Abstract
Keywords
Extracellular Vesicles Exosomes Micro/nanovesicles Menstrual Blood Stem Cells Regenerative Medicine
1. Context
Over the years, cell-based therapies have gained great attention in tissue repair and regeneration (1). Stem cells derived from several sources have been widely studied in regenerative medicine because of their protective role in tissue repair and modulation of the immune system (2). Besides extensive experimental evidence, several clinical trials have shown promising results for stem cell therapy in tissue healing (3). However, previous research shows that, for example, embryonic stem cells and induced pluripotent stem cells (iPSCs) could be associated with teratoma formation (4). Hence, such concerns should be tackled before the large-scale use of stem cells in therapeutic settings (5, 6).
It has been shown that stem cells derived from bone marrow or umbilical cord blood (UCB) can provide much more safety and be used for patient treatment with strong confidence (7, 8). Also, numerous studies are undergoing to evaluate their long-term safety in different contexts. Researchers have also attempted to isolate stem cells from amniotic fluid, placental tissues, dental pulp, and menstrual blood (9, 10). It has been evidenced that Mesenchymal Stem Cells (MSCs) separated from these tissues represent low levels of major histocompatibility molecules, giving them the ability to evade immune destruction and the potential for treating autoimmune diseases and regenerative purposes (11, 12). A body of evidence has reported mesenchymal stem cell changes from various sources for treating specific defects. In this regard, research has focused on finding new sources with higher potential.
Previous studies have shown that menstrual blood-derived stem cells can be used therapeutically (13). Ease of harvest, high immune system, and proliferation activity are possible therapeutic bases for their application (14, 15). However, as mentioned earlier, because of concerns about their safety, stem cell-based therapies are moving towards the use of non-cellular approaches. The secretion of lungs and other vital organs and the long-term survival of administered cells are other risks associated with conventional cell therapy methods (16). At the same time, stem cells have been shown to perform their therapeutic activities largely through paracrine secretion (17).
Stem cell secretion profile analysis highlighted extracellular vesicles as the main secretory material (18). These vesicles can be separated from the culture medium of cultured cells without losing their therapeutic potential, and they can be cryopreserved for an extended period of time (19). Therefore, it seems that extracellular vesicles may play a decisive role in the future of regenerative treatment strategies. The main purpose of the current study is to review the therapeutic use of extracellular vesicles isolated from menstrual blood-derived stem cells in regenerative medicine. We also attempt to provide the mechanistic insights underlying the therapeutic potential of these EVs
2. Endometrial Cell Profile and Regenerative Medicine Applications
Prominent regenerative activity in the endometrium has sparked interest in resident stem cells. The monthly menstrual cycle is associated with cell proliferation and angiogenesis to create the conditions for receiving a fertilized egg. Only 5 - 7 mm of the endometrium thickness is involved in the menstrual cycle. Due to this rapid angiogenesis, various cell types, growth factors, and tissue regeneration processes must work in an orchestrated manner to successfully regenerate the fallen endometrium (20). The upper layer of the endometrium is called the functional layer, which is consisted of glands and loose connective tissue. Below this layer is the basalis layer, which contains vasculature and stem cells (21). During menstruation, the functional layer is destroyed; then, the productive cells are transferred from the basal chamber, which will finally regenerate the eliminated parts (22). In addition to mesenchymal stem cells in the endometrium, epithelial and endothelial progenitor cells have been harvested from the endometrial biopsies (23).
There is a population of epithelial progenitor cells in the glands of the basal layer. The cumulative body supports the presence of epithelial colony-forming units of endometrial biopsy, which can form gland-like structures at appropriate signs (24). Stage specific embryonic antigen-1 (SSEA-1) is expressed in epithelial progenitor cells and reflects their high telomerase activity and proliferation rate. It is worth noting that epithelial progenitor cells cannot be removed from menstrual blood for the following reasons (20, 25). First, they may not be shed in the menstrual blood. Second, they may be secured by many fibroblasts in situ. In fact, these cells are also separated from the endometrium of postmenopausal women, which means that epithelial progenitor cells can be the origin of endometrial stem cells after menopause (26, 27).
Menstrual stem cells (MenSCs) have gained therapeutic appeal over the past decades due to the non-invasive nature of their harvest. They have absolute strength markers with embryonic stem cells such as Oct-4, SSEA-4, and c-kit (28-30). Matrix metalloproteinase activity is significantly higher in stem cells separated from menstrual blood than in other mesenchymal stem cells, indicating their high regenerative potential (31, 32). It has also been shown that these cells can differentiate into various cell types such as heart, cartilage, liver cell-like, and fat cells (33). (Suggested: This renewal capacity is an advantage in tissue engineering and regenerative medicine.) This fact is positive for tissue engineering and regenerative medicine. Our previous studies indicated that MenSCs implanted in cellulose amniotic membranes could enhance wound repair. The higher regenerative potential of MenSCs-seeded membrane was attributed to extracellular matrix protein production and secretion activities (34). We also used a polycaprolactone/gelatin nanofibers neural conduction channel to implant MenSCs in a rat sciatic nerve defect model. Functional analysis studies have shown that MenSCs can significantly increase peripheral nerve repair (33).
3. General Characteristics of Extracellular Vesicles
Intercellular communication is one of the most important mechanisms of body homeostasis (16). Over the past decades, a new cell-to-cell contact method has been discovered. The cells produce large amounts of extracellular vesicles (EVs) and secrete them into the body fluid. This mechanism appears to be highly preserved during evolution (35). Also, EVs can alter the behavior of receptor cells in a variety of ways, including the stimulation by the production of ligands and receptors on their surface, the transfer of genetic molecules such as messenger RNA, DNA, and micro-RNA, and the transfer of lipid and protein (36, 37). Therefore, different roles can be assumed for EVs. Previous studies have shown that EVs have a role in immune monitoring, stem cell maintenance, and cancer cell metastasis. On the other hand, EVs have emerged as a new generation of therapeutics since their secreted EVs can recapitulate most effects of stem cells (38, 39).
4. Extracellular Vesicle Biogenesis
All cell types seem to produce and secrete EVs in a controlled manner (40). All EVs separated from stem and cancer cells have found therapeutic and diagnostic appeal (41). Also, EVs are taken from various body fluids. Although EVs are produced through several pathways, they fall into three broad categories: Exosomes, micro-vesicles, and apoptotic bodies (42, 43). Exosomes are heterogeneous vesicles 30 - 120 nanometers in size that are taken from the endosome and released by multivesicular bodies' attachment to the plasma membrane (2, 44). Although the exact mechanism of exosome production is unclear, several possible pathways have been hypothesized. This system involves the cooperation of the endosomal sorting complex required for transport and its accompanying proteins for sorting and enriching the protein and RNA load in the exosomes, hence determining the exact content of the produced vesicles (45, 46). The micro-vesicles are released through the germination of cytoplasmic protrusions and range in size from 200 to 1000 nanometers. Micro-vesicle secretion involves the rearrangement of cytoskeletal proteins in the influx of calcium ions (47). Once exosomes and micro-vesicles are released, they are not recognizable, so the exact naming of these vesicles is clear. The therapeutic performance of stem cell-derived exosomes and micro-vesicles have been reported in various studies. However, no studies have yet compared their therapeutic activities (18, 48, 49). The third type of extracellular vesicle is apoptotic bodies, which are larger vesicles of 1000 to 5000 nanometers released when the plasma membrane ruptures during apoptosis. However, because of their specific cargo, exosomes, and micro-vesicles are the main fractions of extracellular vesicles that have largely been considered in regenerative medicine (50, 51). Figure 1 shows the schematic of the EV biogenesis counts from menstrual blood.
