Abstract
Background:
Hydroxyapatite (HA) has been widely used in hard-tissue repair applications, such as implant coatings and bone substitutes, because of its stability under in vivo conditions and its osteoconductivity.Objectives:
The aim of this study was to investigate the effects of HA coating applied on two different metallic materials that are used as implants, with regard to the substrate and electrochemical parameters of their microstructure.Materials and Methods:
HA coating was applied on AZ31 magnesium alloy and stainless steel 304, metallic materials used as implants, and the effects of the substrate and electrochemical parameters on their microstructure were investigated.Results:
It was observed that at 2 V potential and 85°C for AZ31 and steel 304, the coatings did not have proper coverage and the grains were coarse. By hindering the grain growth, complete coverage with a uniform and fine structure will be achieved. There was a difference in morphology that was probably due to the different open circuit potentials of these two alloys. Magnesium alloys have more negative potential, in which H2 formation can hinder growth of HA flakes, but in Ti base alloys, the formation of H2 gas does not easily form in the working potential, so the HA coating can grow easily.Conclusions:
Nanosized HA coating can be obtained on stainless steel 304, AZ31 magnesium alloy, and Ti-6Al-4V by cathodic deposition using pulse and direct cathodic electrodeposition from a proper electrolyte solution, regardless of the substrate material.Keywords
1. Background
Hydroxyapatite (HA) has been widely used in hard-tissue repair applications, such as implant coatings (1) and bone substitutes (2), because of its stability under in vivo conditions and its osteoconductivity. The apatite crystallites in human bone, enamel, dentin, and cementum are all extremely small in size (2) and can be considered nanostructure materials. HA [Ca10(PO4)6(OH)2] is a biocompatible and bioactive ceramic, capable of interacting and chemically bonding with surrounding bone. However, it cannot be directly used for implant devices because of its brittleness and strength limitations, and hence a great deal of research is concentrated on the development of HA coatings and composites (3, 4).
Growing research for biodegradable materials for repair applications has led to the introduction of some biodegradable polymers, but they did not have the required mechanical strength. Magnesium and its alloys have mechanical strength and are biocompatible, and most importantly, they are biodegradable (5). Application of HA onto magnesium and its alloys not only improves bone compatibility but can control the corrosion rate.
In this respect, biodegradable magnesium-based alloys can be a potential solution. Many clinical cases, as well as in vivo and in vitro assessments, have demonstrated that magnesium alloys possess good biocompatibility (6-9). Among the many techniques investigated for the coating of HA on metallic surfaces (10), electrodeposition of HA has attracted interest due to its simplicity, low cost, and ability to control microstructures. This can be done by controlling the deposition process, which makes it possible to control the size and structure of the coating (11, 12).
2. Objectives
In this study, the effects of substrate, electrolyte temperature, and electrodeposition method on the microstructure of HA coatings were investigated.
3. Materials and Methods
The substrate materials were AZ31 magnesium alloy, with a size of 10 × 10 mm, and Ti-6Al-4V, with a size of 30 × 20 × 3 mm. The sample surfaces of the AZ31 magnesium alloy were ground with 1200-grit SiC paper to ensure the same surface roughness, while for Ti-6Al-4V, abrasive paper number 180 was used. The electrolyte solution contained 0.042 M of Ca(NO3)2 and 0.025 M of NH4H2PO4 at pH of 4.2. Electrodeposition was carried out at stable cathodic potentials of 2, 2.5, and 3 V for 30 minutes at room temperature, and at 85°C for the AZ31 samples. The pulsed potential technique was used at different on/off periods for the Ti-6Al-4V samples. The surface morphology of the coatings was observed using a scanning electron microscope (SEM, model CamScan MV2300). The coating crystal structures were identified by X-ray diffraction using Philips PW1700 XRD.
4. Results and Discussion
Figure 1 shows the morphology of HA coating on AZ31 magnesium alloy (Figure 1A) and stainless steel 304 (Figure 1B) at room temperature.
