Abstract
Background:
The roles of hyperkalemic cardioplegia (CPG) and endothelial ATP-sensitive potassium (KATP) channels in endothelial protection against ischemia-reperfusion injury (IRI) during cardiac surgery remain unclear.Objectives:
This study aimed to investigate the impacts of KATP channel openers (KCOs) on endothelial cell viability and nitric oxide (NO) production under normal conditions and cardioplegia exposure after short and long reperfusion.Methods:
Human endothelial cells (EA.hy926) were subjected to ischemia-reperfusion (IR), with or without the KCOs, including pinacidil, diazoxide, nicorandil, and ZD0947. The effects of nicorandil and ZD0947 were also evaluated in conjunction with cardioplegia. The KCOs preserved endothelial cell viability against IRI under standard conditions at both time points.Results:
Ischemia-reperfusion significantly reduced NO production at 24 hours, while ZD0947 maintained early NO levels and improved NO production at 24 hours, comparable to nicorandil. Cardioplegia alone did not affect cell viability or NO production; however, its application under IR conditions was more detrimental than IR alone. Notably, nicorandil and ZD0947 preserved cell viability in this context. Nitric oxide levels at 30 minutes remained at basal levels in the cardioplegia, IR, and cardioplegia with IR groups. However, treatment with nicorandil and ZD0947 in the cardioplegia with IR condition significantly increased NO levels compared to the control.Conclusions:
ZD0947 and nicorandil effectively mitigated endothelial cell damage in response to IR with cardioplegia.Keywords
KATP Channel Openers Cardio Protection Endothelial Cells Ischemia-Reperfusion Injury Cardioplegia Nitric Oxide
1. Background
Oxygen reperfusion to ischemic tissues can result in ischemia-reperfusion injury (IRI), causing cell damage and death (1). Aortic cross-clamping/unclamping and intermittent perfusion of cardioplegia are recognized contributors to myocardial IRI during cardiac surgery (2). Over the past two decades, the direct effects of ischemia-reperfusion (IR) on myocardial cells have been extensively investigated (3), and these effects are believed to play a role in endothelial dysfunction (4). The altered production of endothelial reactive oxygen species and nitric oxide (NO) induced by IRI is linked to vascular dysfunction and microvascular obstruction, which can subsequently lead to inadequate myocardial perfusion (5). Furthermore, the disruption of endothelial cell-cardiomyocyte crosstalk exacerbates susceptibility to myocardial IRI. A previous study demonstrated that co-culturing pretreated human umbilical vein endothelial cells (HUVECs) improved myocardial cell survival in response to IRI (6). The connection between endothelial functions and myocardial IRI has garnered significant attention in recent years, highlighting the roles of the endothelium and NO as therapeutic targets for cardioprotection during cardiac surgery (7). Notably, ATP-sensitive potassium (KATP) channels, ion channels expressed in the endothelium and myocardium, have emerged as regulators of membrane potential that may influence endothelial NO production (7).
The KATP channels are octameric complex ion channels composed of four Kir6.x family subunits and four sulfonylurea receptors (SUR). These channels play a crucial role in connecting the metabolic state to the electrical activity of the cells in which they are expressed. The common subunits found in endothelial cells, vascular smooth muscle cells, and cardiomyocytes include Kir6.1, Kir6.2, and SUR2B. Specifically, the Kir6.1/SUR2B channel, present in both endothelial and vascular smooth muscle cells, is vital for vascular regulation, including vasodilation, vasoconstriction, and the production of vasoactive substances (8, 9). Activation of vascular KATP channels results in hyperpolarization and subsequent vasodilation. Similarly, the modulation of endothelial KATP channels contributes to vascular mediator secretion and angiogenesis (10). The significance of endothelial KATP channels in cardiac protection has been underscored by studies showing increased myocardial IRI in mice with endothelial Kir6.1 deletion (11). Additionally, a Kir6.1-containing modulator exhibited anti-hypertrophic effects in a heart failure model, likely mediated through endothelial cells and NO production (12). These findings suggest that endothelial KATP channels may play a key role in mitigating IRI-induced endothelial and myocardial dysfunction during cardiac surgery.
The protective effects of KATP channel openers (KCOs) on endothelial cell functions have been well-documented. For instance, pinacidil, a nonselective KCO, and diazoxide, a cardiac mitochondrial KATP channel activator, have been shown to hyperpolarize the endothelial cell membrane potential in rat aorta, leading to increased intracellular Ca2+ and enhanced NO secretion (13). Similarly, nicorandil, which functions as both a nitrate and a mitochondrial KATP channel opener, has demonstrated protective effects against ischemia in human pulmonary artery endothelial cells by regulating cell apoptosis and NO production (14).
