Abstract
Background:
In recent years, the relationship between cancer cells and electromagnetic radiation has received much attention.Objectives:
The present study aimed to evaluate the effects of different intensities of electromagnetic fields on gastric cancer cell lines (AGS).Methods:
After preparing AGS and Hu02 (normal) cell lines, they were exposed to magnetic flux densities of 0.25, 0.5, 1, and 2 millitesla (mT) for 18 h. The cell viability was studied by the 3-(4,5-dimethylthiazol-2-yl)-2,5-diphenyltetrazolium bromide (MTT) assay. The expression levels of hes1 and hsa-circ-0068530 RNAs were studied by the quantitative Real-time-PCR technique.Results:
The inhibition of gastric cancer cell line growth was observed under the influence of electromagnetic fields at different intensities. However, they did not affect the viability of normal cells. A sharp increase in the expression of hes1 and hsa-circ-0068530 genes was observed in normal cells exposed to 2 mT electromagnetic fields.Conclusions:
In general, it can be concluded that the effect of electromagnetic fields on gastric cancer cells depends on their intensity. Magnetic flux densities of 0.25 and 0.5 mT had anti-cancer effects and magnetic flux density of 2 mT showed carcinogenic effects.Keywords
Gastric Cell Line (AGS) Magnetic Field hes1 Expression Hsa-circ-0068530 Expression
1. Background
In recent decades, the use of electricity has expanded significantly and become one of the hallmarks of advanced societies. Therefore, researchers have focused on the biological effects of electromagnetic fields. Several studies have shown a relationship between electromagnetic fields and multiple types of cancer (1, 2). Low-frequency electromagnetic fields can induce heat in tissues and cells. This increase in temperature can lead to cell death (3). It has also been reported that short-term exposure of pregnant rats to the electromagnetic field causes teratogenicity effects in the developing fetus (4). Some researchers believe that low-frequency electromagnetic radiation acts like ionizing waves and causes DNA damage by inducing mutations (5). Increasing the concentration of free radicals and changes in cell behavior following electromagnetic fields irradiation can lead to DNA damage (6). Low-frequency electromagnetic fields can affect cell growth (7), morphology and cell shape (8), carcinogenicity (9), cell differentiation (10), and programmed cell death (11). Exposure to low-frequency electromagnetic fields could increase oxidative stress in chick embryos (12), cultured mammalian cells (13), and human erythrocytes (14). Gastric cancer is a multifactorial disease in which bacterial contamination, environmental factors, and host genetic agents play an important role in its progression (15). A review of statistics from the last 30 years shows that the incidence of gastric cancer in Iran is higher than the global average, and despite the decrease in the incidence of gastric cancer in the world, its incidence is increasing in Iran (16). Studies show that the onset and progression of cancer depend on several factors, including genetic background (17).
The hes1 gene is a target gene for the Notch1 signaling pathway and plays an important role in preserving neural stem cells and intestinal precursor cells and determining cell fate and apoptosis (18). The Notch1 receptor is also one of the most frequent receptors in the Notch signaling pathway. The expression of Notch1-3 and hairy enhancer of split 1 (hes1) has been reported in the human gastric mucosa (19). As known, Notch1 controls the number and fate of intestinal stem cells by increasing hes1 (20). Studies have also shown that Notch receptors and their ligands play an important role in some cancers (21). The expression of Notch1 and hes1 is associated with cancer cell proliferation and angiogenesis, as reported that the expression of these genes is very high in cancer stem cells (CSCs) (22, 23).
A group of non-coding RNAs that have a circular structure is called circRNAs that have been identified for their role in regulating gene expression at the transcriptional level and, subsequently their sponge miRNA function (24). In recent years, the association of circRNAs with a variety of cancers has attracted much attention and has been cited as a biomarker for cancer diagnosis (25).
2. Objectives
This research evaluated the effect of extremely low frequency (ELF) magnetic flux densities (MFDs) of 0.25, 0.5, 1, and 2 mT on hes1 and hsa-Circ-0068530 expression levels.