Schematic diagram of menstrual-blood stem cells from EV biogenesis
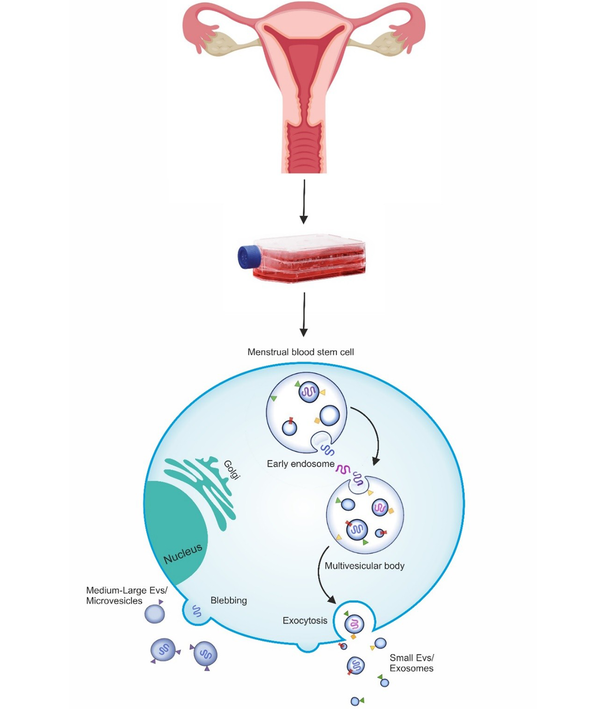
5. Separation and Characterization of Micro/Nano Vesicles
Currently, nanotechnology is becoming a major topic in translation medicine. We can use micro/nanotechnology to separate and detect exosomes and microvesicles. The appropriate methods used to obtain information from inaccessible organism locations are particularly important and characterize a clinical biomarker for providing information on the complexity of several diseases. Cell secretory vesicles contain valuable molecular information from the parental cell, indicating the essential role of intercellular communication. The proper separation method depends on the physicochemical properties, including sample type, size, density, surface charge, light interaction, and available equipment and resources. As known, EVs are complex and heterogeneous because they are secreted from culture media that have their unique compositions. Developing multiple methods for separating EVs with greater specificity and sensitivity is critical. The current methods for separating and enriching EVs and concentrating them include differential ultracentrifugation (52-54), density gradient ultracentrifugation (55, 56), polymer-based precipitation (53, 57), immunoaffinity-based separation (55, 58-61), size-exclusion chromatography (SEC) (62, 63), nanowire-on-micropillar separation (64), and acoustic sorting (65).
6. Physical and Molecular Characterization of Micro/Nano Vesicles
Characterization of samples, such as heterogeneity in size, concentration, or molecular properties, can be done through conventional nanoparticle characterization methods or micro/nanotechnology to separate and detect exosomes and microvesicles. Physical characterization commonly involves transmission electron microscopy (TEM) (66-70), atomic force microscopy (AFM) (71), dynamic light scattering (DLS) (72, 73), nanoparticle tracking analysis (NTA) (74-76), tunable resistive pulse sensing (TRPS) (77, 78), nano-on-chip holographic imaging (79), diagnostic magnetic resonance for exosome detection (80), plasmonic exosome detection (81), flow cytometry (73), Enzyme-linked immune-sorbent assays (ELISA), and Western blot (WB) (82). Molecular characterization is usually done using nucleic acid and protein analysis methods. For example, the best preferable method for RNA is reverse transcription PCR (RT-PCR). Through this method, RNA transcript or miRNA sequencing can be identified to determine transcription (83, 84).
7. Extracellular Vesicles Mechanisms of Action
As known, EVs contain a variety of biologically active agents such as lipids, proteins, and nucleic acids, so it is a treatment package that can also be used for diagnosis (85, 86). The condition of the producing cell directly affects the EV content. For example, cells incubated in hypoxia have been shown to enrich angiogenesis-related microRNAs strongly (87). It is hoped that the exosomes of metastatic cancer cells can be removed before metastasis occurs; therefore, immediate surgery or chemotherapy may be needed to save patients' lives (88). Previous studies have proved the specific interactions between EVs and their receptor cells. Mallegol et al. demonstrated that EVs produced by intestinal epithelial cells can bind exclusively to dendritic cells. Indeed, recent evidence showed that several adhesion molecules expressed on the EVs are responsible for their interactions with different cellular hosts (89).
By turning off antigen information, exosomes can play a role in the functioning of the immune system. For example, Buschow et al. reported that dendritic cell-derived exosomes contain the main set of histocompatibility complex 2 and can elicit a T lymphocyte response (90). In addition, dendritic cell-derived exosomes have been shown to increase the immune response in B lymphocytes (91). Therefore, the stimulatory and inhibitory effects of exosomes have been documented. However, the exact action mechanism of these effects remains to be determined. This potential of exosomes can be used in cancer therapy strategies. Since cancer immunotherapy aims to find an effective and safe way to expose immune cells to cancer antigens, the antigens presented in exosomes can be used effectively for this purpose (92). Clinical trials have been conducted on exosome-based vaccines for cancer therapy, and the preliminary results have shown high immunity to the vaccines produced (93, 94). It has also been shown that the placenta can induce maternal-fetal tolerance through exosomes (95). In addition to these functions in the immune system, the anti-apoptotic, proangiogenic, regenerative, and protective effects of exosomes and micro-vesicles separated from mesenchymal stem cells have been well-established in previous studies (96).
8. Protective Effects of Menstrual Blood Stem Cells Separated EVs on Different Tissues
Fulminant hepatic failure is a severe medical condition in which the liver fails to function properly. Problems associated with it, such as coagulopathy, jaundice, and systemic edema, can lead to significant mortality in clinical settings. The gold standard of treatment is liver transplantation, although it is associated with organ shortage, risk of disease transmission, and immune rejection (97). Stem cell-based therapy has opened up new avenues for regenerative medicine to regenerate the liver. In this regard, stem cells and their EVs have been potentially tested for treating liver defects (98).