Another promising modulator subgroup for endothelial preservation is the Kir6.1/SUR2B opener, which includes levcromakalim, ZD0947, and A278637 (15). For example, iptakalim has been shown to enhance endothelial NO production and inhibit endothelin-1 secretion in aortic endothelial cells (16).
Hyperkalemic cardioplegia (CPG) is extensively employed for myocardial protection during open-heart surgery. Under high potassium conditions, the cell membrane becomes depolarized, leading to action potential disruption and intracellular Ca2+ loading (17). However, the protective effects of CPG on cell viability and NO production remain controversial. Yang et al. demonstrated that CPG did not affect porcine coronary artery NO levels but impaired endothelial-derived hyperpolarizing factor-mediated vasorelaxation, which could be partially restored by nicorandil (18). Conversely, Von Oppell et al. reported a decrease in endothelial cell survival after 24 and 36 hours of exposure to CPG (19), while Evora et al. observed that crystalloid CPG did not impair endothelium-dependent relaxation (20). Furthermore, the protective effects of combining CPG with KCOs against IRI are not yet well understood.
2. Objectives
This study aims to investigate the effects of KCOs, CPG, and the combination of CPG with KCOs on endothelial cell viability and NO production in response to IRI.
3. Methods
3.1. Chemicals and Solutions
The simulated ischemic solution consisted of the following components: 140 mM NaCl, 6 mM KCl, 1 mM MgCl2, 1 mM CaCl2, 5 mM HEPES, 10 mM 2-deoxy-D-glucose, and 10 mM sodium dithionite. The composition of CPG was consistent with clinical practice, comprising 100 mL of acetate Ringer solution, 2 mL of St. Thomas cardioplegia solution (containing 16 mM KCl, 16 mM MgCl2, and 1 mM procaine hydrochloride), and 1.1 mL of 7.5% NaHCO3. ZD094, pinacidil monohydrate, nicorandil, and glibenclamide were obtained from Sigma-Aldrich, while diazoxide was purchased from Abcam. These chemicals were dissolved in dimethyl sulfoxide (DMSO) at final concentrations of 0.001% (pinacidil, diazoxide, and nicorandil), 0.002% (ZD094), and 0.1% (glibenclamide).
3.2. Cell Culture
Endothelial cells (EA.hy926), obtained from the American Type Culture Collection (ATCC, CRL-2922™, USA), were cultured in a T75 flask using Dulbecco’s Modified Eagle’s Medium (DMEM) supplemented with 10% fetal bovine serum and 1% penicillin-streptomycin. The cells were maintained at 37°C in a humidified environment with a 5% CO2 - 95% air mixture. Typically, an overnight incubation results in 70 - 90% cell confluency, which is optimal for subsequent experiments.
3.3. Cell Viability Test
3-(4,5-Dimethylthiazol-2-yl)-2,5-diphenyltetrazolium bromide (MTT) solution at a concentration of 5 mg/mL was freshly prepared by dissolving MTT powder (VWR Life Science®) in DMEM. This solution was added to each well of the cultured cells to achieve a final concentration of 0.5 mg/mL, and the cells were incubated at 37°C for 2 hours. After incubation, the supernatant was carefully removed, and 500 µL of dimethyl sulfoxide was added to dissolve the purple formazan crystals. The absorbance of the samples was measured at a wavelength of 570 nm using a microplate reader. The relative cell viability of the samples was calculated using the following formula:
3.4. Nitric Oxide Assay
The NO concentration was quantified using the commercial colorimetric NO Assay Kit (Ab272517, Abcam, United Kingdom) according to the manufacturer’s protocol. Briefly, cell culture supernatants from the control and treated wells were deproteinized by treatment with ZnSO4 and NaOH. Subsequently, 100 µL of the supernatant was mixed with 200 µL of working reagent and incubated at 37°C for 60 minutes. After incubation, the mixture was centrifuged at 14,000 rpm for 10 minutes, and the supernatant was transferred to a 96-well plate for NO level measurement using a microplate reader (SpectraMax® iD3) at an excitation wavelength of 540 nm. A standard curve was generated for each assay, and only assays with an R² value greater than 0.95 were considered valid. Nitrite measurements for the standards, controls, and treated samples were conducted in duplicate. The relative NO production (%) of the sample was calculated using the following formula:
3.5. Optimization of Ischemia-Reperfusion Model
The IR model was optimized by varying ischemic durations, including a control (no ischemia) and 30, 45, 60, 90, 120, and 150 minutes. Simulated ischemia was induced by replacing the cell medium with 400 µL of simulated ischemic solution at 37°C. After the ischemic period, the cells were reperfused by rebathed in normoxic DMEM for 10 minutes before being processed for the MTT assay.
