receptor stimulation on neuroinflammation, neurogenesis and memory during normal aging be useful in AD prevention? J Neuroinflammation. 2012;9:10. [PubMed ID: 22248015]. https://doi.org/10.1186/1742-2094-9-10.
-
13.
Tsuji K, Spears GW, Nakamura K, Tojo T, Seki N, Sugiyama A, et al. Synthesis and antinephritic activities of quinoline-3-carboxamides and related compounds. Bioorg Med Chem Lett. 2002;12(1):85-8. [PubMed ID: 11738579].
-
14.
Bu X, Chen J, Deady LW, Smith CL, Baguley BC, Greenhalgh D, et al. Synthesis and cytotoxic activity of N-[(alkylamino)alkyl]carboxamide derivatives of 7-oxo-7H-benz[de]anthracene, 7-oxo-7H-naphtho[1,2,3-de]quinoline, and 7-oxo-7H-benzo[e]perimidine. Bioorg Med Chem. 2005;13(11):3657-65. [PubMed ID: 15862994]. https://doi.org/10.1016/j.bmc.2005.03.033.
-
15.
Silverman RB. The Organic Chemistry of Drug Design and Drug Action. San Diego: Academic Press; 1992.
-
16.
Thompson LA, Ellman JA. Synthesis and Applications of Small Molecule Libraries. Chem Rev. 1996;96(1):555-600. [PubMed ID: 11848765].
-
17.
Franzen RG. Recent advances in the preparation of heterocycles on solid support: a review of the literature. J Comb Chem. 2000;2(3):195-214. [PubMed ID: 10827923].
-
18.
Foley M, Tilley L. Quinoline antimalarials: mechanisms of action and resistance and prospects for new agents. Pharmacol Ther. 1998;79(1):55-87. [PubMed ID: 9719345].
-
19.
Kouznetsov VV, Médez LYV, Góez CMM. Reviews on classical quinoline syntheses. Curr Org Chem. 2005;9:141.
-
20.
Madapa S, Tusi Z, Batra S. Advances in the synthesis of quinoline and quinoline-annulated ring systems. Curr Org Chem. 2008;12:1116-83.
-
21.
Marco-Contelles J, Perez-Mayoral E, Samadi A, Carreiras Mdo C, Soriano E. Recent advances in the Friedlander reaction. Chem Rev. 2009;109(6):2652-71. [PubMed ID: 19361199]. https://doi.org/10.1021/cr800482c.
-
22.
Li An-Hu, Beard DJ, Coate H, Honda A, Kadalbajoo M, Kleinberg A, et al. One-Pot Friedländer Quinoline Synthesis: Scope and Limitations. Synthesis. 2010;2010(10):1678-86.
-
23.
Aghera VK, Patel JP, Parsania PH. Synthesis, spectral and microbial studies of some novel quinoline derivatives via Vilsmeier–Haack reagent. Arkivoc. 2008;12:195-204.
-
24.
Shivaraj Y, Naveen MH, Vijayakumar GR, Kumar DBA. Design, Synthesis and Antibacterial Activity Studies of Novel Quinoline Carboxamide Derivatives. J Korea Chem Soc. 2013;57(2):241-5.
-
25.
Ebenso EE, Obot IB, Murulana LC. Quinoline and its derivatives as effective corrosion inhibitors for mild steel in acidic medium. Int. J. Electrochem. Sci. 2010;5:1574-86.
-
26.
Manske RH, Kulka M. The skraup synthesis of quinolines. Org React. 1953;7:59.
-
27.
Durce JGF. The Preparation of Certain Organic Stanno- and Stanni- chlorides. Chem News. 1918;117:346.
-
28.
Glass M, Dragunow M, Faull RL. The pattern of neurodegeneration in Huntington's disease: a comparative study of cannabinoid, dopamine, adenosine and GABA(A) receptor alterations in the human basal ganglia in Huntington's disease. Neuroscience. 2000;97(3):505-19. [PubMed ID: 10828533].
-
29.
Reiner A, Albin RL, Anderson KD, D'Amato CJ, Penney JB, Young AB. Differential loss of striatal projection neurons in Huntington disease. Proc Natl Acad Sci U S A. 1988;85(15):5733-7. [PubMed ID: 2456581].
-
30.
Allen KL, Waldvogel HJ, Glass M, Faull RL. Cannabinoid (CB(1)), GABA(A) and GABA(B) receptor subunit changes in the globus pallidus in Huntington's disease. J Chem Neuroanat. 2009;37(4):266-81. [PubMed ID: 19481011]. https://doi.org/10.1016/j.jchemneu.2009.02.001.