Chen et al. used the exosomes from human menstrual blood to treat fulminant hepatic failure. They separated the exosomes from cultured MENCs, characterized them by transmission electron microscopy and Western blot analysis, and analyzed their cytokine content by antibody assay. In vitro studies were conducted on D-GalN/LPS treated with AML12 cells, and in vivo studies were performed on a mice model of fulminant hepatic failure. The exosomes were administered 24 hours before the induction of hepatic failure. The presence of mononuclear cells and the level of apoptotic-activated protein caspase-3 were measured in the treated mice. The cytokine assay showed that the separated vesicles expressed various proteins such as angiopoietin-2, Axl, angiogenin, IGFBP-6, osteoprotegerin, IL-6, and IL-8. Exosomes derived from MenSCs could significantly increase liver function, improve survival rate, and reduce hepatocytes apoptosis. In addition, MenSCs-derived exosomes could reduce the number of mononuclear cells and the level of the apoptotic active protein caspase-3 in the damaged liver. They concluded that EVs separated from MenSCs could be used to treat fulminant hepatic failure in clinics (99).
Wound healing is a complex process that involves several interconnected phases. Disruption of any of these phases can lead to chronic non-healing wounds (100). Several stem cells have been used on skin wounds (101). Recently, some research has shed light on how stem cells are used in wound healing. Also, EVs have been shown to have the main role in the protective effects of mesenchymal stem cells in wound healing (102). Dalirfardouei et al. reported the healing effects of MenSCs-derived exosomes on diabetic wounds. The exosomes were separated from the MenSCs conditioned medium and characterized by scanning electron microscopy and western blot using ultra-centrifugation. A full-thickness excisional wound was made in the back of diabetic mice. Animals were divided into three groups: Mice treated with exosome, mice treated with MenSCs, and wounds treated with phosphate-buffered saline. They showed that exosomes induced M1-M2 macrophage polarization and increased vascularization, evident by the rearrangement of vascular endothelial growth factor A. They also found that re-epithelialization was probably higher in exosome-treated wounds than in other groups through the NF-κB signaling pathway. They also reported less scarring formation in the exosome group. Their results proved using exosomes separated from MenSCs in regenerative and aesthetic medicine (103).
Parkinson's disease, characterized by the death of brain cells, constitutes many neurodegenerative diseases (104). Studies over the past decades have shown that mesenchymal stem cell administration can reduce the symptoms of Parkinson's disease in animal models (105). Li et al. investigated the protective effects of human MenSCs conditioned medium on 1-methyl-4-phenylpyridinium-induced cytotoxicity in neuroblastoma SH-SY5Y cells, an in vitro model of Parkinson's disease. After 24 and 48 hours of incubation with MenSCs, conditioned cell viability, apoptosis, cell proliferation, and mitochondrial membrane potential were investigated. The protein content of the conditioned medium was also analyzed. The results indicated that 1-methyl-4-phenylpyridinium treatment caused inflammatory response, mitochondrial damage, elevated levels of reactive oxygen species, and apoptosis in the SH-SY5Y cell line. Also, the conditioned medium could reduce apoptosis, increase cell viability, and decrease the production of reactive oxygen species. Due to the neuroprotective effects, various neurotrophic factors were observed in the conditioned medium of MenSCs (106).
Old age reduces the chance of in vitro fertilization success by increasing the production of reactive oxygen species that damage the residing follicles (107). However, EVs separated from mesenchymal stem cells have been shown to exhibit antioxidant activity. Therefore, they can increase in vitro fertilization yield at older ages. Marinaro et al. studied the effects of MenSCs-derived EVs on in vitro fertilization models. Stem cells were harvested from menstrual blood, and their surface markers and potential for multiracial differentiation were investigated. Oocytes were separated from 21 B6D2 mice and exposed to sperm from young men aged 8-12 weeks old. The formed zygotes were incubated with different concentrations of EVs, including 0, 10, 20, 40, or 80 µg/mL in KSOM medium. The number of blastocysts was counted, and 25 blastocysts per group were exploited for qPCR. Blastocyst yield was highest in the 20 µg/mL group. This value was significantly higher for all treated groups than for the control group.
The zygotes incubated with 20 - 80 µg/mL of EVs reduced glutathione peroxidase expression, and treatments of 10 - 40 µg/mL decreased superoxide dismutase expression compared to control. However, Bax mRNA expression remained unchanged. They concluded that the radical scavenging activity of EVs from MenSCs could be a potential way of fertilization in the older population (108). Figure 2 shows a schematic diagram of the applications of EVs caused by menstrual blood stem cells in treating different diseases.
Schematic diagram of the applications of EVs caused by menstrual blood stem cells in treating different diseases
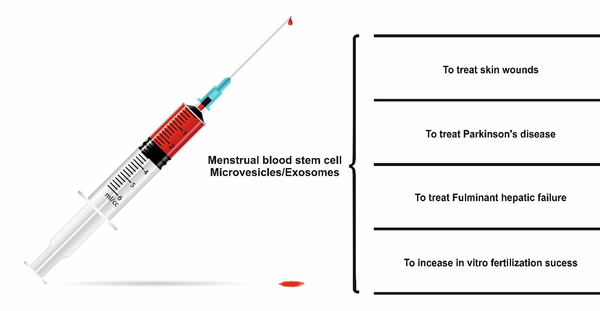
9. Conclusions
With advances in nanotechnology, micro/nanovesicles derived from different cellular sources will be added to therapeutic regimens. On the other hand, with a deep understanding of the composition and biology of the extracellular vesicles in normal physiology and the pathobiology of different diseases, we can devise novel treatment modalities based on these nanoparticles. This paper highlights the promising potential of EVs separated from menstrual blood in regenerative medicine. Also, it summarizes the established measures needed to study these EVs. In conclusion, there is still much work to be done for the large-scale use of these MenSCs-EVs. However, it is obvious that using EVs derived from stem cell sources, potentially those derived from MenSCs, will play a decisive role in the future of regenerative medicine.
References
-
1.
Farzamfar S, Nazeri N, Salehi M, Valizadeh A, Marashi SM, Savari Kouzehkonan G, et al. Will Nanotechnology Bring New Hope for Stem Cell Therapy? Cells Tissues Organs. 2018;206(4-5):229-41. [PubMed ID: 31288229]. https://doi.org/10.1159/000500517.
-
2.
Keshtkar S, Azarpira N, Ghahremani MH. Mesenchymal stem cell-derived extracellular vesicles: novel frontiers in regenerative medicine. Stem Cell Res Ther. 2018;9(1):63. [PubMed ID: 29523213]. [PubMed Central ID: PMC5845209]. https://doi.org/10.1186/s13287-018-0791-7.
-
3.
Galipeau J, Sensebe L. Mesenchymal Stromal Cells: Clinical Challenges and Therapeutic Opportunities. Cell Stem Cell. 2018;22(6):824-33. [PubMed ID: 29859173]. [PubMed Central ID: PMC6434696]. https://doi.org/10.1016/j.stem.2018.05.004.
-
4.
Volarevic V, Markovic BS, Gazdic M, Volarevic A, Jovicic N, Arsenijevic N, et al. Ethical and Safety Issues of Stem Cell-Based Therapy. Int J Med Sci. 2018;15(1):36-45. [PubMed ID: 29333086]. [PubMed Central ID: PMC5765738]. https://doi.org/10.7150/ijms.21666.
-
5.
da Cruz L, Fynes K, Georgiadis O, Kerby J, Luo YH, Ahmado A, et al. Phase 1 clinical study of an embryonic stem cell-derived retinal pigment epithelium patch in age-related macular degeneration. Nat Biotechnol. 2018;36(4):328-37. [PubMed ID: 29553577]. https://doi.org/10.1038/nbt.4114.
