Abstract
Keywords
Electrospinning Tissue Engineering Biomaterials Nanofibers Regenerative Medicine
1. Context
Electrospinning is a spinning technique that uses electrostatic forces to produce ECM-mimicking fibrous scaffolds from biocompatible polymers. It demonstrates some limitations, such as the usage of toxic solvents, poor cell infiltration, and inhomogeneous cell distribution. To overcome these limitations, a novel method called cell-electrospinning (CE) was introduced. As known, CE is an electrospinning-based technique that generates fibers with living cells embedded within, first introduced by Jayasinghe et al. in 2006 as cited in Hong et al. (1). Electrospinning is the method of spinning fibers using electrostatic forces (2). It is one of the most versatile forms of nanofiber synthesis processes (3). In 1934, Anton Formhals patented his first innovation, a method and equipment for manufacturing artificial filaments with electric charges. In the early 1930s, electrospinning was developed as a feasible fiber-spinning process. This method yields submicron fibers with a high surface-to-volume ratio, tunable porosity, and the capacity to modify nanofiber composition (4). The application of electricity directly causes drying in electrospinning, resulting in less energy loss. Other drying technologies, such as hot melt extrusion (HME) and spray drying, are less environmentally friendly. Another advantage of ES is that its products have morphologies that are very similar to the extracellular matrix of the body, making them potentially ideal for tissue engineering applications (5). Engineering and biological expertise are used in the fascinating field of tissue engineering (TE) to generate or repair tissue and organs. Cells, biomaterials, and biomolecules are its three primary means of operation. A quick and adaptable method called electrospinning creates scaffolds made of nano- and micro-fibers. These scaffolds have important uses in regenerative medicine because they provide a suitable environment for cellular growth (6). This review article describes the use of CE in regenerative medicine based on current research, the introduction of biomaterials, and the many types of cells that are used in this procedure.
2. Cell Electrospinning
Due to its benefits, electrospinning has generated much attention in biomedical applications. This approach has several drawbacks, including the use of hazardous solvents, inadequate cell penetration, and uneven cell dispersion. To get over these restrictions, the CE approach was developed (1, 7). This approach has been investigated for directly manufacturing fibers and scaffolds from a cell suspension, including a broad variety of cells, such as immortalized, primary, stem cells (including iPS cells), and complete organisms. This method is the most advanced technique for creating cell-laden fibers and scaffolds (8).
Electrospinning as a viable method for spinning fibers with a tiny diameter goes back to 1934. Formhals devised a method and equipment for spinning synthetic fibers using electric charges (9). Charles L. Norton created a method for producing fibers from viscous solution in 1936. In 1952, Vonnegut and Newbauer created a technique for producing homogeneous droplets with a diameter of 0.1 mm by disintegrating liquids electrically. Baumgarten created the electrospinning of acrylic resin in dimethyl formamide (DMF) solvent to create acrylic fibers in 1971. Hayati hypothesized in 1987 that semi-conductive and insulating liquids form more stable jets than highly conductive liquids. Cell Electrospinning was firstly introduced by Jayasinghe et al. in 2006 as cited in Hong et al., to show the viability of creating physiologically active scaffolds using a modified ES technique (1).
Cell Electrospinning is based on the application of a high voltage through a DC source to a conducting coaxial needle system positioned above a grounded collecting grill or revolving mandrel. The matrix for the cells is composed of a biopolymer with low conductivity and high viscosity, and its qualities sustain the process, resulting in the development of a continuous cell-bearing fiber that, over time, creates a living scaffold or membrane (Figure 1) (10).
Schematic diagram of cell electrospinning processes for production of cell-laden matrices. The scheme shows the formation of an electrospun membrane with cells encapsulated in fibers from a polymer solution in the syringe (11).