To determine the optimal concentration of KCOs, the cells were exposed to different concentrations of KCOs added to the simulated ischemic solution for 120 minutes. These concentrations included 1, 10, 100, 500, and 1,000 µM of ZD0947; 0.1, 1, 10, 100, 500, and 1,000 µM of pinacidil; 0.1, 1, 10, 100, and 500 µM of diazoxide; and 0.1, 1, 10, 100, and 500 µM of nicorandil. Following ischemic exposure, the cells were reperfused by replacing the simulated ischemic solution with DMEM for 10 minutes before assessing cell viability. Each optimization test was conducted in triplicate and repeated three times (n = 3 × 3).
3.6. Evaluating the Impact of KATP Channel Openers Against Ischemia-Reperfusion Injury
The cells were divided into the following groups: Normal control (C), IR, and IR with KCO treatment. The latter group was further subdivided based on the specific treatment: IR-P, IR-D, IR-N, and IR-Z, representing treatment with 1 µM pinacidil, 1 µM diazoxide, 1 µM nicorandil, and 1 µM ZD0947, respectively. To block the effect of KCOs, glibenclamide (G) was added. The MTT assay was performed after 10 minutes and 24 hours of reperfusion. Additionally, supernatant NO levels were measured at 30 minutes and 24 hours of reperfusion (Figure 1A n = 4 × 3).
Experimental protocols for investigating the effects of CPG and KCOs in response to IR. A, in the absence of CPG; B, in the presence of CPG. PBS: Phosphate-buffered saline; IR: Ischemia-reperfusion; IR-P: Ischemia-reperfusion with pinacidil treatment; IR-PG: Ischemia-reperfusion with pinacidil and glibenclamide treatment; IR-D: Ischemia-reperfusion with diazoxide treatment; IR-DG: Ischemia-reperfusion with diazoxide and glibenclamide treatment; IR-N: Ischemia-reperfusion with nicorandil treatment; IR-NG: Ischemia-reperfusion with nicorandil and glibenclamide treatment; IR-Z: Ischemia-reperfusion with ZD0947 treatment; IR-ZG: Ischemia-reperfusion with ZD0947 and glibenclamide treatment; MTT: 3-(4,5-Dimethylthiazol-2-yl)-2,5-diphenyltetrazolium bromide; NO: Nitric oxide; CPG: Cardioplegia; I-CPGR: Cardioplegia with ischemia-reperfusion; I-CPGR-N: Cardioplegia with ischemia-reperfusion followed by nicorandil treatment; I-CPGR-Z: Cardioplegia with ischemia-reperfusion followed by ZD0947 treatment; DMEM: Dulbecco's Modified Eagle Medium.
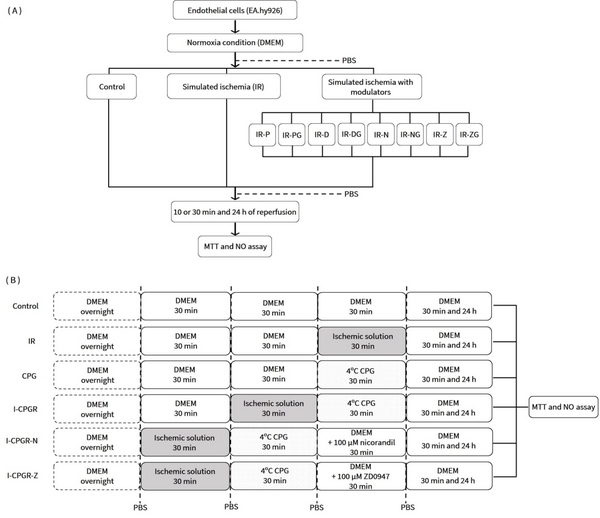
To simulate IRI during CPG administration, the cells were incubated with simulated ischemic solution for 30 minutes before reperfusion with CPG. Following this, the cells were incubated with normoxic DMEM, with or without a KCO, for 30 minutes. The MTT assay and NO measurement were conducted after 30 minutes and 24 hours of reperfusion, as outlined in Figure 1B. This experiment was repeated five times (n = 5 × 3).
3.7. Statistical Analysis
The data with a normal distribution are presented as mean ± standard error of the mean (SEM). One-way analysis of variance (ANOVA) with Fisher’s least significant difference (LSD) post hoc test was used to compare relative cell viability and relative NO production among the groups. Statistical significance was defined as P < 0.05. Graphs and statistical analyses were generated and performed using SPSS version 23.