-
6.
Oguro H. Generation of Hematopoietic Stem and Progenitor Cells from Human Pluripotent Stem Cells. Methods Mol Biol. 2019;2048:245-57. [PubMed ID: 31396942]. https://doi.org/10.1007/978-1-4939-9728-2_19.
-
7.
Kern S, Eichler H, Stoeve J, Kluter H, Bieback K. Comparative analysis of mesenchymal stem cells from bone marrow, umbilical cord blood, or adipose tissue. Stem Cells. 2006;24(5):1294-301. [PubMed ID: 16410387]. https://doi.org/10.1634/stemcells.2005-0342.
-
8.
Azzopardi JI, Blundell R. Review: Umbilical Cord Stem Cells. Stem Cell Discovery. 2018;8(1):1-11. https://doi.org/10.4236/scd.2018.81001.
-
9.
Peak TC, Praharaj PP, Panigrahi GK, Doyle M, Su Y, Schlaepfer IR, et al. Exosomes secreted by placental stem cells selectively inhibit growth of aggressive prostate cancer cells. Biochem Biophys Res Commun. 2018;499(4):1004-10. [PubMed ID: 29627574]. [PubMed Central ID: PMC5929996]. https://doi.org/10.1016/j.bbrc.2018.04.038.
-
10.
Uzieliene I, Urbonaite G, Tachtamisevaite Z, Mobasheri A, Bernotiene E. The Potential of Menstrual Blood-Derived Mesenchymal Stem Cells for Cartilage Repair and Regeneration: Novel Aspects. Stem Cells Int. 2018;2018:5748126. [PubMed ID: 30627174]. [PubMed Central ID: PMC6304826]. https://doi.org/10.1155/2018/5748126.
-
11.
Patel AN, Silva F. Menstrual blood stromal cells: the potential for regenerative medicine. Regen Med. 2008;3(4):443-4. [PubMed ID: 18588464]. https://doi.org/10.2217/17460751.3.4.443.
-
12.
Verdi J, Tan A, Shoae-Hassani A, Seifalian AM. Endometrial stem cells in regenerative medicine. J Biol Eng. 2014;8:20. [PubMed ID: 25097665]. [PubMed Central ID: PMC4121626]. https://doi.org/10.1186/1754-1611-8-20.
-
13.
Xu Y, Naijun L. Endometrial regenerative cells and endometrial cancer stem cells: new insights may provide novel therapeutic targets. Int J Clin Exp Med. 2019;12(8):9607-15.
-
14.
Rahimi M, Mohseni-Kouchesfehani H, Zarnani AH, Mobini S, Nikoo S, Kazemnejad S. Evaluation of menstrual blood stem cells seeded in biocompatible Bombyx mori silk fibroin scaffold for cardiac tissue engineering. J Biomater Appl. 2014;29(2):199-208. [PubMed ID: 24445773]. https://doi.org/10.1177/0885328213519835.
-
15.
Meng X, Ichim TE, Zhong J, Rogers A, Yin Z, Jackson J, et al. Endometrial regenerative cells: a novel stem cell population. J Transl Med. 2007;5:57. [PubMed ID: 18005405]. [PubMed Central ID: PMC2212625]. https://doi.org/10.1186/1479-5876-5-57.
-
16.
Farzamfar S, Hasanpour A, Nazeri N, Razavi H, Salehi M, Shafei S, et al. Extracellular micro/nanovesicles rescue kidney from ischemia-reperfusion injury. J Cell Physiol. 2019;234(8):12290-300. [PubMed ID: 30609022]. https://doi.org/10.1002/jcp.27998.
-
17.
Tapparo M, Bruno S, Collino F, Togliatto G, Deregibus MC, Provero P, et al. Renal Regenerative Potential of Extracellular Vesicles Derived from miRNA-Engineered Mesenchymal Stromal Cells. Int J Mol Sci. 2019;20(10). [PubMed ID: 31091699]. [PubMed Central ID: PMC6567878]. https://doi.org/10.3390/ijms20102381.
-
18.
Stahl PD, Raposo G. Extracellular Vesicles: Exosomes and Microvesicles, Integrators of Homeostasis. Physiology (Bethesda). 2019;34(3):169-77. [PubMed ID: 30968753]. https://doi.org/10.1152/physiol.00045.2018.
-
19.
de Jong OG, Kooijmans SAA, Murphy DE, Jiang L, Evers MJW, Sluijter JPG, et al. Drug Delivery with Extracellular Vesicles: From Imagination to Innovation. Acc Chem Res. 2019;52(7):1761-70. [PubMed ID: 31181910]. [PubMed Central ID: PMC6639984]. https://doi.org/10.1021/acs.accounts.9b00109.
-
20.
Gargett CE, Nguyen HP, Ye L. Endometrial regeneration and endometrial stem/progenitor cells. Rev Endocr Metab Disord. 2012;13(4):235-51. [PubMed ID: 22847235]. https://doi.org/10.1007/s11154-012-9221-9.
-
21.
Cousins FL, O DF, Gargett CE. Endometrial stem/progenitor cells and their role in the pathogenesis of endometriosis. Best Pract Res Clin Obstet Gynaecol. 2018;50:27-38. [PubMed ID: 29503126]. https://doi.org/10.1016/j.bpobgyn.2018.01.011.
-
22.
Chan RWS, Cao M, Ng EHY, Yeung WSB. Microenvironmental regulation of human endometrial mesenchymal stem-like cells. The 35th Annual Meeting of European Society of Human Reproduction and Embryology (ESHRE). Oxford University Press; 2019.
-
23.
Gargett CE, Chan RW, Schwab KE. Endometrial stem cells. Curr Opin Obstet Gynecol. 2007;19(4):377-83. [PubMed ID: 17625422]. https://doi.org/10.1097/GCO.0b013e328235a5c6.
-
24.
Gargett CE, Schwab KE, Zillwood RM, Nguyen HP, Wu D. Isolation and culture of epithelial progenitors and mesenchymal stem cells from human endometrium. Biol Reprod. 2009;80(6):1136-45. [PubMed ID: 19228591]. [PubMed Central ID: PMC2849811]. https://doi.org/10.1095/biolreprod.108.075226.
-
25.
Gargett CE, Ye L. Endometrial reconstruction from stem cells. Fertil Steril. 2012;98(1):11-20. [PubMed ID: 22657248]. https://doi.org/10.1016/j.fertnstert.2012.05.004.
-
26.
Gargett CE, Schwab KE, Brosens JJ, Puttemans P, Benagiano G, Brosens I. Potential role of endometrial stem/progenitor cells in the pathogenesis of early-onset endometriosis. Mol Hum Reprod. 2014;20(7):591-8. [PubMed ID: 24674992]. https://doi.org/10.1093/molehr/gau025.
-
27.
Gargett CE, Schwab KE, Deane JA. Endometrial stem/progenitor cells: the first 10 years. Hum Reprod Update. 2016;22(2):137-63. [PubMed ID: 26552890]. [PubMed Central ID: PMC4755439]. https://doi.org/10.1093/humupd/dmv051.