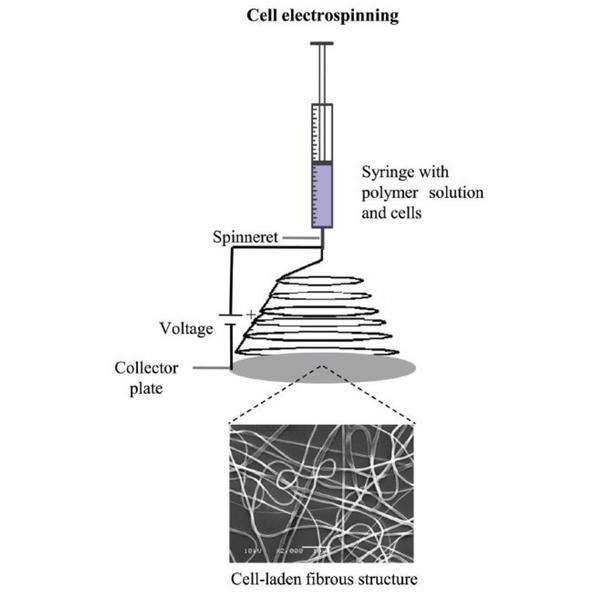
In 2004, Smith et al. as cited in Haider et al. first asserted the use of electrospinning in medicine. To shield or treat potential wounds, they electrospun fibers straight onto the skin's surface to create a mask. In addition, electrospinning fibers have been explored as medication transporters, with encouraging results. Electrospinning nanofibers have also been proven in studies to have a role in enzyme immobilization, wound dressing, and antibacterial effects (12).
Using cell electrospun nanofiber, a number of researchers have tried to regenerate diverse tissues, including skin, blood vessels, tendons/ligaments, and neurons. Nanofibers surrounded by adequate growth factors, cells, or bioactive substances have a significant potential for application in tissue regeneration because they provide cells with the required physical and chemical qualities (13).
A biomedical technique called tissue engineering attempts to produce new organs and tissues for the medical reconstruction of damaged areas. The device is a sophisticated biosystem made of biomaterials that serve as scaffolds and have cell cultures growing on their surface. The ease of preparation and the extent to which they structurally, chemically, and mechanically resemble the extracellular matrix (ECM) are two important benefits of electrospun fibers (14). Frequently, scaffolds used in tissue engineering are "bioabsorbable," which means that when the fibers progressively break down, they serve as a template that is gathered over time by host cells (5). Scaffolds are crucial in regenerative medicine because they provide cells the support they need to carry out their typical duties. The phenotypic and genotype of cells alter in the absence of an appropriate scaffold. The CE method achieves the necessary objective of tissue engineering by immediately constructing an integrated cell-scaffold structure (15).
The molecular weight of the polymer is one of several variables that might impact electrospinning. These include the applied electrical voltage, the operating distance between the spinner and the collector, the motion of the grounded target, and ambient variables (temperature, humidity, and airspeed) (13).
The wide range of electrospinning polymers and solvents makes it difficult to establish the "gold standard" settings that are suitable for every operation. Changes in circumstances may lead to the creation of several fibers with various basic characteristics for any given polymer-solvent system. The intensity of the electric field during spinning is a crucial element in the production of nanoscale fibers. By directly changing the polymer flow rate, field strength has a direct impact on the diameter and shape of fibers (14).
Strong electric fields cause highly conductive solutions to become very unstable, resulting in fibers that deviate significantly from the standard diameter. Low conductivity solutions are frequently used to generate fibers with less uniform diameter distributions. Utilizing extremely volatile solvents fills the surrounding environment with vapor, creating a porous surface (14).
In order to create electrospun nanofibers with desired physicochemical features, several nanofiber synthesis procedures may sequentially alter the polymer viscosity, applied voltage, operating distance, or flow rate while retaining other parameters (13). The viscosity of the bioink, the applied electric field, the pace at which the bioink is fed, the distance from the nozzle tip to the collector, and environmental conditions are some of the factors affecting CE (16). When employing CE to create cell-loaded fibers, the viscosity and surface tension of the printing solution or bioink are crucial. Kim et al. as cited in Hong et al. found in an experiment that there was a substantial decrease in cell viability when the collagen content of bioink was more than 7% by weight (1).
The electric field is one of the most crucial variables in ES-based approaches, as already noted. Since CE involves the creation of cell-embedded fibers, the electric field strength in CE is also significant and must be taken into account to ensure that both fiber production and cell viability are not diminished. Strong electric fields have been shown to result in low cell viability (1). In research by Yeo and Kim, the electric field range of 0.05 - 0.075 kV/mm produced the best cell viability (90%). However, when the electric field intensity grew, cell viability drastically decreased (17). Weak electric fields, on the other hand, may result in improper fiber production. Despite good cell survival (90%) in another investigation utilizing a modest electric field, the microfibers generated were not well-formed (18). The gap between the nozzle's tip and the collector affects the electric field. The flow rate of the solution is another factor that affects the CE procedure. Both fibrogenesis and cell viability depend heavily on flow rate. Since it is directly connected to shear stress, the material should be taken into consideration (1).