4. Results
4.1. Optimization of the Ischemia-Reperfusion Model and the Identification of Optimal KATP Channel Opener Concentration
The results indicated a significant reduction in relative cell viability after 30 minutes of ischemia, with further decreases observed at 53.46 ± 9.66% and 52.17 ± 12.32% following 120 and 150 minutes of ischemia, respectively. Based on these findings, a 120-minute ischemia period followed by a 10-minute reperfusion was selected as the IR model. Treatment with the KCOs pinacidil and diazoxide at varying concentrations (1, 10, and 100 μM) significantly enhanced cell viability compared to the IR group. Similarly, cells treated with nicorandil (0.1, 1, and 10 μM) or ZD0947 (1, 10, and 100 μM) exhibited improved viability (Figure 2). Consequently, pinacidil, diazoxide, and ZD0947 at a concentration of 1 μM, along with nicorandil at a concentration of 10 μM, were chosen for subsequent experiments.
Relative cell viability responded to varying concentrations of KCOs. * P < 0.05 vs. control; # P < 0.05 vs. IR. IR: Ischemia-reperfusion; IR-P: Ischemia-reperfusion with pinacidil treatment; IR-D: Ischemia-reperfusion with diazoxide treatment; IR-N: Ischemia-reperfusion with nicorandil treatment; IR-Z: Ischemia-reperfusion with ZD0947 treatment.
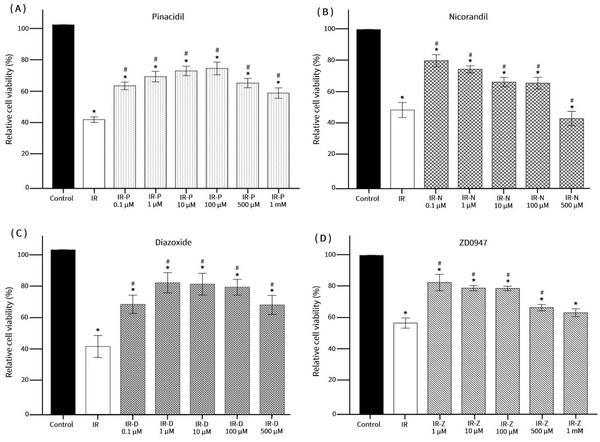
4.2. Effects of KATP Channel Openers on Endothelial Cell Viability in Response to Ischemia-Reperfusion
Figure 3 illustrates the protective effects of KCOs on cell viability under IR conditions after 10 minutes and 24 hours of reperfusion. The addition of nicorandil and ZD0947 to the simulated ischemic solution demonstrated a significant protective effect on endothelial cells. After 10 minutes of reperfusion, cell viability increased from 49.51 ± 3.80% in the IR group to 89.47 ± 7.80% in the IR-N group and 92.48 ± 6.42% in the IR-Z group. Similarly, cell viability in the IR-P and IR-D groups was significantly higher than that in the IR group (Figure 4A). However, the addition of glibenclamide (G) partially inhibited the protective effects of the KCOs.
The effects of KCOs on cell viability during IR. A, cell viability after 10 min of reperfusion; B, cell viability after 24 h of reperfusion. * P < 0.05 vs. control; # P < 0.05 vs. IR. IR: Ischemia-reperfusion; IR-P: Ischemia-reperfusion with pinacidil treatment; IR-PG: Ischemia-reperfusion with pinacidil and glibenclamide treatment; IR-D: Ischemia-reperfusion with diazoxide treatment; IR-DG: Ischemia-reperfusion with diazoxide and glibenclamide treatment; IR-N: Ischemia-reperfusion with nicorandil treatment; IR-NG: Ischemia-reperfusion with nicorandil and glibenclamide treatment; IR-Z: Ischemia-reperfusion with ZD0947 treatment; IR-ZG: Ischemia-reperfusion with ZD0947 and glibenclamide treatment.
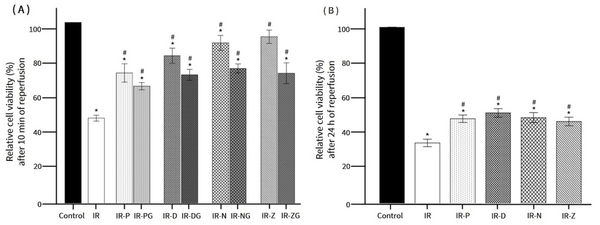
The effects of KATP channel openers (KCOs) on NO production. A, NO production after 30 min of reperfusion; B, NO production after 24 h of reperfusion. * P < 0.05 vs. control; # P < 0.05 vs. IR. IR: Ischemia-reperfusion; IR-P: Ischemia-reperfusion with pinacidil treatment; IR-D: Ischemia-reperfusion with diazoxide treatment; IR-N: Ischemia-reperfusion with nicorandil treatment; IR-Z: Ischemia-reperfusion with ZD0947 treatment.