3. Methods
3.1. Cell Culture
Two AGS cell lines and a Hu02 fibroblast cell line were purchased from the National Genetic Re-sources Center of Iran. Ham's F12 medium (Gibco, USA) was used for gastric cancer cell line culture, and Dulbecco's modified Eagle's medium (DMEM; Gibco, USA) was used for culture of normal Hu02 cells. A humidified incubator at 37 ± 2°C with 5% CO2 was used to maintain each cell line (26).
3.2. Exposure System
Figure 1 shows the exposure system that included a solenoid cylinder explained in our previous study (27, 28). The cells were exposed to ELF magnetic flux densities of 0.25, 0.5, 1, and 2 mT for 18 h. The control and exposure cells were incubated in a constant condition of temperature, humidity, and CO2 (26).
Magnetic field exposure setup
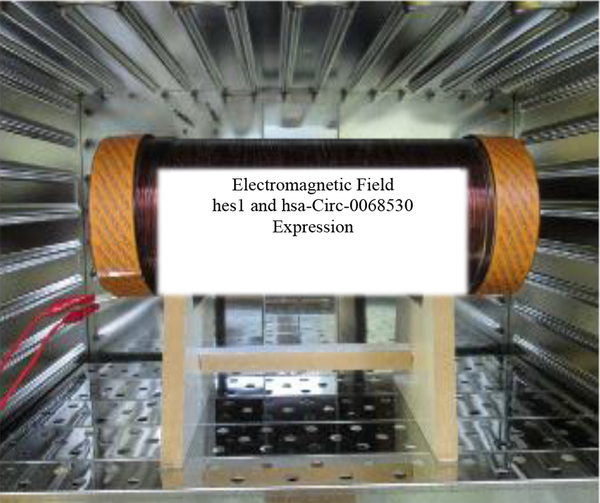
3.3. MTT Assay
The MTT assay was used to evaluate cell viability according to our previous study (26).
3.4. Quantitative Real-Time Polymerase Chain Reaction (qRT-PCR)
The Real-time PCR was used to measure expression changes of hes1 and hsa-Circ-0068530. Total RNA was extracted by using TRIzol reagent (TRI Sigma-Aldrich) according to the manufacturer’s instructions. Total extracted RNA concentration was qualified by measuring the absorbance at 260 nm. Then, cDNA was synthesized from the total extracted RNA using the cDNA Synthesis Kit (Biofact, Korea) with oligo (dT) or random primers. Finally, qRT-PCR was performed by Bioneer ExicyclerTM's 96 Detection System. The primer sequences are listed in Table 1.
Sequences of GAPDH, hes1, and hsa-circ-0068530 Primers
Genes | Sequences |
---|---|
hsa-circ-0068530 | |
Forward | 5'-GGAAATGACAGTGAAGCACCTCC-3' |
Reverse | 5'-GAAGCGGGTCACCTCGTTCATG-3' |
hes1 | |
Forward | 5'-GAGTGCATGAACGAGGTGAC-3' |
Reverse | 5'-GGTCATGGCATTGATCTGGG-3' |
GAPDH | |
Forward | 5'-GCACCGTCAAGGCTGAGAAC-3' |
Reverse | 5'-GGATCTCGCTCCTGGAAGATG-3' |
The 2−ΔΔCT method was applied to determine relative changes in gene expression in samples (26, 29). Each experiment consisted of six separated flasks of cells. After extraction of RNA and synthesis of cDNA, the qRT-PCR test was done in duplicate.
3.5. Statistical Analysis
All statistical analyses were performed with SPSS 25.0 (IBM, SPSS, Chicago, USA). The values are expressed as mean ± standard deviation (SD) from three independent experiments performed in duplicate. Statistical analyses were carried out using a two-independent-sample and Mann-Whitney U test. Bivariate correlations between variables were analyzed by the Spearman test. Differences in values were considered significant if P-value < 0.05.