-
28.
Hapangama DK, Drury J, Da Silva L, Al-Lamee H, Earp A, Valentijn AJ, et al. Abnormally located SSEA1+/SOX9+ endometrial epithelial cells with a basalis-like phenotype in the eutopic functionalis layer may play a role in the pathogenesis of endometriosis. Hum Reprod. 2019;34(1):56-68. [PubMed ID: 30496412]. [PubMed Central ID: PMC6295963]. https://doi.org/10.1093/humrep/dey336.
-
29.
Lopez-Perez N, Gil-Sanchis C, Ferrero H, Faus A, Diaz A, Pellicer A, et al. Human Endometrial Reconstitution From Somatic Stem Cells: The Importance of Niche-Like Cells. Reprod Sci. 2019;26(1):77-87. [PubMed ID: 29575998]. https://doi.org/10.1177/1933719118766251.
-
30.
Yin M, Zhou HJ, Lin C, Long L, Yang X, Zhang H, et al. CD34(+)KLF4(+) Stromal Stem Cells Contribute to Endometrial Regeneration and Repair. Cell Rep. 2019;27(9):2709-2724 e3. [PubMed ID: 31141693]. [PubMed Central ID: PMC6548470]. https://doi.org/10.1016/j.celrep.2019.04.088.
-
31.
Cuenca J, Le-Gatt A, Castillo V, Belletti J, Diaz M, Kurte GM, et al. The Reparative Abilities of Menstrual Stem Cells Modulate the Wound Matrix Signals and Improve Cutaneous Regeneration. Front Physiol. 2018;9:464. [PubMed ID: 29867527]. [PubMed Central ID: PMC5960687]. https://doi.org/10.3389/fphys.2018.00464.
-
32.
Lin J, Xiang D, Zhang JL, Allickson J, Xiang C. Plasticity of human menstrual blood stem cells derived from the endometrium. J Zhejiang Univ Sci B. 2011;12(5):372-80. [PubMed ID: 21528491]. [PubMed Central ID: PMC3087093]. https://doi.org/10.1631/jzus.B1100015.
-
33.
Farzamfar S, Naseri-Nosar M, Ghanavatinejad A, Vaez A, Zarnani AH, Salehi M. Sciatic nerve regeneration by transplantation of menstrual blood-derived stem cells. Mol Biol Rep. 2017;44(5):407-12. [PubMed ID: 28980161]. https://doi.org/10.1007/s11033-017-4124-1.
-
34.
Farzamfar S, Salehi M, Ehterami A, Naseri-Nosar M, Vaez A, Zarnani AH, et al. Promotion of excisional wound repair by a menstrual blood-derived stem cell-seeded decellularized human amniotic membrane. Biomed Eng Lett. 2018;8(4):393-8. [PubMed ID: 30603224]. [PubMed Central ID: PMC6209087]. https://doi.org/10.1007/s13534-018-0084-1.
-
35.
Raposo G, Stoorvogel W. Extracellular vesicles: exosomes, microvesicles, and friends. J Cell Biol. 2013;200(4):373-83. [PubMed ID: 23420871]. [PubMed Central ID: PMC3575529]. https://doi.org/10.1083/jcb.201211138.
-
36.
E. L. Andaloussi S, Mager I, Breakefield XO, Wood MJ. Extracellular vesicles: biology and emerging therapeutic opportunities. Nat Rev Drug Discov. 2013;12(5):347-57. [PubMed ID: 23584393]. https://doi.org/10.1038/nrd3978.
-
37.
van Niel G, D'Angelo G, Raposo G. Shedding light on the cell biology of extracellular vesicles. Nat Rev Mol Cell Biol. 2018;19(4):213-28. [PubMed ID: 29339798]. https://doi.org/10.1038/nrm.2017.125.
-
38.
Vader P, Mol EA, Pasterkamp G, Schiffelers RM. Extracellular vesicles for drug delivery. Adv Drug Deliv Rev. 2016;106(Pt A):148-56. [PubMed ID: 26928656]. https://doi.org/10.1016/j.addr.2016.02.006.
-
39.
Boulanger CM, Loyer X, Rautou PE, Amabile N. Extracellular vesicles in coronary artery disease. Nat Rev Cardiol. 2017;14(5):259-72. [PubMed ID: 28150804]. https://doi.org/10.1038/nrcardio.2017.7.
-
40.
Marote A, Teixeira FG, Mendes-Pinheiro B, Salgado AJ. MSCs-Derived Exosomes: Cell-Secreted Nanovesicles with Regenerative Potential. Front Pharmacol. 2016;7:231. [PubMed ID: 27536241]. [PubMed Central ID: PMC4971062]. https://doi.org/10.3389/fphar.2016.00231.
-
41.
Tan A, De La Pena H, Seifalian AM. The application of exosomes as a nanoscale cancer vaccine. Int J Nanomedicine. 2010;5:889-900. [PubMed ID: 21116329]. [PubMed Central ID: PMC2990382]. https://doi.org/10.2147/IJN.S13402.
-
42.
Colombo M, Raposo G, Thery C. Biogenesis, secretion, and intercellular interactions of exosomes and other extracellular vesicles. Annu Rev Cell Dev Biol. 2014;30:255-89. [PubMed ID: 25288114]. https://doi.org/10.1146/annurev-cellbio-101512-122326.
-
43.
Tkach M, Thery C. Communication by Extracellular Vesicles: Where We Are and Where We Need to Go. Cell. 2016;164(6):1226-32. [PubMed ID: 26967288]. https://doi.org/10.1016/j.cell.2016.01.043.
-
44.
Zuba-Surma EK, Labedz-Maslowska A, Cheng G, Kmiotek-Wasylewska K, Bobis-Wozowicz S, Sekula M, et al. Biological and regenerative properties of extracellular vesicles from mesenchymal stem cells of various origin in cardiovascular regeneration. J Extracell Vesicles. 2018;7:136-7.
-
45.
Bjorge IM, Kim SY, Mano JF, Kalionis B, Chrzanowski W. Extracellular vesicles, exosomes and shedding vesicles in regenerative medicine - a new paradigm for tissue repair. Biomater Sci. 2017;6(1):60-78. [PubMed ID: 29184934]. https://doi.org/10.1039/c7bm00479f.
-
46.
Zhao T, Sun F, Liu J, Ding T, She J, Mao F, et al. Emerging Role of Mesenchymal Stem Cell-derived Exosomes in Regenerative Medicine. Curr Stem Cell Res Ther. 2019;14(6):482-94. [PubMed ID: 30819086]. https://doi.org/10.2174/1574888X14666190228103230.
-
47.
Jing H, He X, Zheng J. Exosomes and regenerative medicine: state of the art and perspectives. Transl Res. 2018;196:1-16. [PubMed ID: 29432720]. https://doi.org/10.1016/j.trsl.2018.01.005.
-
48.
Busatto S, Pham A, Suh A, Shapiro S, Wolfram J. Organotropic drug delivery: Synthetic nanoparticles and extracellular vesicles. Biomed Microdevices. 2019;21(2):46. [PubMed ID: 30989386]. [PubMed Central ID: PMC6686136]. https://doi.org/10.1007/s10544-019-0396-7.
