Abstract
Context:
Bardet-Biedl syndrome is a rare genetic disorder with variable prevalence rates across populations, characterized by symptoms such as retinal degeneration and intellectual disability. In this study, researchers investigated renal cystic epithelia from patients with PKD1 mutations. This study identified the upregulation of genes related to the Jak-STAT pathway and NF-κB signaling in these renal cells. These pathways appear to be crucial in regulating immune responses within cystic epithelial and renal cell types in PKD-affected kidneys.Evidence Acquisition:
This study was carried out through a literature search with the keywords of polycystic kidney disease (PKD), Newborn, and Bardet-Biedl syndrome (BBS), combined with Drug Therapy in Scopes, PubMed, and Web of Science. This study included relevant articles (i.e., randomized controlled trials, observational studies, guidelines, and reviews) written in English and published between 2000 and 2023.Results:
Recent genome-wide expression analyses have provided valuable insights into the molecular mechanisms associated with PKD. The Jak-STAT system, essential for immune signaling, can be activated by cytokines, such as interleukin 6 (IL-6) and interferon-gamma (IFN-γ).Conclusions:
Promising developments in the treatment of PKD have emerged from studies involving immune-modulating drugs in animal models. Glucocorticoids and rosmarinic acid exhibited positive effects, reducing cystic indices and preserving renal function in PKD mice and rats. Mycophenolate mofetil, an immunosuppressive drug, showed effectiveness in reducing cyst area, inflammation, and fibrosis in rat models. Additionally, COX-2 inhibitors, PPARγ agonists, and vasopressin V2 receptor antagonists demonstrated potential in slowing cystic disease progression.Keywords
1. Context
Bardet-Biedl syndrome (BBS) is an uncommon hereditary condition that can be found in various populations with different estimated prevalence rates. In northern Europeans, the prevalence is approximately 1 in 160,000 individuals; however, in the Bedouin population of Kuwait (1), it is higher at around 1 in 13,500 individuals. The syndrome’s discovery has led to an increased interest in studying its diverse phenotypes, which include birth defects and metabolic disorders. The underlying cause of BBS can be traced back to Mutations in both alleles of a single gene. Additionally, the presence of a third allele on a second locus can contribute to variations in the phenotypes’ penetrance (the proportion of individuals with a specific genotype who express the associated trait) and expressivity (the extent to which the trait is expressed) within and between families. Bardet-Biedl syndrome primarily arises from basal body/cilium dysfunction at the cellular level. This places BBS in a group of related disorders known as ciliopathies, which are characterized by abnormalities in the structure or function of BBS, mainly associated with the malfunction of the primary cilium, which is a small, hair-like structure located on the surface of numerous cells (2). Ciliopathies can affect various organ systems and lead to a wide range of symptoms and complications.
Bardet-Biedl syndrome represents a multifaceted condition characterized by various traits, including obesity, kidney problems, eye complications, extra digits, intellectual disability, and male infertility. It is primarily associated with issues in the primary cilium, an organelle found in many cell types except immune cells (3), crucial for multiple cellular processes by accumulating specific receptors and regulating diverse signals. Approximately 24 genes contribute to BBS, most related to the BBSome, a group of proteins believed to control the removal of specific proteins from the cilium. Although the evidence suggests potential immune system involvement in BBS, the understanding remains constrained due to the assumed absence of the cilium in immune cells. Tsyklauri et al. established a correlation between the primary cilium and immune functions by identifying a higher prevalence of autoimmune disorders, such as rheumatoid arthritis and diabetes type I, among BBS patients (4). However, the impact of obesity, prevalent in both BBS and autoimmune diseases, complicates the interpretation of these findings. Larger studies are necessary to comprehend obesity’s role in the increased occurrence of autoimmune diseases among BBS patients.
Recent research emphasizes potential connections between cilia and immune issues. Components shared by the T-cell immunological synapse and the primary cilium might affect T-cell signaling if they malfunction. Moreover, the BBSome, involved in morphogen pathways, such as Shh and Wnt (5), might influence T-cell development (Figure 1). Further investigations on mice lacking Bbs4, a BBSome component, revealed mild disruptions in B-cell development, with notable changes in developing and mature B-cell populations. However, no apparent differences in T-cell populations were observed between mutant and wild-type mice lacking Bbs4. The hindrances in B-cell development could originate from issues in non-ciliated hematopoietic cells or non-hematopoietic cells, potentially impacting the stromal microenvironment. Conditional Bbs4 knockout mice lacking Bbs4 in all hematopoietic lineages did not show broad abnormalities in B-cell development (6), suggesting that the differentiation block might stem from non-hematopoietic cells. To exclude the influence of obesity, two BBS mouse models were studied, both demonstrating comparable B-cell development issues. This finding implies that observed effects were not solely attributable to obesity. Despite some shared characteristics between these models, such as the absence of sperm flagella, other traits, such as obesity and severe preweaning lethality, were inconsistent (Figure 1).
The group of proteins known as the BBSome, originating from eight specific genes (BBS1, -2, -4, -5, -7, -8, -9, and -18), plays a critical role in transporting molecular cargo to the base of the primary cilium. This transportation utilizes pathways via the Golgi or recycling endosomes. Additionally, the BBSome aids in removing loaded cargo from the primary cilium through intraflagellar transport. These cargoes include essential receptors, such as sonic hedgehog (SHH), Wnt, PDGFα, and other vital signaling molecules necessary for embryonic development, cell growth, migration, and survival. Moreover, proteins linked to the primary cilium contribute significantly to regulating energy balance. Moreover, the complete expression of intact BBS10 effectively restored insulin signaling by reinstating insulin receptor tyrosine phosphorylation within BBS10C91fsX95 neurons (6). Furthermore, mutations found in BBS1 and BBS10 hindered the activation of leptin-mediated p-STAT3 in hypothalamic neurons derived from induced pluripotent stem cells (iPSCs), leading to an inflammatory response.