Surface Morphology of HA Coating With Applied Potentials of 2 V Formed on AZ31 Magnesium Alloy, Seen With Optical Microscopy (A), and Stainless Steel 304, Seen With SEM (B) at Room Temperature
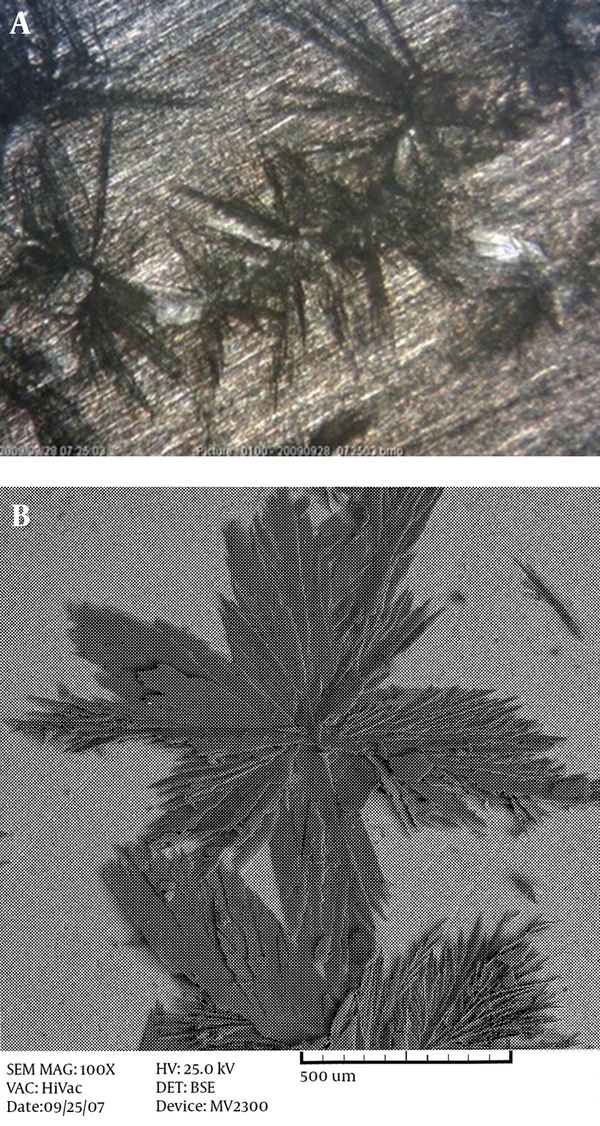
As can be seen from the illustrations, at this temperature, the coatings do not have the proper coverage and the grains are coarse. By hindering the grain growth, complete coverage with a uniform and fine structure will result. When the production of atoms is fast and the diffusion rate of adatoms to kink sites in the network structure is low, the structure will be fine. In this case, by increasing the temperature, the rate of nucleation and diffusion is increased, but the chance of atoms reaching the right location is decreased. Hence, the growth is mainly in a longitudinal direction and the structure will become narrower. However, at 85°C, grains with a nano-width size are produced. Figure 2 shows the morphology of the HA coating on the Ti-6AL-4V alloy (Figure 2A) and the AZ31 magnesium alloy (Figure 2B). It can be seen that a fibrous structure is formed on the magnesium alloy, and the macrostructure is a honeycomb-like structure, while a flake-like morphology is observed on the Ti alloy. With the same surface preparation and surface roughness, this difference in morphology is probably due to the difference in the open circuit potential of these two alloys. Magnesium alloys have more negative potential, in which H2 formation can hinder the growth of HA flakes, but in Ti base alloys, the formation of H2 gas does not easily form in the working potential, so the HA coating can grow easily. The same fibrous-like morphology is obtained when using stainless steel 304 as the substrate, with a cathodic potential of 3 V (Figure 3).
