Abstract
Context:
Coagulation, chemical oxidation and disinfection are essential processes in water and waste treatment. A chemical that can be applied for all the above mentioned purposes is ferrate(VI). Although there are many studies about ferrate(VI), no comprehensive review paper can be found about ferrate(VI) from production to applications. The aim of this study was to review ferrate(VI) production, measurement, stability and utilization in water and wastewater treatment.Evidence Acquisition:
In acidic conditions, the oxidation and reduction capacity of ferrate(VI) is superior to all currently utilized oxidizers and disinfectants in water and wastewater treatment. New researches have provided the technology of using ferrate(VI) for coagulation, chemical oxidation and disinfection of water and wastewater in a reactor simultaneously, which can reduce the size of water and wastewater treatment plants and increase the treatment efficiency.Results:
Despite the existence of these technologies, there is no full-scale application of ferrate(VI) in the water and wastewater industry which it is due to difficulties associated with I, the lack of adequate researches that have demonstrated its capabilities and advantages over the existing water and wastewater treatment methods; ii, the instability of ferrate(VI) depending on its method of preparation, and iii, the relatively low yield of ferrate(VI).Conclusions:
To solve the above mentioned difficulties, fundamental study most be carried out to discover the novel methods of ferrate(VI) production, focusing on increasing the product stability and the production yield.Keywords
1. Context
Ferrate(VI) is one of the oxidized forms of iron, to which serious attention has been recently given due to its extraordinary ability of oxidization. Nowadays, using ferrate(VI) has been introduced as an environmental-friendly method for removal of a wide range of toxic compounds in water. Study of ferrate(VI) seems to be essential due to its high capacity for the removal of organic and inorganic compound that are harmful for the environment. Ferrate(VI) in comparison with other current oxidants is a cheap and low-risk one (1). Three methods have been developed to produce ferrate(VI) including (i) electrochemical method, (ii) thermal method, and (iii) chemical method (2, 3). Analytical methods can also be used to express the capacity and structure of ferrate(VI) species (1, 4, 5). Ferrate(VI) can be used as an oxidizer, coagulant and disinfector in water and wastewater treatment. Ferrate(VI) has been applied for removal of pollutants such as medicines, ammonium, cyanides, sulfides, phosphates, arsenic, estrogens, anilines and phenols (1, 6). Many studies have been carried out on the production of ferrate(VI) between 1950 and 1960. However, the study of ferrate(VI) applications for water and wastewater treatment has been started since the last decade (7, 8). The aim of the present study was to collect all necessary information about i, chemical structure of ferrate(VI); ii, different methods of ferrate(VI) production; iii, ferrate(VI) measurement methods; IV, ferrate(VI) stability; and finally V, applications of ferrate(VI) as an oxidant, disinfectant and coagulant. The collected information is extremely useful for all researchers who would like to study on ferrate applications.
2. Evidence Acquisition
2.1. Iron Oxidation States
As we know, iron is among the most important elements in nature which is observed in free form (zero-valent iron) or in any other form with various oxidation values, presented in Table 1.
Different States of Iron Oxidation
No. | Compound | Name |
---|---|---|
1 | FeO | Ferrous oxide |
2 | Fe2O3 | Ferric oxide |
3 | Fe3O4 | Ferrosoferric oxide |
4 | Fe2O3.H2O | Ferric oxide monohydrate |
5 | FeOOH | Ferric oxyhydroxide |
6 | FeO22- | Hypoferrite |
7 | FeO2- | Ferrite |
8 | FeO32- | Ferrate (IV) |
9 | FeO44- | Ferrate (IV) |
10 | FeO43- | Ferrate (V) |
11 | FeO42- | Ferrate(VI) |
12 | FeO52- | Ferrate (VIII) |
Iron and various oxidation states of it can be considered for different applications, for example in the production of magnetic pigments, catalysts, and magnetic fluids. Amorphous iron oxides (formless) possess significant applicability in industries as well as water purification technology. In recent years, iron oxides in form of nanoparticles have shown unique properties in many applications of technology. Iron nanoparticles and iron oxides in combination with oxygen and hydrogen peroxide have shown promising results in oxidation of oxidation-resistant compounds. In addition to the three stable iron oxidation states of 0, +2 and +3, ascendance into higher oxidation states can also be observed in an environment of strong oxidizing capacity (for example +8, +6, +5, +4, etc.). These iron oxidation states are known as ferrates. Among ferrates, the +6 oxidation state is relatively stable and easier to synthesize in comparison to the rest (9, 10). It should be noted that the activities of the oxidation states of +4 and +5 are higher than the +6 state (7, 11). The application of ferrates in elimination of emerging pollutants such as arsenic, estrogen and released drugs into the environment has been well recognized. Ferrates are recently being applied as a green chemical compound in synthesizing the aforementioned organic compounds as well as the elimination of toxic waste from water (4, 5). As a result, ferrates have a high potential capacity in addressing major environmental issues (8, 12, 13).
2.2. Chemical Structure of Hexavalent Iron
The X-ray spectroscopy studies on a solid crystal like K2FO4 suggested that ferrate(VI) is shaped from four oxygen atoms which establish a covalent bond with a central iron atom with an oxidation number of +6 (5, 14). The tetrahedral structure of ferrate(VI) is confirmed through oxygen isotopic studies in an aqueous solvent and suggests that four oxygen atoms surround the iron atom (15). Other researches have demonstrated that ferrate(VI) ion can have three resonance hybrid structures with the atoms of oxygen. Structures 2 and 3 are considered as the primary structures (Figure 1) (1, 16).