-
49.
Taheri B, Soleimani M, Fekri Aval S, Esmaeili E, Bazi Z, Zarghami N. Induced pluripotent stem cell-derived extracellular vesicles: A novel approach for cell-free regenerative medicine. J Cell Physiol. 2019;234(6):8455-64. [PubMed ID: 30478831]. https://doi.org/10.1002/jcp.27775.
-
50.
Brock CK, Wallin ST, Ruiz OE, Samms KM, Mandal A, Sumner EA, et al. Stem cell proliferation is induced by apoptotic bodies from dying cells during epithelial tissue maintenance. Nat Commun. 2019;10(1):1044. [PubMed ID: 30837472]. [PubMed Central ID: PMC6400930]. https://doi.org/10.1038/s41467-019-09010-6.
-
51.
Yuan FL, Xu RS, Ye JX, Zhao MD, Ren LJ, Li X. Apoptotic bodies from endplate chondrocytes enhance the oxidative stress-induced mineralization by regulating PPi metabolism. J Cell Mol Med. 2019;23(5):3665-75. [PubMed ID: 30892812]. [PubMed Central ID: PMC6484318]. https://doi.org/10.1111/jcmm.14268.
-
52.
Thery C, Amigorena S, Raposo G, Clayton A. Isolation and characterization of exosomes from cell culture supernatants and biological fluids. Curr Protoc Cell Biol. 2006;Chapter 3:Unit 3 22. [PubMed ID: 18228490]. https://doi.org/10.1002/0471143030.cb0322s30.
-
53.
Alvarez ML, Khosroheidari M, Kanchi Ravi R, DiStefano JK. Comparison of protein, microRNA, and mRNA yields using different methods of urinary exosome isolation for the discovery of kidney disease biomarkers. Kidney Int. 2012;82(9):1024-32. [PubMed ID: 22785172]. https://doi.org/10.1038/ki.2012.256.
-
54.
Linares R, Tan S, Gounou C, Arraud N, Brisson AR. High-speed centrifugation induces aggregation of extracellular vesicles. J Extracell Vesicles. 2015;4:29509. [PubMed ID: 26700615]. [PubMed Central ID: PMC4689953]. https://doi.org/10.3402/jev.v4.29509.
-
55.
Tauro BJ, Greening DW, Mathias RA, Ji H, Mathivanan S, Scott AM, et al. Comparison of ultracentrifugation, density gradient separation, and immunoaffinity capture methods for isolating human colon cancer cell line LIM1863-derived exosomes. Methods. 2012;56(2):293-304. [PubMed ID: 22285593]. https://doi.org/10.1016/j.ymeth.2012.01.002.
-
56.
Zhang Z, Wang C, Li T, Liu Z, Li L. Comparison of ultracentrifugation and density gradient separation methods for isolating Tca8113 human tongue cancer cell line-derived exosomes. Oncol Lett. 2014;8(4):1701-6. [PubMed ID: 25202395]. [PubMed Central ID: PMC4156197]. https://doi.org/10.3892/ol.2014.2373.
-
57.
Zeringer E, Barta T, Li M, Vlassov AV. Strategies for isolation of exosomes. Cold Spring Harb Protoc. 2015;2015(4):319-23. [PubMed ID: 25834266]. https://doi.org/10.1101/pdb.top074476.
-
58.
Bobrie A, Colombo M, Krumeich S, Raposo G, Thery C. Diverse subpopulations of vesicles secreted by different intracellular mechanisms are present in exosome preparations obtained by differential ultracentrifugation. J Extracell Vesicles. 2012;1. [PubMed ID: 24009879]. [PubMed Central ID: PMC3760636]. https://doi.org/10.3402/jev.v1i0.18397.
-
59.
Mathivanan S, Lim JW, Tauro BJ, Ji H, Moritz RL, Simpson RJ. Proteomics analysis of A33 immunoaffinity-purified exosomes released from the human colon tumor cell line LIM1215 reveals a tissue-specific protein signature. Mol Cell Proteomics. 2010;9(2):197-208. [PubMed ID: 19837982]. [PubMed Central ID: PMC2830834]. https://doi.org/10.1074/mcp.M900152-MCP200.
-
60.
Vaidyanathan R, Naghibosadat M, Rauf S, Korbie D, Carrascosa LG, Shiddiky MJ, et al. Detecting exosomes specifically: a multiplexed device based on alternating current electrohydrodynamic induced nanoshearing. Anal Chem. 2014;86(22):11125-32. [PubMed ID: 25324037]. https://doi.org/10.1021/ac502082b.
-
61.
Taylor DD, Gercel-Taylor C. MicroRNA signatures of tumor-derived exosomes as diagnostic biomarkers of ovarian cancer. Gynecol Oncol. 2008;110(1):13-21. [PubMed ID: 18589210]. https://doi.org/10.1016/j.ygyno.2008.04.033.
-
62.
Lobb RJ, Becker M, Wen SW, Wong CS, Wiegmans AP, Leimgruber A, et al. Optimized exosome isolation protocol for cell culture supernatant and human plasma. J Extracell Vesicles. 2015;4:27031. [PubMed ID: 26194179]. [PubMed Central ID: PMC4507751]. https://doi.org/10.3402/jev.v4.27031.
-
63.
Szatanek R, Baran J, Siedlar M, Baj-Krzyworzeka M. Isolation of extracellular vesicles: Determining the correct approach (Review). Int J Mol Med. 2015;36(1):11-7. [PubMed ID: 25902369]. [PubMed Central ID: PMC4494580]. https://doi.org/10.3892/ijmm.2015.2194.
-
64.
Wang Z, Wu HJ, Fine D, Schmulen J, Hu Y, Godin B, et al. Ciliated micropillars for the microfluidic-based isolation of nanoscale lipid vesicles. Lab Chip. 2013;13(15):2879-82. [PubMed ID: 23743667]. [PubMed Central ID: PMC3740541]. https://doi.org/10.1039/c3lc41343h.
-
65.
Lee K, Shao H, Weissleder R, Lee H. Acoustic purification of extracellular microvesicles. ACS Nano. 2015;9(3):2321-7. [PubMed ID: 25672598]. [PubMed Central ID: PMC4373978]. https://doi.org/10.1021/nn506538f.
-
66.
van der Pol E, Hoekstra AG, Sturk A, Otto C, van Leeuwen TG, Nieuwland R. Optical and non-optical methods for detection and characterization of microparticles and exosomes. J Thromb Haemost. 2010;8(12):2596-607. [PubMed ID: 20880256]. https://doi.org/10.1111/j.1538-7836.2010.04074.x.
-
67.
van der Pol E, van Gemert MJ, Sturk A, Nieuwland R, van Leeuwen TG. Single vs. swarm detection of microparticles and exosomes by flow cytometry. J Thromb Haemost. 2012;10(5):919-30. [PubMed ID: 22394434]. https://doi.org/10.1111/j.1538-7836.2012.04683.x.