Surface Morphology of HA Coating With Applied Potentials of 3 V Formed on Ti-6AL-4V (A) and AZ31 Magnesium Alloys (B)
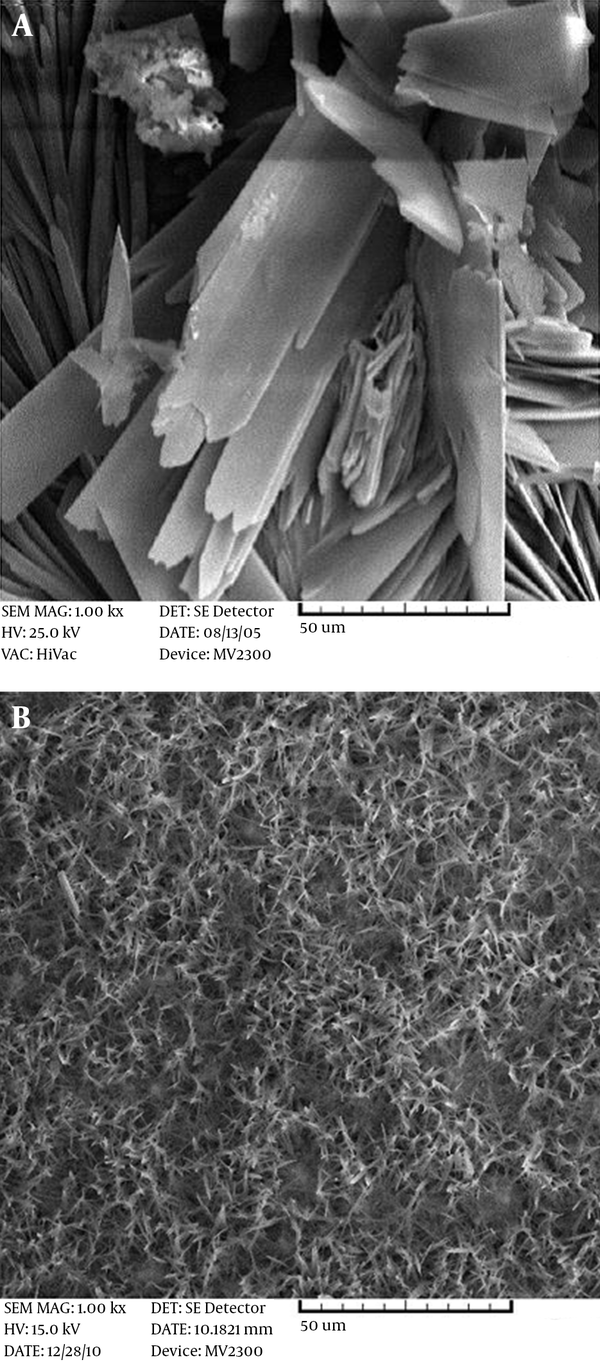
Surface Morphology of HA Coating With Applied Potentials of 3 V Formed on Stainless Steel 304
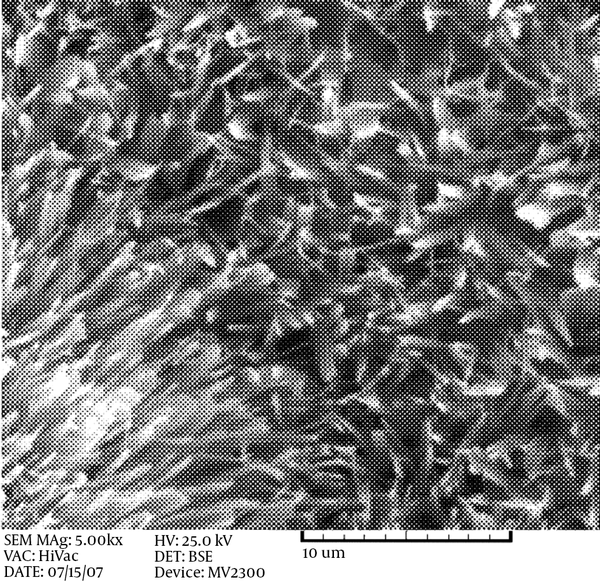
In electrolytic deposition, the formation of a deposit layer begins with the nucleation and growth of a colloidal mass. The possibility of nucleation in unit-time is increased by increasing the potential, and the chance for growing these nuclei is reduced. Thus, the growth mechanism is retarded and the structure becomes finer. In contrast, it is observed that by increasing the potential, a coarse coating layer is formed on the samples. This phenomenon can be explained by the DLVO theory. At the beginning of the coating process with high applied voltage, the electrochemical reactions can occur so quickly that the ion concentration around the electrode decreases. Therefore, a critical concentration on the electrode surface is needed to overcome the repulsion forces. The electrochemical reactions are almost stopped because of this reduction in concentration, and the motivating applied force is used for grain growth