4. Results
4.1. MTT Assay
The results showed a significant difference in the survival rate of the tumor and normal cells following exposure to different electromagnetic fields (P < 0.05). Electromagnetic fields decreased the survival rate of tumor cells while normal cells continued to multiply and their percentage increased. The inhibitory effect of tumor cell division was observed from an intensity of 0.5 to 2 mT (Figure 2). Thus, electromagnetic fields showed inhibitory effects on cell division in the gastric tumor cell line.
Effects of magnetic flux densities of 0.25 - 2 mT on cell survival of gastric cancer cell and normal cell lines (*** P value < 0.001)
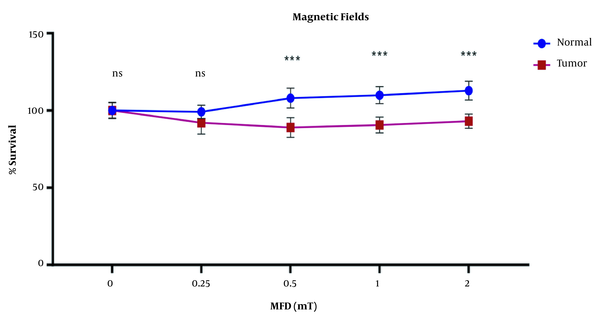
4.2. hes1 Expression
The expression of the hes1 gene in tumor cells was dependent on the intensities of electromagnetic fields, but in normal cells, no change in the expression of this gene was observed with increasing the intensity of the electromagnetic field. The expression of hes1 in gastric cancer cells was downregulated at intensities of 0.25 and 0.5 mT compared to controls, but with an increase in the electromagnetic field to 2 mT, this gene was overexpressed (Figure 3).
Effects of magnetic flux densities of 0.25 - 2 mT on hes1 gene expression in normal and gastric cancer lines
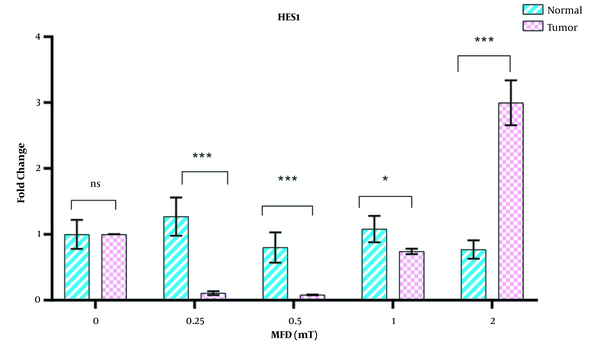
4.3. hsa-circ-0068530
There was a significant difference in the expression of hsa-circ-0068530 between normal and tumor cells exposed to different intensities of the electromagnetic field. The expression of this circRNA in tumor cells initially showed a decreasing trend when exposed to the intensities of 0.25, 0.5, and 1 mT, which overexpressed sharply with increasing the electromagnetic field to 2 mT (2.5 times compared to the control (Figure 4). In normal cells, the expression of hsa-circ-006853 decreased under exposure to electromagnetic fields in comparison with the control group, and the greatest decrease was seen in the magnetic flux density of 2 mT.
Effects of magnetic flux densities of 0.25 - 2 mT on hsa-circ-0068530 expression in normal and gastric cancer lines
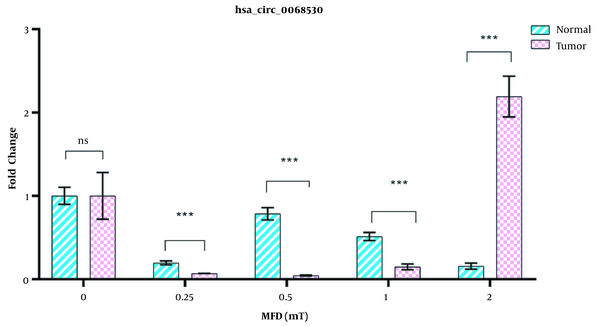
4.4. Correlation Analysis
A positive significant correlation (r = 0.908, 95% P < 0.0001) was observed between the expression levels of hsa-circ-0068530 and hes1 genes in the AGS cell lines exposed to electromagnetic fields (Figure 5A). But, there was no significant correlation between the expression levels of hsa-circ-0068530 and hes1 genes in normal cells exposed to electromagnetic fields (P = 0.540) (Figure 5B).