Temperature and humidity are only two variables that may have an impact on the CE process. The rheological characteristics of the bioink, such as viscosity and elasticity, may be impacted by temperature. The materials are sometimes thermally cross-linked using temperature, as well. According to studies, lower viscoelasticity and surface tension together have an impact on fiber shape (19). To avoid harm to the cells throughout the CE procedure, the temperature must be properly adjusted. Humidity is a significant factor affecting fiber development. Because high humidity causes the initial jet to elongate further, it causes beaded fibers. Beads occur between portions of thin strands during electrospinning at high humidity (1).
Cell electrospun fibers are nanomaterials and nanofibers that replicate the ECM structure and have a broad variety of therapeutic uses in the area of regenerative medicine. The capacity to create high cellular density, infiltration, and more even cell dispersion are further benefits of CE that may aid in the formation of functional connections between cells (20).
Despite being an acknowledged leader in biotechnology, CE has drawbacks and limits. Because the CE technique employs hydrogels to encapsulate cells in the fibers, the final structure could not have a high mechanical strength. Due to the whipping phenomenon that takes place during the manufacture of fibers, it is challenging to accomplish fiber deposition at the
precise site (1).
Application of Electrospinning and Cell Electrospinning
Application | Technique |
---|---|
Direct alive scaffold preparation | Cell electrospinning |
Drug and bioactive molecules enriched scaffold with elevated mass transport property | Cell electrospinning |
Electrospun nanofibers for wound healing and wound dressing | Electrospinning |
Cell-free nanofiber for tissue engineering | Electrospinning |
3. Biomaterials for Cell Electrospinning
Due to their great processability, several synthetic polymers, including polymethyl methacrylate, polycaprolactone (PCL), and polylactic acid, were transformed into fibrous architectures by ES. Alginate, collagen, and cellulose are just a few natural polymers that are employed in the ES process. These polymers have limits in electrospunability because of weak molecular chain entanglement or the repulsive attraction between ions while being biocompatible and hydrophilic (21, 22).
Natural biomaterials offer significant benefits over synthetic polymers for bioink. Bioactive signals from natural polymers improve cellular activity. A variety of natural polymers or mixtures of them have been used as alternatives for synthetic materials. These comprise collagen and gelatin, as well as alginate with poly (ethylene oxide) (PEO) and lecithin composition for bone regeneration (1).
4. Different Cells Used in Cell Electrospinning
CE has been employed with different biomaterials and techniques to create cell-laden fibers for the regeneration of diverse tissues in order to offer optimal micro- or nano-environments. This section will provide a quick overview of some of the researchers' efforts to use CE to recreate various tissues.
5. Bone Cells
Allogeneic bone regeneration therapy has gained much interest in recent years owing to the drawbacks and risks of autologous bone grafting and the significance of bone tissue regeneration in bone abnormalities such as infections, osteonecrosis, and cartilage disorders. Indeed, the surface-to-volume ratio and ECM-like environment needed to create bone-regenerating scaffolds cannot be achieved with 3D printing (1). Yeo and Kim used 3D printing technology with CE for the first time in 2015 to finish a high-strength 3D mechanical structure and an ECM-like structure for bone repair (18). In 2021, Das et al. created a bone tissue engineering construct using CE technology and a biopolymer of polyglutamic acid. Their technique of cell culturing produced healthy, functioning cells (23).
6. Stem Cells
To regenerate tissue using stem cells, ECM-like microenvironments must be created. Using ASCs and CE, Chen et al. produced a bioactive membrane. In this work, ASCs in a cell culture plate were used to measure the cellular activity of ASCs on electrospun fibers (1, 20, 24).
7. Muscle Cells
Nobody can deny the significant function of human muscles, whether they are smooth, skeletal, or cardiac. In 2014, Ehlar and Jayasinghe employed CE to restore old or injured heart tissue using three-dimensional cardiac patches and collagen-rich myocytes. After CE, the cell viability was somewhere about 80% (25).
For both the CE and cell printing processes, the same bioink—alginate/PEOE embedded with C2C12 cells (mouse myoblast cell line)—was employed. When cell morphology was examined on day 7, it was found that the electrospinning technique's cells were more aligned and elongated than those on the printed structure. The scaffold markedly increased the production of sarcomeric-actinin and myosin heavy chains. This demonstrates controllability in micro- or nanotopological cues as well as compatibility with various muscle cells (1, 17).