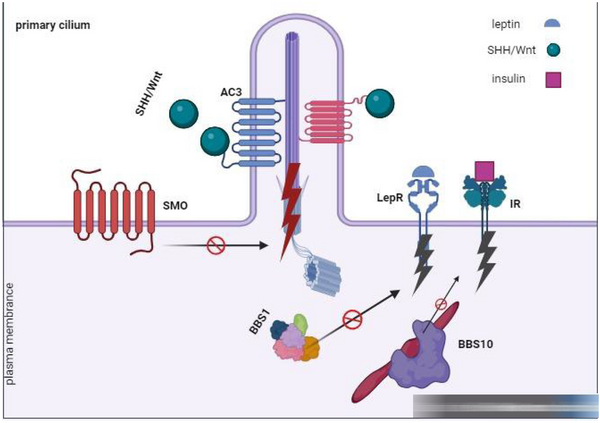
2. Evidence Acquisition
The review analyzed articles regarding pharmaceutical approaches for managing inflammation in individuals diagnosed with BBS. Researchers gathered these articles from global databases, such as ScienceDirect, Scopus, PubMed, Web of Science, and Medline. This study involved a comprehensive literature search for English-language articles using keywords like polycystic kidney disease (PKD), Newborn, and Bardet-Biedl syndrome (BBS). The review encompassed a range of research types, including randomized controlled trials (RCTs), cohort studies, systematic reviews, and narrative reviews.
2.1. Search Strategy
This review encompassed articles with titles or abstracts containing the following keywords: "Newborn," "Bardet-Biedl syndrome (BBS)," "neonatal," and "polycystic kidney disease." Additionally, articles with titles or abstracts mentioning "pharmacology" or "treatment" were considered. The included articles were limited to those published in English between 2000 and 2023, comprising a variety of study types, including RCTs, observational studies, guidelines, and reviews.
3. Results
This study provided a summary of critical topics concerning pharmacotherapy in children with BBS as follows:
(1) Major symptoms of BBS
(2) Role of inflammation and fibrosis in PKD
(3) Pathobiology of immune response in PKD
(4) Immune therapies for PKD
3.1. Major Symptoms of Bardet-Biedl syndrome
3.1.1. Retinal Degeneration
Retinal degeneration, specifically retinitis pigmentosa (RP), is a prominent characteristic of BBS. Bardet-Biedl syndrome manifests as an atypical pigmentary retinal dystrophy affecting both rod and cone photoreceptors. This leads to early maculopathy, and over 90% of BBS-affected children experience retinitis pigments as early as 7 - 8 years old. Clinical observations show that rod and cone electroretinography become extinguished before the appearance of black pigment in the peripheral retina. As the disease progresses, patients usually develop night blindness during their second decade of life (Figure 2), followed by a gradual decline. In advanced cases of BBS, there can be a loss of peripheral vision, and over time, central vision might also deteriorate.
Recent trials utilizing autologous bone marrow-derived stem cells to address retinal diseases, such as RP and non-exudative (dry) age-related macular degeneration (AMD), have displayed promising but temporary effects on patients' vision and life quality. Several Phase I and Phase II trials, particularly conducted at institutions such as the University of Sao Paulo and the University of California, Davis, have initially highlighted safety and potential benefits in managing RP-related macular edema and other retinal conditions (7). Although these studies showcased short-term enhancements in visual acuity and life quality, the long-term outcomes beyond a year post-treatment remain uncertain. Ongoing clinical trials in Spain, Thailand, India, and Saudi Arabia are further investigating the use of bone marrow stem cells for RP and dry AMD, signaling a growing interest in this therapeutic avenue.
Retinitis pigmentosa (RP) is one of the primary features of Bardet-Biedl syndrome (BBS), and it affects the vision of more than 90% of BBS-affected children of 7 to 8 years old. Retinitis pigmentosa is a progressive genetic eye disorder that leads to the gradual degeneration of the light-sensitive cells (rods and cones) in the retina, which are essential for vision. As the disease progresses, individuals with RP experience a gradual loss of peripheral vision, night blindness, and, in advanced cases, a decrease in central vision. Source: Steve Allen/Getty Images.
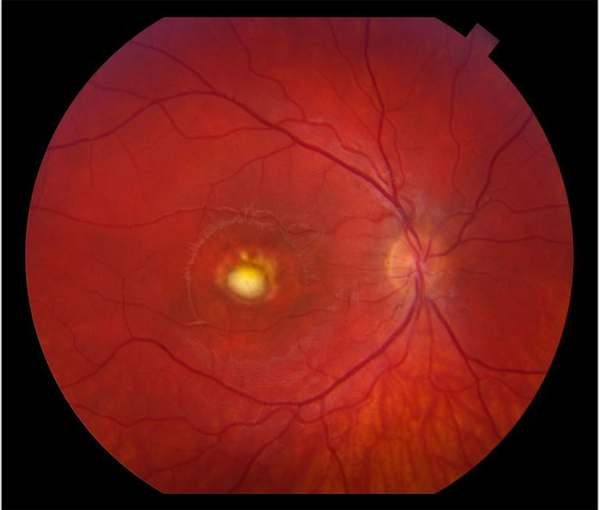
3.1.2. Intellectual Disability
Bardet-Biedl syndrome can be associated with intellectual disability, particularly during childhood. Approximately 62% of BBS patients experience significant learning disabilities during this stage of life (8). Although impaired vision might play a role in these conditions, intelligence quotient (IQ) tests have indicated deficiencies in mental faculties in approximately 40% of individuals diagnosed with BBS. This finding suggests that BBS can have an impact on cognitive abilities beyond the visual impairments it presents.