Correlation analysis between hes1 and hsa-circ-0068530 gene expression in A, gastric tumor cells; and B, normal cell lines exposed to magnetic flux densities of 0.25 - 2 mT
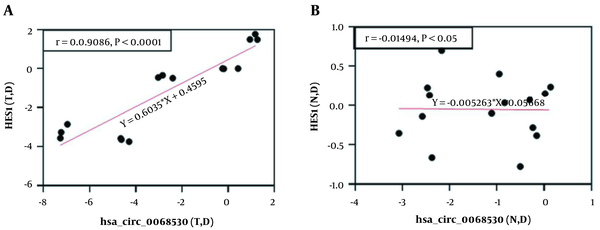
5. Discussion
In this study, AGS gastric cancer cell line and normal fibroblast cell line were exposed to ELF-MFDs of 0.25, 0.5, 1, and 2 mT. The results showed that normal cells continued to proliferate over time and their viability increased. However, the survival rate of tumor cells decreased following exposure to the electromagnetic fields. Therefore, it seems that the magnetic fields can damage gastric tumor cells and prevent them from growing and multiplying. Interaction of ELF-MF with living organisms can induce different biological effects that depend on the type, MFD, frequency, and time of exposure (30, 31).
In recent years, concerns have been raised about the strong electromagnetic fields of household appliances, and more importantly, high-pressure towers and cell phone waves, on human health. It seems that with the increasing intensity of electromagnetic fields, their biological effects on living systems increase (32). The production of liver cysts with fibrotic bands, severe obstructive hepatitis, and edema in chick embryos exposed to 50 Hz electromagnetic field (33), DNA damage due to oxidative stress (34), and slow cell division due to the inhibition of mitotic spindle formation (35) have been reported. In the present study, normal fibroblast cells following exposure to magnetic fields showed no reduction in viability, which is contrary to the findings of the above-mentioned studies. This can be attributed to the different magnetic intensities used in the present study. The inhibitory effect of magnetic fields on the growth and proliferation of gastric cancer cells can be attributed to the DNA fragmentation of cancer cells, inhibition of antioxidant enzymes, and reduced cell tolerance to oxidative stress. These events affect the cell signal transduction pathways and the expression of genes specific for the inflammatory response, cell growth, differentiation, and proliferation, and generally reduce cancer cell growth and inhibit its proliferation (36). Cancer and normal cells showed different cellular behaviors to interact with electromagnetic fields. It has been shown that electromagnetic fields induce an increase in free radicals in the environment. On the other hand, an increase in free radicals leads to oxidative stress, which is one of the causes of cell death (37). In normal cells, several detoxification processes regulated through antioxidant enzymes balance the ROS levels. Therefore, the homeostasis of ROS is well sustained, which can contribute to the maintenance of redox balance in normal cells (38).
In the present study, significant differences in terms of hes1 expression were observed in gastric tumor cells exposed to different electromagnetic field intensities. Expression of this gene decreased at low intensities of electromagnetic field (0.25 and 0.5 mT) in tumor cells but increased sharply at higher intensities (2 mT). It seems that intensities above 2 mT of electromagnetic fields may lead to increased expression of this gene in tumor cells. Research has shown that hes1 is overexpressed in cancer stem cells Therefore using down-regulation of hes1 by targeting therapy can reduce the number of CSCs (23). Hence, hes1 is an oncogene that can lead to the development of gastric cancer (39). A decrease in hes1 expression was observed at low intensities of 0.25 and 0.5 mT of magnetic fields. However, when the intensity of the electromagnetic field increased to 2 mT, a sharp increase in the expression of this gene was observed in gastric cancer cells, which could indicate the carcinogenic effect of electromagnetic fields at high intensities. Therefore, it seems that the electromagnetic field can also exert its anti-proliferative effect through changes in the Notch transduction pathway. However, more studies are needed in this area.