8. Application of Cell Electrospinning in Regenerative Medicine
By delivering support structures, cells, and chemical and mechanical signals for regeneration to the necessary location, tissue engineering strives to build new tissues and organs for the therapeutic regeneration of damaged persons (26, 27). A tissue engineering structure is a complex biosystem composed of a biomaterial scaffold that has been strategically oriented and supports the growth of cell cultures (28, 29). Electrospun fibers stand out among other scaffolds used in tissue engineering for two key reasons. First, they are simple to manufacture, and second, they more closely resemble the extracellular matrix in terms of structure (14). The designed structure should facilitate oxygen and nutrient circulation, as well as the removal of metabolic waste, during tissue regeneration in addition to imitating the extracellular matrix (30, 31).
There are a number of techniques for tissue engineering that may be divided into two categories: Jet-based and non-jet-based. Inkjet printing (IJP), laser-guided cell writing (LCD), and bio-electrosprays are examples of jet-based techniques enabling the direct engineering of live organs (bio-electrospinning). Cryo-electrolysis, electrophysiology, and stem cell treatment are examples of non-jet-based methods for direct engineering of living organs (32).
Living cells may now be incorporated into fibrous scaffolds without a separate cell seeding procedure, thanks to the development of CE technology, which creates fibrous scaffolds using electrostatic force. The cells are evenly dispersed, survive well, and spread across the fibrous scaffolds in this process. Multiple three-dimensional shapes are created using CE (33).
The creation of artificial tissue, which can meet the expanding need for tissue replacement, rejuvenation, and repair, includes electrospinning as a key stage. Numerous studies have shown electrospinning's capacity to directly create tissues, which is crucial for both clinical and regenerative medicine. The creation of a 3D multicellular culture using this technique opens up the possibility of using biotechnology to directly produce artificial tissue that has already been developed and planned (34).
CE cardiac patches were used by Ehlar et al. in 2014 for heart tissue engineering. It offered a potentially effective method for regenerating, replacing, and repairing cardiac tissues. The original cardiac myocytes used in these research projects were directly processed utilizing CE to create live heart fibers and scaffolds (25).
In order to stimulate cell development, Chen et al. created a membrane structure that may resemble the natural microenvironment. This accomplishment held up the potential of using CE to replace soft tissues as part of regenerative therapy. This membrane created a new intercellular matrix, boosted the capacity of cells to attach to the membrane, and helped cells preserve phenotypic morphology in addition to giving cells room to develop (20).
In a study, myoblast cells with CE application were compared in vitro, focusing on various cell activities such as cell survival, morphology, and differentiation. High cell viability was achieved with both procedures, but CE significantly enhanced cell elongation, alignment, and differentiation as a result of the aligned fibers. As a result, it was demonstrated that the CE procedure holds great promise for regenerating muscle tissue (17).
A bone tissue engineering structure was created in 2021 by Das et al. utilizing the polyglutamic acid biopolymer and CE technology. Results indicated that the cells in this experiment maintained their functionality and had a high vitality, as well as the capacity to multiply and differentiate. This technique may be used to correct large-area deficiencies in patients who have special arthroplasty, pseudoarthrosis, or tumor removal procedures (23).
9. Conclusions
In this article, we became familiar with the CE method as a new technology. Its benefits and drawbacks were also brought to our attention, and some of its uses in different types of tissue engineering during the last several years were examined. However, some obstacles need to be addressed before CE can be used on a large scale or in different forms of tissue engineering. These obstacles are likely to be overcome in the near future.
References
-
1.
Hong J, Yeo M, Yang GH, Kim G. Cell-electrospinning and its application for tissue engineering. Int J Mol Sci. 2019;20(24). [PubMed ID: 31835356]. [PubMed Central ID: PMC6940787]. https://doi.org/10.3390/ijms20246208.
-
2.
Subbiah T, Bhat GS, Tock RW, Parameswaran S, Ramkumar SS. Electrospinning of nanofibers. J Applied Polymer Sci. 2005;96(2):557-69. https://doi.org/10.1002/app.21481.
-
3.
Teo WE, Ramakrishna S. A review on electrospinning design and nanofibre assemblies. Nanotechnology. 2006;17(14):R89-R106. [PubMed ID: 19661572]. https://doi.org/10.1088/0957-4484/17/14/R01.
-
4.