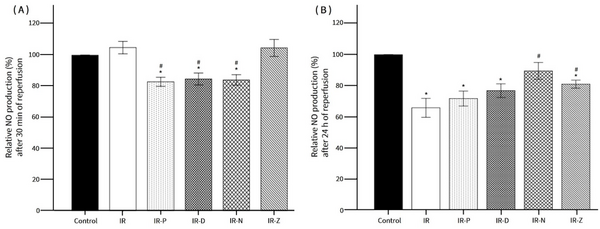
4.3. Effects of KATP Channel Openers on Endothelial Nitric Oxide Production During Ischemia-Reperfusion
At the 30-minute reperfusion time point, the relative NO levels in both the IR and IR-Z groups were comparable to those in the control group. In contrast, the NO levels in the IR-P, IR-D, and IR-N groups were significantly reduced compared to the control group (Figure 4A). After 24 hours of reperfusion, the relative NO production in the IR, IR-P, IR-D, and IR-Z groups showed a significant decrease compared to the control group and was lower than the NO levels measured at the 30-minute reperfusion time point for each respective group (Figure 4B). Notably, the IR-N group exhibited a significant increase in NO levels compared to the IR group after 24 hours of reperfusion.
4.4. Impacts of CPG on Cell Viability and Nitric Oxide Production Against Ischemia-Reperfusion Injury
The results demonstrated that exposure to CPG did not adversely affect cell viability at either the 30-minute or 24-hour reperfusion time points. However, it is noteworthy that CPG under IR conditions (I-CPGR) significantly reduced cell survival compared to the control, CPG alone, and IR alone (Figure 5).
Effects of nicorandil and ZD0947 on cell viability and nitric oxide (NO) production in response to IR and CPG. A, cell viability after 30 min of reperfusion; B, cell viability after 24 h of reperfusion; C, NO production after 30 min of reperfusion; D, NO production after 24 h of reperfusion. * P < 0.05 vs. control; β P < 0.05 vs. I-CPGR. CPG: Cardioplegia; IR: Ischemia-reperfusion; I-CPGR: Cardioplegia with ischemia-reperfusion; I-CPGR-N: Cardioplegia with ischemia-reperfusion followed by nicorandil treatment; I-CPGR-Z: Cardioplegia with ischemia-reperfusion followed by ZD0947 treatment.
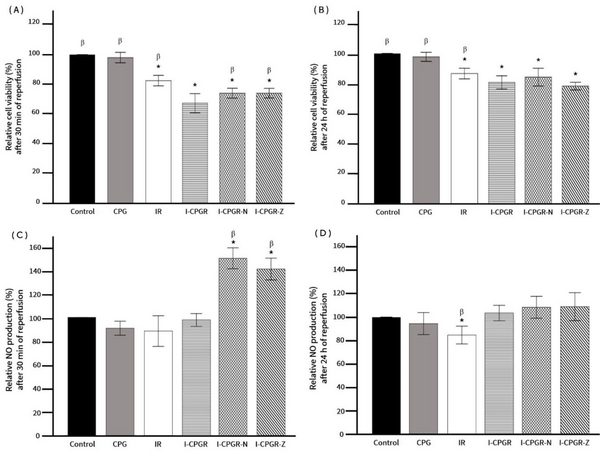
Further evaluation of the effects of nicorandil and ZD0947 on cell viability and NO production indicated that both KCOs effectively enhanced cell viability and NO production compared to I-CPGR at the 30-minute reperfusion time point (Figure 5A and C). However, after 24 hours of reperfusion, neither KCO demonstrated an improvement in cell viability compared to I-CPGR (Figure 5B), and NO levels in both KCO-treated groups returned to levels comparable to the control (Figure 5D).
5. Discussion
In the present study, IR clearly exerted detrimental effects on endothelial cells, while KCOs demonstrated protective effects on endothelial cells in terms of cell viability and NO production under IR conditions. A prior study reported that IRI-induced endothelial cell apoptosis could be detected at an early stage (5 minutes) of reperfusion and preceded cardiomyocyte apoptosis (21). This suggests that soluble pro-apoptotic mediators released from endothelial cells are associated with cardiomyocyte apoptosis. Additionally, cell migration and autophagy have been documented as mechanisms of endothelial dysfunction during IR (22).
In the absence of CPG, we observed that the supernatant NO level in the IR group remained stable at the early stage of reperfusion, even as cell viability dropped to 50%. However, a significant decrease in NO levels was noted at 24 hours of reperfusion. The link between reduced cell viability and stable NO production in response to IRI may involve inducible NO synthase activity and apoptosis. Ischemia-reperfusion -induced apoptosis of HUVECs has been shown to involve inducible NO synthase activity (22).
In the current study, ZD0947 was found to maintain NO production at levels similar to the IR group. The mechanism underlying ZD0947's ability to increase NO production is unclear and warrants further investigation. We hypothesize that ZD0947 may act through a mechanism distinct from IR, as it exhibited a protective effect on endothelial cell survival. ZD0947 may influence NO release through changes in membrane potential, potentially via the activation of endothelial membrane KATP channels, which leads to membrane hyperpolarization, elevated intracellular calcium levels, and subsequent NO release.