One of the most important molecular elements involved in regulating gene expression is circRNAs, which can originate in either exon or intron regions (40). In recent years, the role of circRNAs in causing cancer has received much attention, which has led to the identification of a large number of circRNAs involved in a variety of cancers (41). As known, CircRNAs have multiple regions for binding to miRNAs and act as miRNA sponges. They play a role in various cancers (42). In the present study, a sharp increase in the expression of hsa-circ-0068530 in a magnetic flux density of 2mT could indicate its carcinogenic role in gastric cancer. In normal cells, no significant difference was observed in the expression hsa-circ-0068530 at different intensities of magnetic fields. In this study, the expression of hsa-circ-0068530 in the cancer cell line was studied for the first time, so its importance must be confirmed in other studies. Correlation analysis was performed to find the relationship between the hes1 gene and its circRNA hsa-circ-0068530, which showed a direct relationship between gene expression and its circRNA in tumor cells in electromagnetic fields. There was no correlation between hes1 and hsa-circ-0068530 gene expression in normal cells. Considering the effect of electromagnetic fields on reducing the expression of this circRNA in normal cells, it can be said that the electromagnetic field is safe for normal cells.
5.1. Conclusion
The inhibition of AGS gastric cancer cell growth under exposure to electromagnetic fields at intensities of 0.25 and 0.5 mT was observed, indicating the cytotoxic effect of these waves on the tumor. At intensities of 1 and 2 mT, the electromagnetic fields showed an increase in the expression of hes1 and hsa-circ-0068530 genes, which could indicate the carcinogenic effects of electromagnetic fields at high intensities.
Acknowledgements
References
-
1.
Blackman CF. Can EMF exposure during development leave an imprint later in life? Electromagn Biol Med. 2006;25(4):217-25. [PubMed ID: 17178582]. https://doi.org/10.1080/15368370601034086.
-
2.
Belpomme D, Irigaray P, Hardell L. Electromagnetic fields as cancer-causing agents. Environ Res. 2008;107(2):289-90. https://doi.org/10.1016/j.envres.2008.01.017.
-
3.
Juutilainen J. Developmental effects of electromagnetic fields. Bioelectromagnetics. 2005;Suppl 7:S107-15. [PubMed ID: 16037961]. https://doi.org/10.1002/bem.20125.
-
4.
Yang MJ, Liu JY, Wang YF, Lang HY, Miao X, Zhang LY, et al. Effects of electromagnetic pulse on polydactyly of mouse fetuses. Theriogenology. 2013;80(1):18-23. [PubMed ID: 23623167]. https://doi.org/10.1016/j.theriogenology.2013.03.004.
-
5.
Reese JA, Jostes RF, Frazier ME. Exposure of mammalian cells to 60-Hz magnetic or electric fields: Analysis for DNA single-strand breaks. Bioelectromagnetics. 1988;9(3):237-47. [PubMed ID: 3178898]. https://doi.org/10.1002/bem.2250090305.
-
6.
Barnes FS. Some engineering models for interactions of electric and magnetic fields with biological systems. Bioelectromagnetics. 1992;Suppl 1:67-85. [PubMed ID: 1285723]. https://doi.org/10.1002/bem.2250130708.
-
7.
Patruno A, Ferrone A, Costantini E, Franceschelli S, Pesce M, Speranza L, et al. Extremely low-frequency electromagnetic fields accelerates wound healing modulating MMP-9 and inflammatory cytokines. Cell Prolif. 2018;51(2). e12432. [PubMed ID: 29357406]. [PubMed Central ID: PMC6528910]. https://doi.org/10.1111/cpr.12432.
-
8.
Koziorowska A, Romerowicz-Misielak M, Solek P, Koziorowski M. Extremely low frequency variable electromagnetic fields affect cancer and noncancerous cells in vitro differently: Preliminary study. Electromagn Biol Med. 2018;37(1):35-42. [PubMed ID: 29513614]. https://doi.org/10.1080/15368378.2017.1408021.