Bhardwaj N, Kundu SC. Electrospinning: A fascinating fiber fabrication technique. Biotechnol Adv. 2010;28(3):325-47. [PubMed ID: 20100560]. https://doi.org/10.1016/j.biotechadv.2010.01.004.
-
5.
Dziemidowicz K, Sang Q, Wu J, Zhang Z, Zhou F, Lagaron JM, et al. Electrospinning for healthcare: Recent advancements. J Mater Chem B. 2021;9(4):939-51. [PubMed ID: 33367446]. https://doi.org/10.1039/d0tb02124e.
-
6.
Braghirolli DI, Steffens D, Pranke P. Electrospinning for regenerative medicine: A review of the main topics. Drug Discov Today. 2014;19(6):743-53. [PubMed ID: 24704459]. https://doi.org/10.1016/j.drudis.2014.03.024.
-
7.
Najafi R, Asadi A, Zahri S, Abdolmaleki A. [Fabrication and Evaluation of Silymarin-Enriched Tragacanth/Polycaprolactone Nanoscaffold Containing Dental Cells for Use in Tissue Engineering]. J Isfahan Med School. 2023;41(714):226-33. Persian.
-
8.
Jayasinghe SN. Cell electrospinning: A novel tool for functionalising fibres, scaffolds and membranes with living cells and other advanced materials for regenerative biology and medicine. Analyst. 2013;138(8):2215-23. [PubMed ID: 23457706]. https://doi.org/10.1039/c3an36599a.
-
9.
Sill TJ, von Recum HA. Electrospinning: Applications in drug delivery and tissue engineering. Biomaterials. 2008;29(13):1989-2006. [PubMed ID: 18281090]. https://doi.org/10.1016/j.biomaterials.2008.01.011.
-
10.
Sampson SL, Saraiva L, Gustafsson K, Jayasinghe SN, Robertson BD. Cell electrospinning: An in vitro and in vivo study. Small. 2014;10(1):78-82. [PubMed ID: 23894081]. https://doi.org/10.1002/smll.201300804.
-
11.
Maurmann N, França FS, Girón J, Pranke P. Cell Electrospinning: A Review of Materials and Methodologies for Biofabrication. Advanced Biology. 2023;7(10):2300058.
-
12.
Haider A, Haider S, Kang I. A comprehensive review summarizing the effect of electrospinning parameters and potential applications of nanofibers in biomedical and biotechnology. Arabian J Chemistry. 2018;11(8):1165-88. https://doi.org/10.1016/j.arabjc.2015.11.015.
-
13.
Kumbar SG, James R, Nukavarapu SP, Laurencin CT. Electrospun nanofiber scaffolds: Engineering soft tissues. Biomed Mater. 2008;3(3):34002. [PubMed ID: 18689924]. https://doi.org/10.1088/1748-6041/3/3/034002.
-
14.
Inozemtseva OA, Salkovskiy YE, Severyukhina AN, Vidyasheva IV, Petrova NV, Metwally HA, et al. Electrospinning of functional materials for biomedicine and tissue engineering. Russian Chemical Rev. 2015;84(3):251-74. https://doi.org/10.1070/rcr4435.
-
15.
Townsend-Nicholson A, Jayasinghe SN. CHAPTER 4. Cell Electrospinning and Technology Transfer from Lab to Market Scale. Electrospinning. 2018. p. 79-94. https://doi.org/10.1039/9781788012942-00079.
-
16.
Abdolmaleki A, Karimian A, Asadi A, A. Ghanimi H. [3D bioprinting applications as new technology for nerve regeneration]. Zahedan J Res Med Sci. 2023;25(2). Persian. https://doi.org/10.5812/zjrms-121121.
-
17.
Yeo M, Kim GH. Anisotropically aligned cell-laden nanofibrous bundle fabricated via cell electrospinning to regenerate skeletal muscle tissue. Small. 2018;14(48). e1803491. [PubMed ID: 30311453]. https://doi.org/10.1002/smll.201803491.
-
18.
Yeo M, Kim G. Fabrication of cell-laden electrospun hybrid scaffolds of alginate-based bioink and PCL microstructures for tissue regeneration. Chemical Engineering J. 2015;275:27-35. https://doi.org/10.1016/j.cej.2015.04.038.
-
19.
Ding B, Kim H, Lee S, Lee D, Choi K. Preparation and characterization of nanoscaled poly(vinyl alcohol) fibers via electrospinning. Fibers and Polymers. 2002;3(2):73-9. https://doi.org/10.1007/bf02875403.
-
20.