Comprehending and diagnosing cognitive disabilities is pivotal for providing tailored professional care, focusing on an individual's intellectual capacity, adaptability to their surroundings, and parental involvement. As cognitive disabilities are not curable, treatment aims at aligning behavior with societal norms. Early intervention, especially in the initial 5 years, leverages brain plasticity for optimal therapeutic results. Acknowledging the parental role in upbringing is crucial, as behavioral issues often trace back to deficient child-rearing practices. Educational objectives prioritize life skills over conventional schooling due to limitations in abstract cognitive thinking. Successful treatment relies on prompt diagnosis, considering severity, affected areas, and rehabilitation techniques employed. Interventions differ among age groups, targeting motor, sensory, linguistic, and perceptual deficiencies. Behavioral therapy, emphasizing positive reinforcement, holds significant importance. Adolescent cases (9), including those previously undiagnosed, benefit from programs fostering independent living skills and vocational training. Pharmacological interventions address comorbidities based on specific disorder criteria, with recent research pointing to improved outcomes with certain medications. The holistic approach to treating cognitive disabilities involves a collaboration among multiple disciplines, including medicine, psychology, education, and rehabilitation.
Both structural and functional renal abnormalities can be observed in individuals with BBS. However, in BBS, anatomical abnormalities are observed in around 46% of patients without the manifestation of physiological symptoms, which occurs in approximately 5% of cases. These anatomical abnormalities include fetal lobulation, calyceal clubbing or calyceal cysts, cortical scarring, and PKD. On the other hand, physiological symptoms that might occur include polyuria, polydipsia, renal tubular acidosis, anemia, and hypertension. Of significant concern, end-stage renal disease resulting from progressive renal abnormalities is the primary cause of morbidity and mortality in BBS patients. This finding highlights the critical impact that renal complications can have on the overall health and well-being of individuals with BBS (10).
Autosomal dominant polycystic kidney disease (ADPKD) commonly originates from an initial mutation in a polycystin gene, followed by a subsequent somatic alteration resulting in the loss of the normal gene, and finally, a third event that triggers cell proliferation. This sequence leads to the dilation of tubules and continued enlargement due to increased cell growth, secretion of fluid, and detachment from the original tubule, ultimately forming cysts. Studies focusing on cystic cells and ADPKD animal models emphasize the significance of calcium and cyclic adenosine monophosphate (cAMP) in the progression of this disease. Initially, mutations in PKD genes lead to decreased intracellular calcium levels, which alters cell characteristics when exposed to cAMP. Although cAMP inhibits the growth of healthy kidney epithelial cells by blocking the Ras/Raf/MEK/ERK pathway, it stimulates proliferation in ADPKD cells due to reduced intracellular calcium levels, activating the B-Raf/MEK/ERK pathway. Increased cAMP levels also affect chloride channels, leading to the secretion of fluid into cysts.
Multiple disrupted pathways contribute to cyst development and advancement, such as impaired planar cell polarity, irregularities in the extracellular matrix, inflammation, heightened apoptosis, genetic variations, and environmental factors. Understanding the molecular mechanisms behind PKD has spurred the exploration of novel therapeutic strategies (11). Studies suggest that treatments aimed at reducing cAMP, increasing intracellular calcium, inhibiting cell proliferation, and suppressing cyst fluid secretion might help alleviate cyst growth, as indicated by paraclinical investigations (Figure 3).
Polycystic kidney disease causes disruptions in calcium regulation and raises cyclic adenosine monophosphate (cAMP) within ADPKD cells due to mutations in polycystins. This leads to lowered intracellular calcium levels, activating Ca2+-inhibitable AC6 and suppressing Ca2+-dependent PDE1, ultimately increasing cAMP levels. Elevated cAMP triggers chloride channel stimulation and fluid secretion and activates MAPK/ERK signaling via Src and Ras pathways. Autosomal dominant polycystic kidney disease cells also show increased levels of EGF, IGF1, and VEGF, which stimulate tyrosine kinase receptors, leading to MAPK/ERK signaling activation and cell proliferation. Additionally, ADPKD involves interstitial inflammation through NF‐κB and JAK‐STAT pathways.
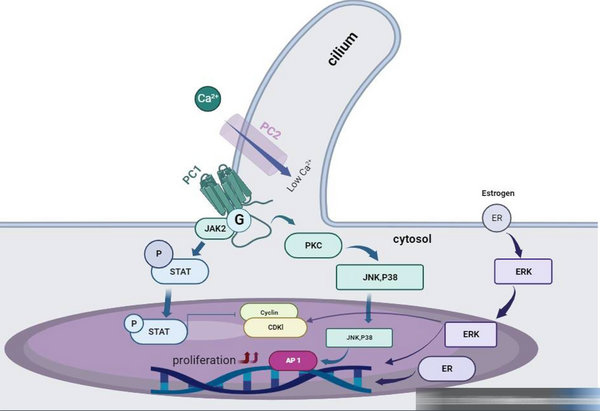
3.2. Role of Inflammation and Fibrosis in Cystic Kidney Disease
Polycystic kidney disease can be categorized into two main types: ADPKD and autosomal recessive polycystic kidney disease (ARPKD).
(1) Autosomal dominant polycystic kidney disease is the more prevalent form, often manifesting symptoms during adulthood. In most instances, the progression of cystic kidneys eventually results in end-stage renal disease. Approximately 85% of ADPKD cases are attributed to mutations in the PKD1 gene, although the remaining 15% are due to mutations in the PKD2 gene (12). Despite being dominantly inherited, ADPKD requires a "second hit" at the molecular level. A germline mutation is initially followed by a somatic mutation occurring in the second allele. The presence of homozygous mutations in PKD1 can result in a severe phenotype resembling ARPKD, which supports the concept of a second hit mutation. This hypothesis provides an explanation for the variation in cyst formation observed within families and the restricted number of affected nephrons as new mutations gradually accumulate. However, the precise factor responsible for cyst formation following the loss of the second allele remains unclear, with some studies suggesting proliferation as a consequence rather than a driving cause.
(2) Autosomal recessive polycystic kidney disease, on the other hand, is caused by mutations in the PKHD1 gene. Autosomal recessive polycystic kidney disease is characterized by its greater severity and early onset, frequently identified during prenatal screening. Sadly, many newborns with ARPKD pass away shortly after birth due to pulmonary hypoplasia. Of those who survive the neonatal period, approximately 80% manage to live beyond the age of 10 years; however, they often develop end-stage renal disease by the time they reach 15 years old (13). Additionally, ARPKD patients face hepatic issues linked to ductal plate malformation and fibrosis. Despite advances in care, the prognosis for ARPKD patients remains poor, and treatment options are limited, often requiring kidney transplantation.