-
9.
Kocaman A, Altun G, Kaplan AA, Deniz OG, Yurt KK, Kaplan S. Genotoxic and carcinogenic effects of non-ionizing electromagnetic fields. Environ Res. 2018;163:71-9. [PubMed ID: 29427953]. https://doi.org/10.1016/j.envres.2018.01.034.
-
10.
Bai W, Li M, Xu W, Zhang M. Comparison of effects of high- and low-frequency electromagnetic fields on proliferation and differentiation of neural stem cells. Neurosci Lett. 2021;741:135463. [PubMed ID: 33129846]. https://doi.org/10.1016/j.neulet.2020.135463.
-
11.
Yadamani S, Neamati A, Homayouni-Tabrizi M, Beyramabadi SA, Yadamani S, Gharib A, et al. Treatment of the breast cancer by using low frequency electromagnetic fields and Mn(II) complex of a Schiff base derived from the pyridoxal. Breast. 2018;41:107-12. [PubMed ID: 30025273]. https://doi.org/10.1016/j.breast.2018.07.001.
-
12.
Siddiqi N, Al Nazwani N. Effects of electromagnetic field on the development of chick embryo: An in vivo study. In: Yeap KH, Hirasawa K, editors. Electromagnetic fields and waves. Norderstedt, Germany: Books on Demand; 2019. https://doi.org/10.5772/intechopen.84704.
-
13.
Kuzniar A, Laffeber C, Eppink B, Bezstarosti K, Dekkers D, Woelders H, et al. Semi-quantitative proteomics of mammalian cells upon short-term exposure to non-ionizing electromagnetic fields. PLoS One. 2017;12(2). e0170762. [PubMed ID: 28234898]. [PubMed Central ID: PMC5325209]. https://doi.org/10.1371/journal.pone.0170762.
-
14.
Hosseinabadi MB, Khanjani N, Samaei SE, Nazarkhani F. Effect of long-term occupational exposure to extremely low-frequency electromagnetic fields on proinflammatory cytokine and hematological parameters. Int J Radiat Biol. 2019;95(11):1573-80. [PubMed ID: 31329007]. https://doi.org/10.1080/09553002.2019.1642542.
-
15.
Mareel M, Leroy A. Clinical, cellular, and molecular aspects of cancer invasion. Physiol Rev. 2003;83(2):337-76. [PubMed ID: 12663862]. https://doi.org/10.1152/physrev.00024.2002.
-
16.
Khalighinejad N, Hariri H, Behnamfar O, Yousefi A, Momeni A. Adenoviral gene therapy in gastric cancer: A review. World J Gastroenterol. 2008;14(2):180-4. [PubMed ID: 18186552]. [PubMed Central ID: PMC2675111]. https://doi.org/10.3748/wjg.14.180.
-
17.
Wang D, Sadee W. Searching for polymorphisms that affect gene expression and mRNA processing: example ABCB1 (MDR1). AAPS J. 2006;8(3):E515-20. [PubMed ID: 17025270]. [PubMed Central ID: PMC2761059]. https://doi.org/10.1208/aapsj080361.
-
18.
Brown DM, Lee HC, Liu S, Quick CM, Fernandes LM, Simmen FA, et al. Notch-1 signaling activation and progesterone receptor expression in ectopic lesions of women with endometriosis. J Endocr Soc. 2018;2(7):765-78. [PubMed ID: 30151432]. [PubMed Central ID: PMC6106104]. https://doi.org/10.1210/js.2018-00007.
-
19.
Katoh M, Katoh M. Notch signaling in gastrointestinal tract (review). Int J Oncol. 2007;30(1):247-51. [PubMed ID: 17143535].
-
20.