However, it remains to be investigated whether ZD0947 also plays a role in endothelial NO synthase phosphorylation, as suggested in a previous study (23). The initial reduction in NO levels observed in the pinacidil, diazoxide, and nicorandil groups was a notable finding. We hypothesize that these potassium channel openers may influence KATP channels beyond the endothelial membrane, potentially leading to a limited alteration in membrane potential and NO release during the initial phase while still preserving cell viability.
The effect of CPG against ischemia and IRI remains a subject of debate. To the best of our knowledge, this study is the first to explore the immediate effects of CPG, both alone and in combination with IR, on endothelial cell viability and NO production. Our findings indicate that CPG does not impair endothelial cell viability or NO production. These results align with previous studies showing that a hyperkalemic solution (16 μM) could protect endothelial cells and cardiomyocytes against IRI by reducing diastolic intracellular Ca²⁺ levels (20, 24).
However, another study reported that the use of St. Thomas cardioplegia with redosing resulted in a significant decrease in HUVEC viability at 24 hours post-application, while no such effect was noted at the 2-hour mark (25). This discrepancy may stem from differences in the composition of cardioplegia used and variations in experimental design. In the current study, a single dose of cardioplegia was used to minimize confounding effects related to cell loss during redosing.
We demonstrated that the adverse effects of IRI on cell viability were significantly exacerbated when combined with CPG application. This finding aligns with a study showing that the glycocalyx—a mesh-like structure crucial for maintaining endothelial permeability—was rapidly degraded after reperfusion in patients undergoing aortic valve replacement with blood cardioplegia (26). The involvement of KATP channels in these effects is presumed. The opening of KATP channels in response to low ATP levels combined with increased extracellular potassium may influence potassium efflux, resulting in action potential alternans and disrupted Ca²⁺ homeostasis. Previous computational simulations have demonstrated that the altered membrane potential caused by extracellular hyperkalemia and IR is regulated by the Na⁺-K⁺ pump and KATP channels (27).
Apoptotic cell death is another critical concern. In this study, nicorandil—a KCO with NO-donating properties—and ZD0947, a Kir6.1/SUR2B-specific KCO, preserved cell viability during IRI in the presence of CPG. Notably, both KCOs significantly increased NO levels in the early phase of reperfusion. The enhanced NO production associated with nicorandil can be attributed to its intrinsic NO-donating effects. For ZD0947, the increase in NO levels may result from its ability to open endothelial membrane KATP channels under hyperkalemic depolarization conditions, facilitating enhanced potassium efflux. This process leads to membrane hyperpolarization and subsequent NO release.
Our study is the first to demonstrate ZD0947’s ability to rapidly increase NO production immediately after reperfusion. This effect may help prevent microvascular dysfunction during cardioplegia administration and minimize inadequate myocardial perfusion. Previous research has shown that CPG reperfusion following hypothermia exacerbated arteriolar constriction and reduced capillary density (28). Further investigation in an animal model is warranted to evaluate the potential of nicorandil and ZD0947 as candidates for "hot shot" interventions before aortic declamping.
Our findings suggest that IR combined with CPG is more detrimental to endothelial cells than cardioplegia or IR alone. An endothelial KCO demonstrated protective effects when administered before reperfusion, both in the short and long term. However, the roles of KCOs in these processes, including the impact of IR on endothelial functions, the mechanisms underlying endothelial cell death, and the interplay between endothelial and myocardial functions, warrant further investigation. This data will be fundamental for refining the strategy and components of cardioplegia solutions to achieve better preservation of both the heart and endothelial cells.
This study has certain limitations, including the use of a simulated ischemic solution to induce metabolic deprivation in cells rather than employing a low oxygen level in the cell culture system. However, this model is a well-established and widely accepted IR model. Additionally, we did not incorporate intermittent perfusion of the cardioplegic solution, which more closely resembles the current techniques used in cardiac surgery.
5.1. Conclusions
Cardioplegia alone was able to preserve endothelial cells. However, CPG under IRI conditions resulted in greater cell death compared with IRI alone. Both in the presence and absence of high-potassium cardioplegia, nicorandil and ZD0947 were effective in preserving endothelial cell viability in response to IRI over both short and long durations. Nicorandil and ZD0947 also significantly increased NO production during the early stages of reperfusion.
References
-
1.
Li YZ, Wu XD, Liu XH, Li PF. Mitophagy imbalance in cardiomyocyte ischaemia/reperfusion injury. Acta Physiol (Oxf). 2019;225(4). e13228. [PubMed ID: 30507035]. https://doi.org/10.1111/apha.13228.
-
2.