Understanding the distinctions between ADPKD and ARPKD is crucial for diagnosis, management, and providing appropriate care to patients affected by these conditions. In recent times, genome-wide expression analyses have significantly contributed to gaining a comprehensive understanding of the biological processes related to PKD (14). In one study analyzing renal cystic epithelia from patients with PKD1 mutations, the Jak-STAT pathway and NF-κB signaling were found to be prominently upregulated among the 100 most upregulated gene sets (15). These pathways likely play a central role in modulating the immune responses of cystic epithelial cells and other renal cell types found in PKD kidneys, including endothelial cells, fibroblasts, and myeloid lineage cells.
The Jak-STAT system is crucial for immune signaling, and it is activated by a variety of cytokines and growth factors, one of which is interleukin 6 (IL-6) and interferon-gamma (IFN-γ) (16). Mutated polycystin-1 and polycystin-2 proteins, associated with PKD, activate Jak1 and Jak2, which in turn regulate the phosphorylation of STAT proteins. Consequently, abnormal Jak-STAT signaling can lead to immunomodulatory effects and might represent one of the initial alterations induced by PKD mutations. NF-κB protein complexes are essential regulators of gene transcription involved in inflammation (17), growth, and apoptosis. In PKD, NF-κB activation has been observed in various studies. In Pkd1-deficient cells, increased NF-κB activity was shown, along with phosphorylated NK-κB found. In cyst-derived epithelial cell nuclei and tubules surrounding cysts, the presence of these pathways has been observed both in mutant Pkd2 mice and human ADPKD kidneys. Inhibiting NF-κB activity in animal PKD models resulted in reduced renal cystic indices, suggesting its involvement in PKD-associated immune responses.
The precise mechanisms responsible for the effects of NF-κB inhibition are not yet fully understood. However, it is possible that NF-κB inhibition could influence renal development or repair processes, as evidenced by the downregulation of nephron development regulators and planar cell polarity regulators in the context of Pkd2 deficiency (18). NF-κB might also play a role in interactions with primary apical cilia, further emphasizing its significance in PKD-related immune responses. Understanding these molecular pathways sheds light on potential therapeutic targets for PKD and helps elucidate the intricate mechanisms underlying this complex kidney disorder. Renal interstitial inflammatory infiltrates are prominent features of PKD, with macrophages being the most extensively studied cell type among these infiltrating inflammatory cells (19). Nevertheless, it is crucial to acknowledge that macrophages do not constitute a homogenous cell group but rather highly heterogeneous, with specific roles in the pathobiology of PKD progression. One type of macrophages, yolk sac-derived resident macrophages, might be involved in the early stages of renal cystic disease and play critical supportive roles in renal tissue, similar to the functions of microglia in the brain. These resident renal macrophages have been shown to be vital for ureteric bud branching during kidney development and are believed to monitor surrounding cells for injury after tissue damage.
Another distinct population of renal macrophages is derived from bone marrow. In humans, macrophages are characterized by the absence of CD16 expression; however, in mice, they are identified as F4/80low and Cd11bhigh (Myb-dependent population) (20). Following an injury, such as renal ischemia-reperfusion injury (IRI), these macrophages, or their earlier stage of differentiation known as monocytes, infiltrate renal tissue. At the outset, they undergo differentiation into inflammatory macrophages. As the cystic kidney experiences increasing injury-like responses over time, these macrophages might play more pronounced roles in disease progression during the advanced stages of PKD. In addition to distinguishing macrophage populations based on their origin (yolk sac-derived resident vs. bone marrow-derived infiltrating macrophages), they can also be categorized according to their "activation" pathway. This classification helps characterize the functional states and roles of macrophages in different physiological and pathological conditions.
Ly6Chigh macrophages are linked to the "classic" macrophage activation pathway, often designated as M1 activation (21). These macrophages express IL-1β and Cxcl2 and are typically associated with IFN-γ activation and the recognition of pathogens through pattern recognition receptors, such as the CD14-TLR4 complex that recognizes lipopolysaccharide (LPS). On the other hand, Ly6Clow macrophages exhibit gene expression traits similar to the "alternative" or M2 macrophage activation pathway (22). The classification of the M2 pathway is intricate and likely involves multiple M2 macrophage subtypes. Nevertheless, it is believed that at least a subset of M2 macrophages can downregulate the production of pro-inflammatory cytokines and contribute to tissue repair and fibrosis. The Ly6Chigh monocytes, a major infiltrating cell subtype, are responsible for inducing injury after events such as IRI and unilateral ureteric obstruction (UUO) (23). The impact of these infiltrating cells is further enhanced by phenotypic and physiological changes they activate in macrophages and other mononuclear phagocytes that are already present in renal tissue.
Although M1 macrophages are associated with inflammation and phagocytosis, M2 macrophages are believed to play a role in tissue repair and modulation of the immune response. Additionally, there is another model suggesting that M1 macrophages are involved in the clearance of necrotic or apoptotic cells, and functions of M1 and M2 macrophages provide valuable insights into the complex immune responses involved in PKD progression. In the context of PKD (24), macrophages play a significant role in the progression of the disease and the associated inflammation. Macrophages can exhibit different activation states, including M1 and M2, which have distinct functions in the immune response. In PKD, M1 macrophages can be involved in the phagocytosis of dying or damaged cells. After engulfing these cells, they transform into M2-like macrophages. M2 macrophages secrete anti-inflammatory cytokines that aid in resolving inflammation and promoting tissue healing (25).