Kay SK, Harrington HA, Shepherd S, Brennan K, Dale T, Osborne JM, et al. The role of the Hes1 crosstalk hub in Notch-Wnt interactions of the intestinal crypt. PLoS Comput Biol. 2017;13(2). e1005400. [PubMed ID: 28245235]. [PubMed Central ID: PMC5363986]. https://doi.org/10.1371/journal.pcbi.1005400.
-
21.
Rani A, Greenlaw R, Smith RA, Galustian C. HES1 in immunity and cancer. Cytokine Growth Factor Rev. 2016;30:113-7. [PubMed ID: 27066918]. https://doi.org/10.1016/j.cytogfr.2016.03.010.
-
22.
Liu X, Yun F, Shi L, Li ZH, Luo NR, Jia YF. Roles of signaling pathways in the epithelial-mesenchymal transition in cancer. Asian Pac J Cancer Prev. 2015;16(15):6201-6. [PubMed ID: 26434817]. https://doi.org/10.7314/apjcp.2015.16.15.6201.
-
23.
Yan B, Liu L, Zhao Y, Xiu LJ, Sun DZ, Liu X, et al. Xiaotan Sanjie decoction attenuates tumor angiogenesis by manipulating Notch-1-regulated proliferation of gastric cancer stem-like cells. World J Gastroenterol. 2014;20(36):13105-18. [PubMed ID: 25278704]. [PubMed Central ID: PMC4177489]. https://doi.org/10.3748/wjg.v20.i36.13105.
-
24.
Li P, Chen H, Chen S, Mo X, Li T, Xiao B, et al. Circular RNA 0000096 affects cell growth and migration in gastric cancer. Br J Cancer. 2017;116(5):626-33. [PubMed ID: 28081541]. [PubMed Central ID: PMC5344286]. https://doi.org/10.1038/bjc.2016.451.
-
25.
Li P, Chen S, Chen H, Mo X, Li T, Shao Y, et al. Using circular RNA as a novel type of biomarker in the screening of gastric cancer. Clin Chim Acta. 2015;444:132-6. [PubMed ID: 25689795]. https://doi.org/10.1016/j.cca.2015.02.018.
-
26.
Mansoury F, Babaei N, Abdi S, Entezari M, Doosti A. Evaluation of the PTEN and circRNA-CDR1as gene expression changes in gastric cancer and normal cell lines following the exposure to weak and moderate 50 hz electromagnetic fields. Int J Cancer Manag. 2021;14(4). e111079. https://doi.org/10.5812/ijcm.111079.
-
27.
Abdi S, Dorranian D, Naderi GA, Razavi AE. Changes in physicochemical charachteristics of human low density lipoprotein nano-particles by electromagnetic field exposure. STUD U BABES-BOL CHE. 2016;61(1):185-97.
-
28.
Abdi S, Dorranian D, Razavi AE, Naderi GA, Boshtam M, Ghorannevis M. Evaluation of the effects of weak and moderate static magnetic fields on the characteristics of human low density lipoprotein in vitro. Bioelectromagnetics. 2013;34(5):397-404. [PubMed ID: 23361580]. https://doi.org/10.1002/bem.21779.
-
29.
Mansoury F, Babaei N, Abdi S, Entezari M, Doosti A. Changes in NOTCH1 gene and its regulatory circRNA, hsa_circ_0005986 expression pattern in human gastric adenocarcinoma and human normal fibroblast cell line following the exposure to extremely low frequency magnetic field. Electromagn Biol Med. 2021;40(3):375-83. [PubMed ID: 33620018]. https://doi.org/10.1080/15368378.2021.1891092.
-
30.
Bahar M, Majd A, Abdi S. Effects of (ELF) extremely low frequency (50 Hz) AC and DC magnetic fields on lentil germination and seedlings growth. Iran Phys J. 2009;3(2):12-6.
-
31.
Aalami Zavareh F, Abdi S, Entezari M. Up-regulation of miR-144 and miR-375 in the human gastric cancer cell line following the exposure to extremely low-frequency electromagnetic fields. Int J Radiat Biol. 2021;97(9):1324-32. [PubMed ID: 34125651]. https://doi.org/10.1080/09553002.2021.1941376.