Zammert M, Gelman S. The pathophysiology of aortic cross-clamping. Best Pract Res Clin Anaesthesiol. 2016;30(3):257-69. [PubMed ID: 27650338]. https://doi.org/10.1016/j.bpa.2016.07.006.
-
3.
Heusch G. Myocardial ischaemia-reperfusion injury and cardioprotection in perspective. Nat Rev Cardiol. 2020;17(12):773-89. [PubMed ID: 32620851]. https://doi.org/10.1038/s41569-020-0403-y.
-
4.
Herrera-Zelada N, Zuniga-Cuevas U, Ramirez-Reyes A, Lavandero S, Riquelme JA. Targeting the Endothelium to Achieve Cardioprotection. Front Pharmacol. 2021;12:636134. [PubMed ID: 33603675]. [PubMed Central ID: PMC7884828]. https://doi.org/10.3389/fphar.2021.636134.
-
5.
Chen G, Xu C, Gillette TG, Huang T, Huang P, Li Q, et al. Cardiomyocyte-derived small extracellular vesicles can signal eNOS activation in cardiac microvascular endothelial cells to protect against Ischemia/Reperfusion injury. Theranostics. 2020;10(25):11754-74. [PubMed ID: 33052245]. [PubMed Central ID: PMC7546010]. https://doi.org/10.7150/thno.43163.
-
6.
Riquelme JA, Westermeier F, Hall AR, Vicencio JM, Pedrozo Z, Ibacache M, et al. Dexmedetomidine protects the heart against ischemia-reperfusion injury by an endothelial eNOS/NO dependent mechanism. Pharmacol Res. 2016;103:318-27. [PubMed ID: 26607864]. https://doi.org/10.1016/j.phrs.2015.11.004.
-
7.
Yang Q, Yu CM, He GW, Underwood MJ. Protection of coronary endothelial function during cardiac surgery: potential of targeting endothelial ion channels in cardioprotection. Biomed Res Int. 2014;2014:324364. [PubMed ID: 25126553]. [PubMed Central ID: PMC4122001]. https://doi.org/10.1155/2014/324364.
-
8.
Foster MN, Coetzee WA. KATP Channels in the Cardiovascular System. Physiol Rev. 2016;96(1):177-252. [PubMed ID: 26660852]. [PubMed Central ID: PMC4698399]. https://doi.org/10.1152/physrev.00003.2015.
-
9.
Tinker A, Aziz Q, Thomas A. The role of ATP-sensitive potassium channels in cellular function and protection in the cardiovascular system. Br J Pharmacol. 2014;171(1):12-23. [PubMed ID: 24102106]. [PubMed Central ID: PMC3874693]. https://doi.org/10.1111/bph.12407.
-
10.
Aziz Q, Li Y, Tinker A. Endothelial biology and ATP-sensitive potassium channels. Channels (Austin). 2018;12(1):45-6. [PubMed ID: 29219667]. [PubMed Central ID: PMC5774186]. https://doi.org/10.1080/19336950.2017.1412151.
-
11.
Aziz Q, Li Y, Anderson N, Ojake L, Tsisanova E, Tinker A. Molecular and functional characterization of the endothelial ATP-sensitive potassium channel. J Biol Chem. 2017;292(43):17587-97. [PubMed ID: 28893911]. [PubMed Central ID: PMC5663864]. https://doi.org/10.1074/jbc.M117.810325.
-
12.
Gao S, Long CL, Wang RH, Wang H. K(ATP) activation prevents progression of cardiac hypertrophy to failure induced by pressure overload via protecting endothelial function. Cardiovasc Res. 2009;83(3):444-56. [PubMed ID: 19304734]. https://doi.org/10.1093/cvr/cvp099.
-
13.
Bondarenko OI. [Effect of adenosine triphosphate-dependent potassium channel activators on electrical reactions of intact and cultured endothelial cells]. Fiziol Zh (1994). 2009;55(1):49-56. [PubMed ID: 19441715].
-
14.
Wang H, Zuo X, Wang Q, Yu Y, Xie L, Wang H, et al. Nicorandil inhibits hypoxia-induced apoptosis in human pulmonary artery endothelial cells through activation of mitoKATP and regulation of eNOS and the NF-kappaB pathway. Int J Mol Med. 2013;32(1):187-94. [PubMed ID: 23670355]. https://doi.org/10.3892/ijmm.2013.1379.
-
15.
Dyhring T, Jansen-Olesen I, Christophersen P, Olesen J. Pharmacological Profiling of K(ATP) Channel Modulators: An Outlook for New Treatment Opportunities for Migraine. Pharmaceuticals (Basel). 2023;16(2). [PubMed ID: 37259373]. [PubMed Central ID: PMC9966414]. https://doi.org/10.3390/ph16020225.
-
16.