Macrophages have been observed in the renal interstitium during both the early and late stages of ADPKD and ARPKD. In certain cases, macrophage recruitment might be linked to cystic kidney infection. Their presence in patients without infections suggests that macrophage accumulation is an indicator of an underlying inflammatory process. The presence of macrophages appears to be an intrinsic feature of PKD, indicating that their role extends beyond being solely an antimicrobial response. In PKD, the predominant type of interstitial macrophages is the alternatively activated M2 type. This type of macrophage response was first identified in the Cys1cpk mouse model of ARPKD and subsequently confirmed in other relevant experimental models. The orthologous Pkd1fl/fl Pkhd1-Cre mouse model is used in PKD research. The depletion of these macrophages in PKD animal models has been shown to improve cystic indices and renal function (26), suggesting that M2 macrophages might promote cystic disease progression by stimulating cystic epithelial cell proliferation.
3.3. Monocyte Chemoattractant Protein-1
Monocyte chemoattractant protein-1 (MCP1) is a chemokine that plays a crucial role in facilitating the recruitment of monocytes and other inflammatory responses. Indeed, MCP-1 is responsible for recruiting various cell types, including monocytes, tubular epithelial cells, and different types of macrophages in the interstitial space, secreting MCP-1. Monocyte chemoattractant protein-1 has been implicated in the pathogenesis of numerous renal diseases and has been identified as a potential biomarker for acute kidney injury. In the context of ADPKD (27), studies have shown that renal cyst fluid contains higher levels of MCP-1 than urine. Furthermore, elevated MCP-1 levels were associated with worse renal function and higher height-adjusted total kidney volume (TKV) (28) and the presence of the PKD1 genotype over the PKD2 genotype. Studies have demonstrated a strong association between elevated MCP-1 levels and declining glomerular filtration rate (GFR) serves as a predictor of stage 3 CKD in ADPKD patients. Therefore, urinary MCP-1 might be considered a potential marker for disease progression in individuals with ADPKD.
3.4. Tumor Necrosis Factor-Alpha
Tumor necrosis factor-alpha (TNF-α) is a well-known immune cytokine that plays a significant role in activating inflammatory signaling and has important functions in various biological processes and clinical conditions. In the context of PKD, TNF-α has been implicated in the disease's pathogenesis. One way it affects PKD is by interfering with the processing and membrane presentation of polycystin-2, a protein involved in PKD development (29). In ADPKD, TNF-α has been detected in renal cysts, with concentrations similar to those found in synovial fluid in psoriatic arthritis. The intracystic levels of TNF-α correlated with the size of renal cysts. Experiments have demonstrated that TNF-α can promote cystogenesis in a co-culture of Pkd2−/− and wild-type embryonic kidney explants. Moreover, intraperitoneal administration of TNF-α increased the severity of renal cystic disease in Pkd2−/− mice. Importantly, when TNF-α was inhibited using etanercept, a TNF-α blocker, cystogenesis was attenuated in Pkd2−/− mice. However, this treatment was not effective in animal models with established cystic kidney disease, such as the Pkhd1PCK rat and PKD2 (ws25/w183) mouse (30). The aforementioned findings indicate that TNF-α plays a significant role in promoting cystogenesis during the early stages of kidney disease of PKD development. Inhibiting TNF-α signaling could be a potential therapeutic approach to slow the progression of PKD. However, the effectiveness of such treatments might vary depending on the disease stage and other factors, warranting further investigation and clinical studies.
3.5. Pathobiology of Immune Response in PKD
Immune changes associated with PKD were believed to be consequences of advanced renal cystic disease. Mainly affecting the later stages of disease progression, the presence of inflammatory infiltrates observed during these advanced phases coincided with the concurrent development of severe interstitial fibrosis and cyst formation. However, studies have shown that immune responses play significant roles in the progression of renal cystic disease, with functional relevance. from the early stages, young mice experiencing a fast progression of renal cystic disease, similar to what is observed in the Cys1cpk mouse model of ARPKD, showed the overexpression of various innate immune factors. This finding suggested that abnormal immune responses might contribute to the development and worsening of cystogenesis. Additionally, this rapid pace of renal cystic disease progression in juvenile mice might contribute to adverse alterations in the extracellular matrix and facilitate the development of interstitial fibrosis.
Macrophages in PKD kidneys have been shown to express markers of pro-fibrotic activity (31), indicating their potential involvement in fibrosis development. Furthermore, immune responses might affect the primary cilia and primary cilia-associated proteins, which play essential roles in PKD. For instance, exposure to certain cytokines, such as IL-1, can lead to cilia elongation and alter the production of prostanoids with immunomodulatory properties. Tumor necrosis factor-alpha treatment has been found to disrupt the interaction between polycystin-1 and polycystin-2, which are vital proteins for ciliary function and mechanosensory responses. These alterations might potentially enhance cystogenesis. Although immune responses in PKD (28) can have adverse effects on disease progression, they might also have restorative potential. Some immune cell populations associated with PKD have been linked to protective pro-angiogenic factors and anti-inflammatory markers in other types of injuries, suggesting they might play a role in repair processes. However, the chronic activation of these responses in the context of PKD can contribute to sustained inflammation and further exacerbate the progression of the disease. This accelerated progression of renal cystic disease in juvenile mice might lead to "futile" repair (32), which further worsens the disease by promoting increased cyst formation and interstitial fibrosis. In summary, immune responses in PKD are complex and can have both detrimental and potentially restorative effects on disease progression. Further research is required to gain a deeper understanding of these interactions and to identify potential therapeutic targets for managing PKD more effectively.
Currently, there is no Food and Drug Administration (FDA)-approved therapy specifically designed for the treatment of PKD. Although several drugs have been assessed as potential PKD therapeutics in clinical studies, further research is needed to establish effective treatments. Most treatments have not shown substantial efficacy in slowing disease progression. One potential therapeutic option for PKD is the vasopressin receptor 2 antagonist tolvaptan (33), which is currently undergoing advanced phase 3b clinical testing.