-
32.
McNamee JP, Bellier PV, McLean JR, Marro L, Gajda GB, Thansandote A. DNA damage and apoptosis in the immature mouse cerebellum after acute exposure to a 1 mT, 60 Hz magnetic field. Mutat Res. 2002;513(1-2):121-33. [PubMed ID: 11719097]. https://doi.org/10.1016/s1383-5718(01)00302-3.
-
33.
Lahijani MS, Tehrani DM, Sabouri E. Histopathological and ultrastructural studies on the effects of electromagnetic fields on the liver of preincubated white Leghorn chicken embryo. Electromagn Biol Med. 2009;28(4):391-413. [PubMed ID: 20017630]. https://doi.org/10.3109/15368370903287689.
-
34.
Calvente I, Fernandez MF, Villalba J, Olea N, Nunez MI. Exposure to electromagnetic fields (non-ionizing radiation) and its relationship with childhood leukemia: A systematic review. Sci Total Environ. 2010;408(16):3062-9. [PubMed ID: 20451240]. https://doi.org/10.1016/j.scitotenv.2010.03.039.
-
35.
Boorman GA, McCormick DL, Findlay JC, Hailey JR, Gauger JR, Johnson TR, et al. Chronic toxicity/oncogenicity evaluation of 60 Hz (power frequency) magnetic fields in F344/N rats. Toxicol Pathol. 1999;27(3):267-78. [PubMed ID: 10356702]. https://doi.org/10.1177/019262339902700301.
-
36.
Simko M. Induction of cell activation processes by low frequency electromagnetic fields. ScientificWorldJournal. 2004;4 Suppl 2:4-22. [PubMed ID: 15517098]. [PubMed Central ID: PMC5956406]. https://doi.org/10.1100/tsw.2004.174.
-
37.
Dai X, Wang L, Deivasigamni A, Looi CY, Karthikeyan C, Trivedi P, et al. A novel benzimidazole derivative, MBIC inhibits tumor growth and promotes apoptosis via activation of ROS-dependent JNK signaling pathway in hepatocellular carcinoma. Oncotarget. 2017;8(8):12831-42. [PubMed ID: 28086233]. [PubMed Central ID: PMC5355059]. https://doi.org/10.18632/oncotarget.14606.
-
38.
Aggarwal V, Tuli HS, Varol A, Thakral F, Yerer MB, Sak K, et al. Role of reactive oxygen species in cancer progression: Molecular mechanisms and recent advancements. Biomolecules. 2019;9(11):735. [PubMed ID: doi:10.3390/biom9110735].
-
39.
Ying M, Wang S, Sang Y, Sun P, Lal B, Goodwin CR, et al. Regulation of glioblastoma stem cells by retinoic acid: Role for Notch pathway inhibition. Oncogene. 2011;30(31):3454-67. [PubMed ID: 21383690]. https://doi.org/10.1038/onc.2011.58.
-
40.
Zhang Y, Zhang XO, Chen T, Xiang JF, Yin QF, Xing YH, et al. Circular intronic long noncoding RNAs. Mol Cell. 2013;51(6):792-806. [PubMed ID: 24035497]. https://doi.org/10.1016/j.molcel.2013.08.017.
-
41.
Kristensen LS, Hansen TB, Veno MT, Kjems J. Circular RNAs in cancer: Opportunities and challenges in the field. Oncogene. 2018;37(5):555-65. [PubMed ID: 28991235]. [PubMed Central ID: PMC5799710]. https://doi.org/10.1038/onc.2017.361.
-
42.
Ren S, Lin P, Wang J, Yu H, Lv T, Sun L, et al. Circular RNAs: Promising molecular biomarkers of human aging-related diseases via functioning as an miRNA sponge. Mol Ther Methods Clin Dev. 2020;18:215-29. [PubMed ID: 32637451]. [PubMed Central ID: PMC7326721]. https://doi.org/10.1016/j.omtm.2020.05.027.