Chen H, Liu Y, Hu Q. A novel bioactive membrane by cell electrospinning. Exp Cell Res. 2015;338(2):261-6. [PubMed ID: 26297530]. https://doi.org/10.1016/j.yexcr.2015.08.007.
-
21.
Karimian A, Khoshnazar SM, Kazemi T, Asadi A, Abdolmaleki A. Role of secretomes in cell-free therapeutic strategies in regenerative medicine. Cell Tissue Bank. 2023. [PubMed ID: 36725732]. https://doi.org/10.1007/s10561-023-10073-5.
-
22.
Najafi R, Asadi A, Zahri S, Abdolmaleki A. Comparison of Biocompatibility and Morphology of PC12 Cell Line on a Polycaprolactane/Silymarin Scaffold and a Polycaprolactane/Tragacanth Scaffold. Gene, Cell Tissue. 2023;10(4). https://doi.org/10.5812/gct-131955.
-
23.
Das P, Hore A, Ghosh A, Datta P. Bone tissue engineering construct fabricated using a cell electrospinning technique with polyglutamic acid biopolymer. J Polymer Res. 2021;28(7). https://doi.org/10.1007/s10965-021-02612-z.
-
24.
Aran S, Zahri S, Asadi A, Khaksar F, Abdolmaleki A. Hair follicle stem cells differentiation into bone cells on collagen scaffold. Cell Tissue Bank. 2020;21(2):181-8. [PubMed ID: 32016616]. https://doi.org/10.1007/s10561-020-09812-9.
-
25.
Ehler E, Jayasinghe SN. Cell electrospinning cardiac patches for tissue engineering the heart. Analyst. 2014;139(18):4449-52. [PubMed ID: 25058315]. https://doi.org/10.1039/c4an00766b.
-
26.
Abbaszadeh S, Asadi A, Zahri S, Abdolmaleki A, Mahmoudi F. Does phenytoin have neuroprotective role and affect biocompatibility of decellularized sciatic nerve scaffold? Gene, Cell and Tissue. 2020;8(1). https://doi.org/10.5812/gct.108726.
-
27.
Abdolmaleki A, Ghayour M, Zahri S, Asadi A, Behnam-Rassouli M. [Preparation of acellular sciatic nerve scaffold and it’s mechanical and histological properties for use in peripheral nerve regeneration]. Tehran Univ Med J TUMS Publications. 2019;77(2):115-22. Persian.
-
28.
Ghayour MB, Abdolmaleki A, Fereidoni M. [Role of extracellular matrix in peripheral nerve regeneration process]. Razi J Med Sci. 2015. Persian.
-
29.
Abdolmaleki A, Asadi A, Taghizadeh Momen L, Parsi Pilerood S. [The role of neural tissue engineering in the repair of nerve lesions]. Neuroscience J Shefaye Khatam. 2020;8(3):80-96. Persian. https://doi.org/10.29252/shefa.8.3.80.
-
30.
Rahmati M, Mills DK, Urbanska AM, Saeb MR, Venugopal JR, Ramakrishna S, et al. [Electrospinning for tissue engineering applications]. Progress in Materials Sci. 2021;117. Persian. https://doi.org/10.1016/j.pmatsci.2020.100721.
-
31.
Abdolmaleki A, Ghayour MB. [The importance of fibronectin in development, regeneration and treatment]. Tehran Univ Med J. 2015;73(5). Persian.
-
32.
Jayasinghe SN, Irvine S, McEwan JR. Cell electrospinning highly concentrated cellular suspensions containing primary living organisms into cell-bearing threads and scaffolds. Nanomedicine (Lond). 2007;2(4):555-67. [PubMed ID: 17716138]. https://doi.org/10.2217/17435889.2.4.555.
-
33.
Mamidi N, Gutiérrez HML, Villela-Castrejón J, Isenhart L, Barrera EV, Elías-Zúñiga A. Fabrication of gelatin-poly(epichlorohydrin-co-ethylene oxide) fiber scaffolds by Forcespinning for tissue engineering and drug release. MRS Communications. 2017;7(4):913-21. https://doi.org/10.1557/mrc.2017.117.
-
34.
Poncelet D, de Vos P, Suter N, Jayasinghe SN. Bio-electrospraying and cell electrospinning: Progress and opportunities for basic biology and clinical sciences. Adv Healthc Mater. 2012;1(1):27-34. [PubMed ID: 23184685]. https://doi.org/10.1002/adhm.201100001.