3.6. Immune Therapies for PKD
Some drugs with immune-modulating properties have shown promise in animal models of PKD. In mice with PKD, the administration of glucocorticoids resulted in reduced cystic indices, improved renal function, and decreased infiltration of monocytes in the interstitial areas. Additionally, rosmarinic acid, which covalently binds to the C3 complement (34), demonstrated a reduction in cystic indices and slowed the loss of renal function in both PKD mice and rat models. Mycophenolate mofetil, an immunosuppressive drug, has been explored in the context of PKD. In rat models of PKD, certain drugs with immune-modulating properties, such as COX-2 inhibitors and peroxisome proliferator-activated receptor gamma (PPARγ) agonists (35), have shown promising effects. They reduced cyst area, improved renal function, and decreased inflammation and fibrosis (36). Moreover, vasopressin V2 receptor antagonists have also shown a potential to inhibit cystic disease progression. However, translating these promising results from animal models to human patients has been challenging. Differences in pharmacokinetics, drug doses used in animal models, and several factors could potentially contribute to variations in immune responses between rodents and humans, from differences in their immune systems to the lack of success in clinical trials. For instance, drugs that demonstrated positive effects in animal models include angiotensin receptor blockers and mTOR inhibitors (37). Although immune function-modulating drugs show promise as potential therapeutics, they did not result in significant changes in disease progression during clinical testing for PKD. Further research and critical evaluation are needed before conducting clinical trials. Understanding the specific immune pathways involved in PKD and identifying drugs that target those pathways effectively in humans will be crucial for the development of successful therapies for this condition.
Recent genome-wide expression analyses have provided a comprehensive understanding of the biological processes linked to PKD. One study examined renal cystic epithelia from patients with PKD1 mutations and found prominent upregulation of the Jak-STAT pathway and NF-κB signaling among the 100 most upregulated gene sets (38). The identified pathways are likely to play a central role in modulating immune responses in different renal cell types found in PKD kidneys, including endothelial cells, fibroblasts, and myeloid lineage cells. The Jak-STAT system is crucial for immune signaling, and it is activated by various cytokines and growth factors, including IL-6 and IFN-γ (39). Mutated polycystin-1 and polycystin-2 proteins associated with PKD activate Jak1 and Jak2, which, in turn, regulate the phosphorylation of STAT proteins. Consequently, abnormal Jak-STAT signaling can lead to immunomodulatory effects and might represent one of the initial alterations induced by PKD mutations (40). Understanding these molecular pathways sheds light on potential therapeutic targets for PKD and helps elucidate the intricate mechanisms underlying this complex kidney disorder. Renal interstitial inflammatory infiltrates are prominent features of PKD, with macrophages being the most extensively studied cell type among these infiltrating inflammatory cells. However, it is essential to note that macrophages are not a uniform cell population but rather highly heterogeneous, with specific roles in the pathobiology of PKD progression.
4. Conclusions
Several drugs with immune-modulating properties have displayed promising results in animal models of PKD. For instance, glucocorticoids administered to mice with PKD resulted in reduced inflammation and improved renal function. Furthermore, the administration of rosmarinic acid, which binds to the C3 complement, resulted in reduced cystic indices, improved renal function, and decreased infiltration of monocytes and exhibited positive effects by reducing cystic indices and slowing down renal function loss in both PKD mouse and rat models. Mycophenolate mofetil, an immunosuppressive drug, was also found to be effective in reducing cyst area, improving renal function, and decreasing inflammation and fibrosis in rat models of PKD. Furthermore, other drugs include COX-2 inhibitors, PPARγ agonists, and vasopressin V2 receptor antagonists. Antagonists have shown the potential to inhibit the progression of cystic disease. Despite these promising results in animal studies, translating them to human patients has proven to be challenging. Factors such as differences in drug pharmacokinetics, varying drug doses used in animal models, and discrepancies in immune responses between rodents and humans have contributed to the lack of success in clinical trials. For instance, drugs that exhibited positive effects in animal models, such as angiotensin receptor blockers and mTOR inhibitors, did not produce significant changes in disease progression during clinical testing. As a result, although drugs with immune-modulating properties hold promise as potential treatments for PKD, further research and critical evaluation are necessary before conducting clinical trials. Understanding the specific immune pathways involved in PKD and identifying drugs that can effectively target those pathways in humans will be crucial for the development of successful therapies for this condition. Through continued dedication to research and collaboration between scientists and clinicians, there is hope for discovering effective immune-modulating treatments for PKD in the future.
4.1. Limitations
This review had some limitations, including the uncertainty in assigning evidence levels and recommendation grades (grades A-D) due to variations in article design. Additionally, the review faced constraints related to the limited availability of cohort and trial articles.
References
-
1.
Beales PL, Warner AM, Hitman GA, Thakker R, Flinter FA. Bardet-Biedl syndrome: a molecular and phenotypic study of 18 families. J Med Genet. 1997;34(2):92-8. [PubMed ID: 9039982]. [PubMed Central ID: PMC1050859]. https://doi.org/10.1136/jmg.34.2.92.
-
2.
Green JS, Parfrey PS, Harnett JD, Farid NR, Cramer BC, Johnson G, et al. The cardinal manifestations of Bardet-Biedl syndrome, a form of Laurence-Moon-Biedl syndrome. N Engl J Med. 1989;321(15):1002-9. [PubMed ID: 2779627]. https://doi.org/10.1056/NEJM198910123211503.
-
3.
Nachury MV, Loktev AV, Zhang Q, Westlake CJ, Peranen J, Merdes A, et al. A core complex of BBS proteins cooperates with the GTPase Rab8 to promote ciliary membrane biogenesis. Cell. 2007;129(6):1201-13. [PubMed ID: 17574030]. https://doi.org/10.1016/j.cell.2007.03.053.
-
4.
Tsyklauri O, Niederlova V, Forsythe E, Prasai A, Drobek A, Kasparek P, et al. Bardet-Biedl Syndrome ciliopathy is linked to altered hematopoiesis and dysregulated self-tolerance. EMBO Rep. 2021;22(2). e50785. [PubMed ID: 33426789]. [PubMed Central ID: PMC7857422]. https://doi.org/10.15252/embr.202050785.
-
5.