Wang H, Long C, Duan Z, Shi C, Jia G, Zhang Y. A new ATP-sensitive potassium channel opener protects endothelial function in cultured aortic endothelial cells. Cardiovasc Res. 2007;73(3):497-503. [PubMed ID: 17116295]. https://doi.org/10.1016/j.cardiores.2006.10.007.
-
17.
Lopez JR, Jahangir R, Jahangir A, Shen WK, Terzic A. Potassium channel openers prevent potassium-induced calcium loading of cardiac cells: possible implications in cardioplegia. J Thorac Cardiovasc Surg. 1996;112(3):820-31. [PubMed ID: 8800173]. https://doi.org/10.1016/S0022-5223(96)70070-3.
-
18.
Yang Q, Zhang RZ, Yim AP, He GW. Release of nitric oxide and endothelium-derived hyperpolarizing factor (EDHF) in porcine coronary arteries exposed to hyperkalemia: effect of nicorandil. Ann Thorac Surg. 2005;79(6):2065-71. [PubMed ID: 15919311]. https://doi.org/10.1016/j.athoracsur.2004.11.027.
-
19.
Von Oppell U, Ulrich O, Pfeiffer S, Preiss P, Boehm D, Dunne T, et al. Endothelial cell cytotoxicity of cardioplegic solutions used in South Africa. Cardiovascular J Africa. 1990;1(4):182-8.
-
20.
Evora PR, Pearson PJ, Schaff HV. Crystalloid cardioplegia and hypothermia do not impair endothelium-dependent relaxation or damage vascular smooth muscle of epicardial coronary arteries. J Thorac Cardiovasc Surg. 1992;104(5):1365-74. [PubMed ID: 1434718].
-
21.
Scarabelli T, Stephanou A, Rayment N, Pasini E, Comini L, Curello S, et al. Apoptosis of endothelial cells precedes myocyte cell apoptosis in ischemia/reperfusion injury. Circulation. 2001;104(3):253-6. [PubMed ID: 11457740]. https://doi.org/10.1161/01.cir.104.3.253.
-
22.
Zhu T, Yao Q, Wang W, Yao H, Chao J. iNOS Induces Vascular Endothelial Cell Migration and Apoptosis Via Autophagy in Ischemia/Reperfusion Injury. Cell Physiol Biochem. 2016;38(4):1575-88. [PubMed ID: 27082814]. https://doi.org/10.1159/000443098.
-
23.
Wu Y, He MY, Ye JK, Ma SY, Huang W, Wei YY, et al. Activation of ATP-sensitive potassium channels facilitates the function of human endothelial colony-forming cells via Ca(2+) /Akt/eNOS pathway. J Cell Mol Med. 2017;21(3):609-20. [PubMed ID: 27709781]. [PubMed Central ID: PMC5323860]. https://doi.org/10.1111/jcmm.13006.
-
24.
Madadi F, Aghajani M, Dabbagh A, Fani K, Sehati F, Imani A. Effects of Acute Potassium Chloride Administration on Ventricular Dysrhythmias after Myocardial Infarction in a Rat Model of Ischemia/Reperfusion. J Tehran Heart Cent. 2022;17(1):15-21. [PubMed ID: 36304768]. [PubMed Central ID: PMC9551260]. https://doi.org/10.18502/jthc.v17i1.9320.
-
25.
Gunaydin S, Akbay E, Gunertem OE, McCusker K, Onur MA, Ozisik K. Long-Term Protective Effects of Single-Dose Cardioplegic Solutions in Cell Culture Models. J Extra Corpor Technol. 2020;52(4):279-88. [PubMed ID: 33343030]. [PubMed Central ID: PMC7728504]. https://doi.org/10.1182/ject-2000028.
-
26.
Passov A, Schramko A, Salminen US, Aittomaki J, Andersson S, Pesonen E. Endothelial glycocalyx during early reperfusion in patients undergoing cardiac surgery. PLoS One. 2021;16(5). e0251747. [PubMed ID: 33999952]. [PubMed Central ID: PMC8128269]. https://doi.org/10.1371/journal.pone.0251747.
-
27.
Ferrero JM, Gonzalez-Ascaso A, Matas JFR. The mechanisms of potassium loss in acute myocardial ischemia: New insights from computational simulations. Front Physiol. 2023;14:1074160. [PubMed ID: 36923288]. [PubMed Central ID: PMC10009276]. https://doi.org/10.3389/fphys.2023.1074160.
-
28.
Keller MW, Geddes L, Spotnitz W, Kaul S, Duling BR. Microcirculatory dysfunction following perfusion with hyperkalemic, hypothermic, cardioplegic solutions and blood reperfusion. Effects of adenosine. Circulation. 1991;84(6):2485-94. [PubMed ID: 1659955]. https://doi.org/10.1161/01.cir.84.6.2485.