-
68.
van der Pol E, Coumans FA, Grootemaat AE, Gardiner C, Sargent IL, Harrison P, et al. Particle size distribution of exosomes and microvesicles determined by transmission electron microscopy, flow cytometry, nanoparticle tracking analysis, and resistive pulse sensing. J Thromb Haemost. 2014;12(7):1182-92. [PubMed ID: 24818656]. https://doi.org/10.1111/jth.12602.
-
69.
Cizmar P, Yuana Y. Detection and Characterization of Extracellular Vesicles by Transmission and Cryo-Transmission Electron Microscopy. Methods Mol Biol. 2017;1660:221-32. [PubMed ID: 28828660]. https://doi.org/10.1007/978-1-4939-7253-1_18.
-
70.
Szatanek R, Baj-Krzyworzeka M, Zimoch J, Lekka M, Siedlar M, Baran J. The Methods of Choice for Extracellular Vesicles (EVs) Characterization. Int J Mol Sci. 2017;18(6). [PubMed ID: 28555055]. [PubMed Central ID: PMC5485977]. https://doi.org/10.3390/ijms18061153.
-
71.
Yuana Y, Oosterkamp TH, Bahatyrova S, Ashcroft B, Garcia Rodriguez P, Bertina RM, et al. Atomic force microscopy: a novel approach to the detection of nanosized blood microparticles. J Thromb Haemost. 2010;8(2):315-23. [PubMed ID: 19840362]. https://doi.org/10.1111/j.1538-7836.2009.03654.x.
-
72.
Berne BJ, Pecora R. Dynamic light scattering: with applications to chemistry, biology, and physics. Courier Corporation; 2000.
-
73.
Lawrie AS, Albanyan A, Cardigan RA, Mackie IJ, Harrison P. Microparticle sizing by dynamic light scattering in fresh-frozen plasma. Vox Sang. 2009;96(3):206-12. [PubMed ID: 19175566]. https://doi.org/10.1111/j.1423-0410.2008.01151.x.
-
74.
Dragovic RA, Gardiner C, Brooks AS, Tannetta DS, Ferguson DJ, Hole P, et al. Sizing and phenotyping of cellular vesicles using Nanoparticle Tracking Analysis. Nanomedicine. 2011;7(6):780-8. [PubMed ID: 21601655]. [PubMed Central ID: PMC3280380]. https://doi.org/10.1016/j.nano.2011.04.003.
-
75.
Soo CY, Song Y, Zheng Y, Campbell EC, Riches AC, Gunn-Moore F, et al. Nanoparticle tracking analysis monitors microvesicle and exosome secretion from immune cells. Immunology. 2012;136(2):192-7. [PubMed ID: 22348503]. [PubMed Central ID: PMC3403268]. https://doi.org/10.1111/j.1365-2567.2012.03569.x.
-
76.
Medintz IL, Uyeda HT, Goldman ER, Mattoussi H. Quantum dot bioconjugates for imaging, labelling and sensing. Nat Mater. 2005;4(6):435-46. [PubMed ID: 15928695]. https://doi.org/10.1038/nmat1390.
-
77.
Anderson W, Kozak D, Coleman VA, Jamting AK, Trau M. A comparative study of submicron particle sizing platforms: accuracy, precision and resolution analysis of polydisperse particle size distributions. J Colloid Interface Sci. 2013;405:322-30. [PubMed ID: 23759321]. https://doi.org/10.1016/j.jcis.2013.02.030.
-
78.
Coumans FA, van der Pol E, Boing AN, Hajji N, Sturk G, van Leeuwen TG, et al. Reproducible extracellular vesicle size and concentration determination with tunable resistive pulse sensing. J Extracell Vesicles. 2014;3:25922. [PubMed ID: 25498889]. [PubMed Central ID: PMC4263901]. https://doi.org/10.3402/jev.v3.25922.
-
79.
Tseng D, Mudanyali O, Oztoprak C, Isikman SO, Sencan I, Yaglidere O, et al. Lensfree microscopy on a cellphone. Lab Chip. 2010;10(14):1787-92. [PubMed ID: 20445943]. [PubMed Central ID: PMC2941438]. https://doi.org/10.1039/c003477k.
-
80.
Issadore D, Min C, Liong M, Chung J, Weissleder R, Lee H. Miniature magnetic resonance system for point-of-care diagnostics. Lab Chip. 2011;11(13):2282-7. [PubMed ID: 21547317]. [PubMed Central ID: PMC3115660]. https://doi.org/10.1039/c1lc20177h.
-
81.
Kravets VG, Schedin F, Jalil R, Britnell L, Gorbachev RV, Ansell D, et al. Singular phase nano-optics in plasmonic metamaterials for label-free single-molecule detection. Nat Mater. 2013;12(4):304-9. [PubMed ID: 23314104]. https://doi.org/10.1038/nmat3537.
-
82.
Thery C, Regnault A, Garin J, Wolfers J, Zitvogel L, Ricciardi-Castagnoli P, et al. Molecular characterization of dendritic cell-derived exosomes. Selective accumulation of the heat shock protein hsc73. J Cell Biol. 1999;147(3):599-610. [PubMed ID: 10545503]. [PubMed Central ID: PMC2151184]. https://doi.org/10.1083/jcb.147.3.599.
-
83.
Wei Y, Lai X, Yu S, Chen S, Ma Y, Zhang Y, et al. Exosomal miR-221/222 enhances tamoxifen resistance in recipient ER-positive breast cancer cells. Breast Cancer Res Treat. 2014;147(2):423-31. [PubMed ID: 25007959]. https://doi.org/10.1007/s10549-014-3037-0.
-
84.
Boelens MC, Wu TJ, Nabet BY, Xu B, Qiu Y, Yoon T, et al. Exosome transfer from stromal to breast cancer cells regulates therapy resistance pathways. Cell. 2014;159(3):499-513. [PubMed ID: 25417103]. [PubMed Central ID: PMC4283810]. https://doi.org/10.1016/j.cell.2014.09.051.
-
85.
Wiklander OPB, Brennan MA, Lotvall J, Breakefield XO, El Andaloussi S. Advances in therapeutic applications of extracellular vesicles. Sci Transl Med. 2019;11(492). [PubMed ID: 31092696]. [PubMed Central ID: PMC7104415]. https://doi.org/10.1126/scitranslmed.aav8521.
-
86.
Tang TT, Lv LL, Lan HY, Liu BC. Extracellular Vesicles: Opportunities and Challenges for the Treatment of Renal Diseases. Front Physiol. 2019;10:226. [PubMed ID: 30941051]. [PubMed Central ID: PMC6433711]. https://doi.org/10.3389/fphys.2019.00226.
-
87.
Lu WJ, Liang HB, Li YF, Tu XQ, He JR, Ding KQ, et al. MicroRNA-210-3p Targets RGMA to Enhance the Angiogenic Functions of Endothelial Progenitor Cells Under Hypoxic Conditions. Front Cell Neurosci. 2019;13:223. [PubMed ID: 31164807]. [PubMed Central ID: PMC6536652]. https://doi.org/10.3389/fncel.2019.00223.
-
88.