Tummala P, Arnsdorf EJ, Jacobs CR. The Role of Primary Cilia in Mesenchymal Stem Cell Differentiation: A Pivotal Switch in Guiding Lineage Commitment. Cell Mol Bioeng. 2010;3(3):207-12. [PubMed ID: 20823950]. [PubMed Central ID: PMC2930791]. https://doi.org/10.1007/s12195-010-0127-x.
-
6.
Cassioli C, Baldari CT. A Ciliary View of the Immunological Synapse. Cells. 2019;8(8). [PubMed ID: 31362462]. [PubMed Central ID: PMC6721628]. https://doi.org/10.3390/cells8080789.
-
7.
Park SS, Bauer G, Abedi M, Pontow S, Panorgias A, Jonnal R, et al. Intravitreal autologous bone marrow CD34+ cell therapy for ischemic and degenerative retinal disorders: preliminary phase 1 clinical trial findings. Invest Ophthalmol Vis Sci. 2014;56(1):81-9. [PubMed ID: 25491299]. [PubMed Central ID: PMC4288143]. https://doi.org/10.1167/iovs.14-15415.
-
8.
Katsanis N, Ansley SJ, Badano JL, Eichers ER, Lewis RA, Hoskins BE, et al. Triallelic inheritance in Bardet-Biedl syndrome, a Mendelian recessive disorder. Science. 2001;293(5538):2256-9. [PubMed ID: 11567139]. https://doi.org/10.1126/science.1063525.
-
9.
Ring H, Zia A, Lindeman S, Himlok K. Interactions between seizure frequency, psychopathology, and severity of intellectual disability in a population with epilepsy and a learning disability. Epilepsy Behav. 2007;11(1):92-7. [PubMed ID: 17521964]. https://doi.org/10.1016/j.yebeh.2007.04.002.
-
10.
Badano JL, Kim JC, Hoskins BE, Lewis RA, Ansley SJ, Cutler DJ, et al. Heterozygous mutations in BBS1, BBS2 and BBS6 have a potential epistatic effect on Bardet-Biedl patients with two mutations at a second BBS locus. Hum Mol Genet. 2003;12(14):1651-9. [PubMed ID: 12837689]. https://doi.org/10.1093/hmg/ddg188.
-
11.
Takakura A, Nelson EA, Haque N, Humphreys BD, Zandi-Nejad K, Frank DA, et al. Pyrimethamine inhibits adult polycystic kidney disease by modulating STAT signaling pathways. Hum Mol Genet. 2011;20(21):4143-54. [PubMed ID: 21821671]. [PubMed Central ID: PMC3188991]. https://doi.org/10.1093/hmg/ddr338.
-
12.
Cowley BJ, Ricardo SD, Nagao S, Diamond JR. Increased renal expression of monocyte chemoattractant protein-1 and osteopontin in ADPKD in rats. Kidney Int. 2001;60(6):2087-96. [PubMed ID: 11737583]. https://doi.org/10.1046/j.1523-1755.2001.00065.x.
-
13.
Zheng D, Wolfe M, Cowley BJ, Wallace DP, Yamaguchi T, Grantham JJ. Urinary excretion of monocyte chemoattractant protein-1 in autosomal dominant polycystic kidney disease. J Am Soc Nephrol. 2003;14(10):2588-95. [PubMed ID: 14514736]. https://doi.org/10.1097/01.asn.0000088720.61783.19.
-
14.
Song X, Di Giovanni V, He N, Wang K, Ingram A, Rosenblum ND, et al. Systems biology of autosomal dominant polycystic kidney disease (ADPKD): computational identification of gene expression pathways and integrated regulatory networks. Hum Mol Genet. 2009;18(13):2328-43. [PubMed ID: 19346236]. https://doi.org/10.1093/hmg/ddp165.
-
15.
Schindler C, Levy DE, Decker T. JAK-STAT signaling: from interferons to cytokines. J Biol Chem. 2007;282(28):20059-63. [PubMed ID: 17502367]. https://doi.org/10.1074/jbc.R700016200.
-
16.
Park EY, Seo MJ, Park JH. Effects of specific genes activating RAGE on polycystic kidney disease. Am J Nephrol. 2010;32(2):169-78. [PubMed ID: 20606421]. https://doi.org/10.1159/000315859.
-
17.
Chevalier RL. Obstructive nephropathy: lessons from cystic kidney disease. Nephron. 2000;84(1):6-12. [PubMed ID: 10644902]. https://doi.org/10.1159/000045532.
-
18.
Torres M, Gomez-Pardo E, Dressler GR, Gruss P. Pax-2 controls multiple steps of urogenital development. Development. 1995;121(12):4057-65. [PubMed ID: 8575306]. https://doi.org/10.1242/dev.121.12.4057.
-
19.
Ta MH, Harris DC, Rangan GK. Role of interstitial inflammation in the pathogenesis of polycystic kidney disease. Nephrology (Carlton). 2013;18(5):317-30. [PubMed ID: 23448509]. https://doi.org/10.1111/nep.12045.
-
20.
Huen SC, Cantley LG. Macrophage-mediated injury and repair after ischemic kidney injury. Pediatr Nephrol. 2015;30(2):199-209. [PubMed ID: 24442822]. [PubMed Central ID: PMC5048744]. https://doi.org/10.1007/s00467-013-2726-y.
-
21.
Ruiz-Ortega M, Ruperez M, Esteban V, Rodriguez-Vita J, Sanchez-Lopez E, Carvajal G, et al. Angiotensin II: a key factor in the inflammatory and fibrotic response in kidney diseases. Nephrol Dial Transplant. 2006;21(1):16-20. [PubMed ID: 16280370]. https://doi.org/10.1093/ndt/gfi265.
-
22.
Segerer S, Nelson PJ, Schlondorff D. Chemokines, chemokine receptors, and renal disease: from basic science to pathophysiologic and therapeutic studies. J Am Soc Nephrol. 2000;11(1):152-76. [PubMed ID: 10616852]. https://doi.org/10.1681/ASN.V111152.
-
23.