under a diffusion-control situation. It was observed that with magnesium alloys, high applied potential produced more and more hydrogen gas, which prevented deposition in spite of the higher hydroxyl ion generation. The applied potential of 2 V is the optimum potential at which the coating is uniform with a fine structure (Figure 4). Pulse electrodeposition is a useful method that can modify the deposition process, leading to controlled size and structure. With the use of the pulse electrodeposition method, the flake-like structure of HA is changed to a cluster type of nanosized particles. The off-time was very important because a high number of nuclei produced at the high potential during the on-time had no chance to grow, and consequently, nanosized particles were produced.
Surface Morphology of HA Coating With Applied Potentials of 2 V Formed on AZ31 Magnesium Alloy at Two Different Magnifications
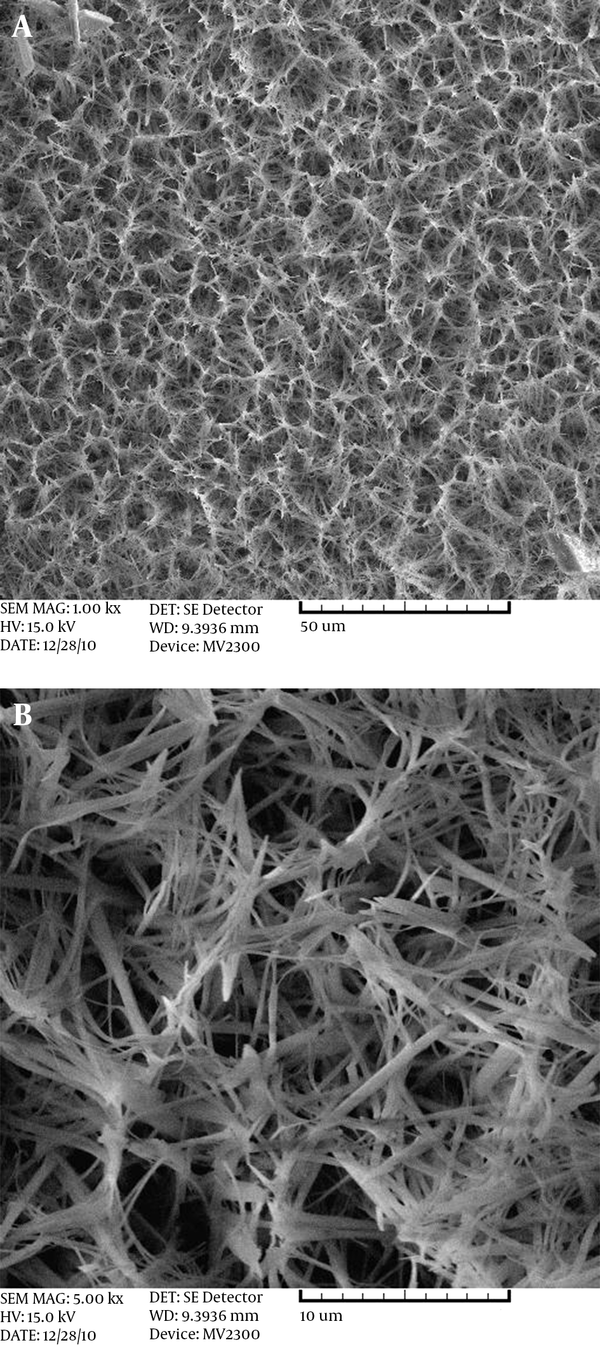
An energy dispersive X-ray (EDX) analysis of the coating with conventional and pulse electrodeposition methods showed that by changing the particle size to nano, the Ca/P ratio changed from 1.08 to 1.38, which is closer to the ideal ratio of 1.67 for HA crystals.