Zhang L, Yu D. Exosomes in cancer development, metastasis, and immunity. Biochim Biophys Acta Rev Cancer. 2019;1871(2):455-68. [PubMed ID: 31047959]. [PubMed Central ID: PMC6542596]. https://doi.org/10.1016/j.bbcan.2019.04.004.
-
89.
Mallegol J, Van Niel G, Lebreton C, Lepelletier Y, Candalh C, Dugave C, et al. T84-intestinal epithelial exosomes bear MHC class II/peptide complexes potentiating antigen presentation by dendritic cells. Gastroenterology. 2007;132(5):1866-76. [PubMed ID: 17484880]. https://doi.org/10.1053/j.gastro.2007.02.043.
-
90.
Buschow SI, Nolte-'t Hoen EN, van Niel G, Pols MS, ten Broeke T, Lauwen M, et al. MHC II in dendritic cells is targeted to lysosomes or T cell-induced exosomes via distinct multivesicular body pathways. Traffic. 2009;10(10):1528-42. [PubMed ID: 19682328]. https://doi.org/10.1111/j.1600-0854.2009.00963.x.
-
91.
Lundy SK, Klinker MW, Fox DA. Killer B lymphocytes and their fas ligand positive exosomes as inducers of immune tolerance. Front Immunol. 2015;6:122. [PubMed ID: 25852690]. [PubMed Central ID: PMC4367442]. https://doi.org/10.3389/fimmu.2015.00122.
-
92.
Gilligan KE, Dwyer RM. Engineering Exosomes for Cancer Therapy. Int J Mol Sci. 2017;18(6). [PubMed ID: 28538671]. [PubMed Central ID: PMC5485946]. https://doi.org/10.3390/ijms18061122.
-
93.
Pitt JM, Andre F, Amigorena S, Soria JC, Eggermont A, Kroemer G, et al. Dendritic cell-derived exosomes for cancer therapy. J Clin Invest. 2016;126(4):1224-32. [PubMed ID: 27035813]. [PubMed Central ID: PMC4811123]. https://doi.org/10.1172/JCI81137.
-
94.
Syn NL, Wang L, Chow EK, Lim CT, Goh BC. Exosomes in Cancer Nanomedicine and Immunotherapy: Prospects and Challenges. Trends Biotechnol. 2017;35(7):665-76. [PubMed ID: 28365132]. https://doi.org/10.1016/j.tibtech.2017.03.004.
-
95.
Nair S, Salomon C. Extracellular vesicles and their immunomodulatory functions in pregnancy. Semin Immunopathol. 2018;40(5):425-37. [PubMed ID: 29616307]. https://doi.org/10.1007/s00281-018-0680-2.
-
96.
Lai RC, Yeo RW, Lim SK. Mesenchymal stem cell exosomes. Semin Cell Dev Biol. 2015;40:82-8. [PubMed ID: 25765629]. https://doi.org/10.1016/j.semcdb.2015.03.001.
-
97.
Marzio DL, Sass DA. Fulminant Hepatic Failure: Diagnosis and Management. Contemporary Liver Transplantation. Springer; 2016. p. 1-18.
-
98.
Parekkadan B, van Poll D, Suganuma K, Carter EA, Berthiaume F, Tilles AW, et al. Mesenchymal stem cell-derived molecules reverse fulminant hepatic failure. PLoS One. 2007;2(9). e941. [PubMed ID: 17895982]. [PubMed Central ID: PMC1978513]. https://doi.org/10.1371/journal.pone.0000941.
-
99.
Chen L, Xiang B, Wang X, Xiang C. Exosomes derived from human menstrual blood-derived stem cells alleviate fulminant hepatic failure. Stem Cell Res Ther. 2017;8(1):9. [PubMed ID: 28115012]. [PubMed Central ID: PMC5260032]. https://doi.org/10.1186/s13287-016-0453-6.
-
100.
Menke NB, Ward KR, Witten TM, Bonchev DG, Diegelmann RF. Impaired wound healing. Clin Dermatol. 2007;25(1):19-25. [PubMed ID: 17276197]. https://doi.org/10.1016/j.clindermatol.2006.12.005.
-
101.
Cha J, Falanga V. Stem cells in cutaneous wound healing. Clin Dermatol. 2007;25(1):73-8. [PubMed ID: 17276204]. https://doi.org/10.1016/j.clindermatol.2006.10.002.
-
102.
Hu L, Wang J, Zhou X, Xiong Z, Zhao J, Yu R, et al. Exosomes derived from human adipose mensenchymal stem cells accelerates cutaneous wound healing via optimizing the characteristics of fibroblasts. Sci Rep. 2016;6:32993. [PubMed ID: 27615560]. [PubMed Central ID: PMC5018733]. https://doi.org/10.1038/srep32993.
-
103.
Dalirfardouei R, Jamialahmadi K, Jafarian AH, Mahdipour E. Promising effects of exosomes isolated from menstrual blood-derived mesenchymal stem cell on wound-healing process in diabetic mouse model. J Tissue Eng Regen Med. 2019;13(4):555-68. [PubMed ID: 30656863]. https://doi.org/10.1002/term.2799.
-
104.
Poewe W, Seppi K, Tanner CM, Halliday GM, Brundin P, Volkmann J, et al. Parkinson disease. Nat Rev Dis Primers. 2017;3:17013. [PubMed ID: 28332488]. https://doi.org/10.1038/nrdp.2017.13.
-
105.
Teixeira FG, Carvalho MM, Panchalingam KM, Rodrigues AJ, Mendes-Pinheiro B, Anjo S, et al. Impact of the Secretome of Human Mesenchymal Stem Cells on Brain Structure and Animal Behavior in a Rat Model of Parkinson's Disease. Stem Cells Transl Med. 2017;6(2):634-46. [PubMed ID: 28191785]. [PubMed Central ID: PMC5442797]. https://doi.org/10.5966/sctm.2016-0071.
-
106.
Li H, Yahaya BH, Ng WH, Yusoff NM, Lin J. Conditioned Medium of Human Menstrual Blood-Derived Endometrial Stem Cells Protects Against MPP(+)-Induced Cytotoxicity in vitro. Front Mol Neurosci. 2019;12:80. [PubMed ID: 31024252]. [PubMed Central ID: PMC6460823]. https://doi.org/10.3389/fnmol.2019.00080.
-
107.
van Rooij IA, Bancsi LF, Broekmans FJ, Looman CW, Habbema JD, te Velde ER. Women older than 40 years of age and those with elevated follicle-stimulating hormone levels differ in poor response rate and embryo quality in in vitro fertilization. Fertil Steril. 2003;79(3):482-8. [PubMed ID: 12620425]. https://doi.org/10.1016/s0015-0282(02)04839-2.
-
108.
Marinaro F, Pericuesta E, Sanchez-Margallo FM, Casado JG, Alvarez V, Matilla E, et al. Extracellular vesicles derived from endometrial human mesenchymal stem cells improve IVF outcome in an aged murine model. Reprod Domest Anim. 2018;53 Suppl 2:46-9. [PubMed ID: 30238659]. https://doi.org/10.1111/rda.13314.