-
31.
McConnell AJ, Song H, Barton JK. Luminescence of [Ru(bpy)2(dppz)]2+ bound to RNA mismatches. Inorg Chem. 2013;52(17):10131-6. [PubMed ID: 23968195]. https://doi.org/10.1021/ic401531r.
-
32.
Arnold AR, Barton JK. DNA Protection by the Bacterial Ferritin Dps via DNA Charge Transport. J Am Chem Soc. 2013;135(42):15726-9. [PubMed ID: 24117127]. https://doi.org/10.1021/ja408760w.
-
33.
Muren NB, Barton JK. Electrochemical Assay for the Signal-On Detection of Human DNA Methyltransferase Activity. J Am Chem Soc. 2013:[Epub ahead of print]. [PubMed ID: 24164112]. https://doi.org/10.1021/ja4085918.
-
34.
Pheeney CG, Barton JK. Intraduplex DNA-mediated electrochemistry of covalently tethered redox-active reporters. J Am Chem Soc. 2013;135(40):14944-7. [PubMed ID: 24079853]. https://doi.org/10.1021/ja408135g.
-
35.
Pheeney CG, Arnold AR, Grodick MA, Barton JK. Multiplexed electrochemistry of DNA-bound metalloproteins. J Am Chem Soc. 2013;135(32):11869-78. [PubMed ID: 23899026]. https://doi.org/10.1021/ja4041779.
-
36.
Komor AC, Barton JK. The path for metal complexes to a DNA target. Chem Commun (Camb). 2013;49(35):3617-30. [PubMed ID: 23423158]. https://doi.org/10.1039/c3cc00177f.
-
37.
Sontz PA, Muren NB, Barton JK. DNA charge transport for sensing and signaling. Acc Chem Res. 2012;45(10):1792-800. [PubMed ID: 22861008]. https://doi.org/10.1021/ar3001298.
-
38.
Song H, Kaiser JT, Barton JK. Crystal structure of Delta-[Ru(bpy)(2)dppz](2)(+) bound to mismatched DNA reveals side-by-side metalloinsertion and intercalation. Nat Chem. 2012;4(8):615-20. [PubMed ID: 22824892]. https://doi.org/10.1038/nchem.1375.
-
39.
Pheeney CG, Barton JK. DNA electrochemistry with tethered methylene blue. Langmuir. 2012;28(17):7063-70. [PubMed ID: 22512327]. https://doi.org/10.1021/la300566x.
-
40.
Ernst PRJ, Komor AC, Barton JK. Luminescent Properties of Ruthenium(II) Complexes with Sterically. Biochemistry.
-
41.
Barton JK, Olmon ED, Sontz PA. Metal Complexes for DNA-Mediated Charge Transport. Coord Chem Rev. 2011;255(7-8):619-634. [PubMed ID: 21643528]. https://doi.org/10.1016/j.ccr.2010.09.002.
-
42.
Slinker JD, Muren NB, Renfrew SE, Barton JK. DNA charge transport over 34 nm. Nature Chem. 2011;3:230–5.
-
43.
Tomisek A, Graham B, Grifith A, Pease CS, Christensen BE. Syntheses of certain 8-nitroquinolines. J Am Chem Soc. 1946;68:1587-9.
-
44.
Hurley MJ, Mash DC, Jenner P. Expression of cannabinoid CB1 receptor mRNA in basal ganglia of normal and parkinsonian human brain. J Neural Transm. 2003;110(11):1279-88. [PubMed ID: 14628192]. https://doi.org/10.1007/s00702-003-0033-7.
-
45.
Jack LiJ. Name Reactions (Acollection of Detailed Reaction Mechanism). Germany: Springer-Verlag; 2003.
-
46.
Palmer MH. The Skraup reaction. Formation of 5- and 7-substituted quinolines. J Chem Soc. 1962:3645-52.
-
47.
Bradford L, Elliott TJ, Rowe FM. The Skraup reaction with m-substituted anilines. J Chem Soc. 1947:437-45.
-
48.
Beynon JH, Saunders RA, Williams AF. Mass Spectra of Organic Molecules. Amsterdam: Elsevier; 1968.
-
49.
van der Stelt M, Fox SH, Hill M, Crossman AR, Petrosino S, Di Marzo V, et al. A role for endocannabinoids in the generation of parkinsonism and levodopa-induced dyskinesia in MPTP-lesioned non-human primate models of Parkinson's disease. FASEB J. 2005;19(9):1140-2. [PubMed ID: 15894565]. https://doi.org/10.1096/fj.04-3010fje.