Li X, Magenheimer BS, Xia S, Johnson T, Wallace DP, Calvet JP, et al. A tumor necrosis factor-alpha-mediated pathway promoting autosomal dominant polycystic kidney disease. Nat Med. 2008;14(8):863-8. [PubMed ID: 18552856]. [PubMed Central ID: PMC3359869]. https://doi.org/10.1038/nm1783.
-
24.
Fischer MB, Ma M, Goerg S, Zhou X, Xia J, Finco O, et al. Regulation of the B cell response to T-dependent antigens by classical pathway complement. J Immunol. 1996;157(2):549-56. [PubMed ID: 8752901].
-
25.
Roix J, Saha S. TNF-alpha blockade is ineffective in animal models of established polycystic kidney disease. BMC Nephrol. 2013;14:233. [PubMed ID: 24160989]. [PubMed Central ID: PMC4231369]. https://doi.org/10.1186/1471-2369-14-233.
-
26.
Hong Y, Zhou W, Li K, Sacks SH. Triptolide is a potent suppressant of C3, CD40 and B7h expression in activated human proximal tubular epithelial cells. Kidney Int. 2002;62(4):1291-300. [PubMed ID: 12234299]. https://doi.org/10.1111/j.1523-1755.2002.kid586.x.
-
27.
Chapman AB, Bost JE, Torres VE, Guay-Woodford L, Bae KT, Landsittel D, et al. Kidney volume and functional outcomes in autosomal dominant polycystic kidney disease. Clin J Am Soc Nephrol. 2012;7(3):479-86. [PubMed ID: 22344503]. [PubMed Central ID: PMC3302672]. https://doi.org/10.2215/CJN.09500911.
-
28.
Nauli SM, Alenghat FJ, Luo Y, Williams E, Vassilev P, Li X, et al. Polycystins 1 and 2 mediate mechanosensation in the primary cilium of kidney cells. Nat Genet. 2003;33(2):129-37. [PubMed ID: 12514735]. https://doi.org/10.1038/ng1076.
-
29.
Partsch G, Steiner G, Leeb BF, Dunky A, Broll H, Smolen JS. Highly increased levels of tumor necrosis factor-alpha and other proinflammatory cytokines in psoriatic arthritis synovial fluid. J Rheumatol. 1997;24(3):518-23. [PubMed ID: 9058659].
-
30.
Arnold L, Henry A, Poron F, Baba-Amer Y, van Rooijen N, Plonquet A, et al. Inflammatory monocytes recruited after skeletal muscle injury switch into antiinflammatory macrophages to support myogenesis. J Exp Med. 2007;204(5):1057-69. [PubMed ID: 17485518]. [PubMed Central ID: PMC2118577]. https://doi.org/10.1084/jem.20070075.
-
31.
Torres VE, Abebe KZ, Chapman AB, Schrier RW, Braun WE, Steinman TI, et al. Angiotensin blockade in late autosomal dominant polycystic kidney disease. N Engl J Med. 2014;371(24):2267-76. [PubMed ID: 25399731]. [PubMed Central ID: PMC4284824]. https://doi.org/10.1056/NEJMoa1402686.
-
32.
Ricardo SD, van Goor H, Eddy AA. Macrophage diversity in renal injury and repair. J Clin Invest. 2008;118(11):3522-30. [PubMed ID: 18982158]. [PubMed Central ID: PMC2575702]. https://doi.org/10.1172/JCI36150.
-
33.
Treille S, Bailly JM, Van Cauter J, Dehout F, Guillaume B. The use of lanreotide in polycystic kidney disease: a single-centre experience. Case Rep Nephrol Urol. 2014;4(1):18-24. [PubMed ID: 24707279]. [PubMed Central ID: PMC3975724]. https://doi.org/10.1159/000358268.
-
34.
Antiga L, Piccinelli M, Fasolini G, Ene-Iordache B, Ondei P, Bruno S, et al. Computed tomography evaluation of autosomal dominant polycystic kidney disease progression: a progress report. Clin J Am Soc Nephrol. 2006;1(4):754-60. [PubMed ID: 17699283]. https://doi.org/10.2215/CJN.02251205.
-
35.
Norman J. Fibrosis and progression of autosomal dominant polycystic kidney disease (ADPKD). Biochim Biophys Acta. 2011;1812(10):1327-36. [PubMed ID: 21745567]. [PubMed Central ID: PMC3166379]. https://doi.org/10.1016/j.bbadis.2011.06.012.
-
36.
Kawai T, Masaki T, Doi S, Arakawa T, Yokoyama Y, Doi T, et al. PPAR-gamma agonist attenuates renal interstitial fibrosis and inflammation through reduction of TGF-beta. Lab Invest. 2009;89(1):47-58. [PubMed ID: 19002105]. https://doi.org/10.1038/labinvest.2008.104.
-
37.
Perico N, Zoja C, Corna D, Rottoli D, Gaspari F, Haskell L, et al. V1/V2 Vasopressin receptor antagonism potentiates the renoprotection of renin-angiotensin system inhibition in rats with renal mass reduction. Kidney Int. 2009;76(9):960-7. [PubMed ID: 19625993]. https://doi.org/10.1038/ki.2009.267.
-
38.
Nelson PJ, Rees AJ, Griffin MD, Hughes J, Kurts C, Duffield J. The renal mononuclear phagocytic system. J Am Soc Nephrol. 2012;23(2):194-203. [PubMed ID: 22135312]. [PubMed Central ID: PMC3269181]. https://doi.org/10.1681/ASN.2011070680.
-
39.
Lim AK, Tesch GH. Inflammation in diabetic nephropathy. Mediators Inflamm. 2012;2012:146154. [PubMed ID: 22969168]. [PubMed Central ID: PMC3432398]. https://doi.org/10.1155/2012/146154.
-
40.
Ibrahim S. Increased apoptosis and proliferative capacity are early events in cyst formation in autosomal-dominant, polycystic kidney disease. Sci World J. 2007;7:1757-67. [PubMed ID: 18040538]. [PubMed Central ID: PMC5901185]. https://doi.org/10.1100/tsw.2007.274.