5. Conclusions
Nanosized HA coating can be obtained on stainless steel 304, AZ31 magnesium alloy, and Ti-6Al-4V by cathodic deposition using pulse and direct cathodic electrodeposition from a proper electrolyte solution (0.042 M of Ca(NO3)2 and 0.025 M of NH4H2PO4 at pH 4.2), regardless of the substrate material. However, the deposition parameters have a noticeable effect on the morphology of the coating. The morphology of the coating can be controlled by maintaining applied potential and electrolyte temperature, namely at 2 V and 85°C, respectively. The structure of the coating can be finer when the cathodic potential is decreased to 2 V.
Acknowledgements
References
-
1.
Jaffe WL, Scott DF. Total hip arthroplasty with hydroxyapatite-coated prostheses. J Bone Joint Surg Am. 1996;78(12):1918-34. [PubMed ID: 8986672].
-
2.
LeGeros RZ. Calcium phosphates in oral biology and medicine. Monogr Oral Sci. 1991;15:1-201. [PubMed ID: 1870604].
-
3.
Cameron HU, Macnab I, Pilliar RM. Evaluation of biodegradable ceramic. J Biomed Mater Res. 1977;11(2):179-86. [PubMed ID: 853042]. https://doi.org/10.1002/jbm.820110204.
-
4.
Mears DC. Materials and Orthopedic Surgery. Baltimore: The Williams and Wilkins Company; 1972.
-
5.
Sivakumar M, Mudali UK, Rajeswari S. Compatibility of ferritic and duplex stainless steels as implant materials: in vitro corrosion performance. J Mater Sci. 1993;28(22):6081-6. https://doi.org/10.1007/bf00365025.
-
6.
McBride ED. Absorbable Metal in Bone Surgery. J Am Med Assoc. 1938;111(27):2464-7. https://doi.org/10.1001/jama.1938.02790530018007.
-
7.
Vormann J. Magnesium: nutrition and metabolism. Mol Aspects Med. 2003;24(1-3):27-37. [PubMed ID: 12537987].
-
8.
Witte F, Kaese V, Haferkamp H, Switzer E, Meyer-Lindenberg A, Wirth CJ, et al. In vivo corrosion of four magnesium alloys and the associated bone response. Biomaterials. 2005;26(17):3557-63. [PubMed ID: 15621246]. https://doi.org/10.1016/j.biomaterials.2004.09.049.
-
9.
Chang E, Chang WJ, Wang BC, Yang CY. Plasma spraying of zirconia-reinforced hydroxyapatite composite coatings on titanium: part I: phase, microstructure and bonding strength. J Mater Sci Mater Med. 1997;8(4):193-200. [PubMed ID: 15348759].
-
10.
Wei M, Ruys AJ, Milthorpe BK, Sorrell CC. Solution ripening of hydroxyapatite nanoparticles: effects on electrophoretic deposition. J Biomed Mater Res. 1999;45(1):11-9. [PubMed ID: 10397952].
-
11.
Damodaran R, Moudgil BM. Electrophoretic deposition of calcium phosphates from non-aqueous media. Colloids and Surfaces A: Physicochemical and Engineering Aspects. 1993;80(2-3):191-5. https://doi.org/10.1016/0927-7757(93)80198-n.
-
12.
Shirkhanzadeh M. Direct formation of nanophase hydroxyapatite on cathodically polarized electrodes. J Mater Sci Mater Med. 1998;9(2):67-72. [PubMed ID: 15348909].