Three Resonance Hybrid Structures of Ferrate(VI) Ion in an Aqueous Solution
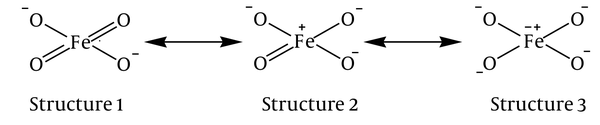
Recent spectroscopic and kinetic studies have suggested that four types of hexavalent iron in aqueous solutions exist through the balancing of acid and alkali (17). Figure 2 presents the various hexavalent irons as a function of pH. Figure 2 suggests that HFeO4- and FeO42- are dominant forms in nature, while ferrate(VI) as the dominant form in alkaline environments converts into Fe(III) (18).
Specious Distribution of Ferrate(VI) in Aqueous Solutions Within Different pHs (19)
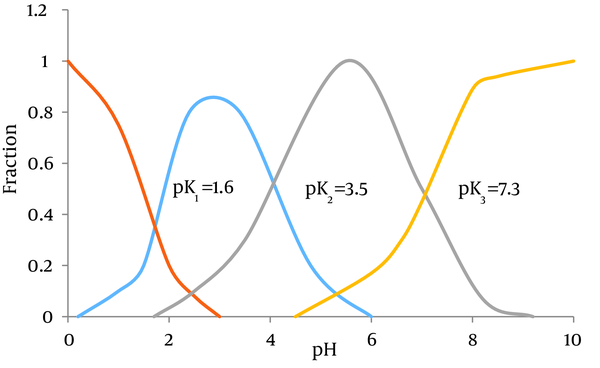
3. Results
3.1. Production of Ferrate(VI)
For the first time in 1702, Stahl noticed a red-purple color during the explosive experiments which was created from a mixture of potassium nitrate and iron filings. The produced substance was eventually identified as hexavalent potassium ferrate (K2FeO4). Eckenberg and his colleague Becquerel observed the same color in 1834 while heating a mixture of potassium hydroxide and iron ore. Later in 1840, Fremy formed the hypothesis that this color was caused by a type of high-capacity iron with the chemical formula of FeO32-. Despite these advances, the principles of hexavalent iron production began 100 years ago (9). Generally, there are three ways to produce hexavalent iron: I, dry oxidation method by heating or melting the iron oxides in the presence of oxygen in an environment with high alkalinity; ii, electrochemical method using iron or its alloys as the anode electrode and an electrolyte solution of NaOH or KOH; iii, wet oxidation method by oxidation of trivalent iron salt at high alkalinity and in the presence of chlorine or hypochlorite.
3.1.1. Ferrate(VI) Production Using the Dry Oxidation Method
Dry oxidation is an old method for the production of ferrate(VI). This method is generally believed to be dangerous and difficult, since increasing the temperature highly increases the risk of explosion (10). Today, several attempts have been made to address this issue by many researchers. For example, potassium and sodium of ferrate(VI) are produced by calcification of potassium and sodium peroxide at 350°C - 370°C. The oxidation of iron oxide by sodium peroxide at a temperature of 370°C in the presence of oxygen and absence of carbon dioxide can also lead to ferrate(VI) production. The result of the two aforementioned methods is the production of FeO54- containing compounds that immediately hydrolyze into FeO42- or tetrahedral ions upon dissolution in water while adopting a red-purple color.

The production of pentavalent iron from galvanized waste was also introduced by Cici and Cuci in 1998. Their innovative method involved heating the galvanized waste along with iron oxide in a furnace up to a temperature of 800°C (12). The heated galvanized waste and iron oxide in combination with sodium peroxide was then melted and immediately cooled to produce sodium ferrate(VI) according to the following equation:

In another method, the potassium and cesium ferrates (VI) could be produced through the reactions of potassium and cesium super oxides with iron oxide powder in high temperatures of about 200°C for 10 hours (17).
3.1.2. Ferrate(VI) Production by Electrochemical Method
Based on the conducted research, there are various methods to synthesize ferrate(VI) including the thermal, chemical and electrochemical methods (20-23). Thermal methods often require high temperatures (About 800°C) and usually possess a low efficiency of about 50% (2). Chemical methods are also multiphase and require high amount of chemical compounds (3, 24, 25). Electrochemical method in comparison to the other two mentioned methods possess advantages such as the purity of the product, low demand for solvents, and the use of one electron which is known as the clean oxidant (Figure 3) (5, 25, 26).
Schematic Representation of the Electrochemical Cell for the Synthesis of Ferrate(VI)
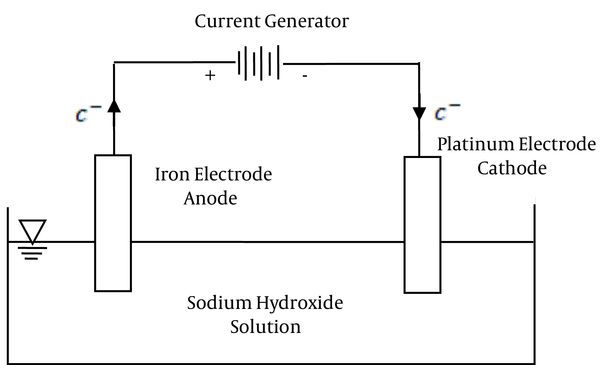
The first electrochemical ferrate(VI) synthesis was carried out in 1841, which is one of the easiest ways to obtain sodium ferrate from solutions with no impurities (27). This synthesis involves the oxidation of raw iron or one of the iron salts in an alkaline solutions by using table salt as a stabilizer. The researchers then conducted several experiments in different alkaline environments with various NaOH concentrations, different current densities, temperature, and electrolysis intervals. Test results suggested that the implementation of iron oxidation is dependent on current density, temperature and electrolysis interval (28, 29). It was revealed that increasing the temperature would increase the oxidation efficiency. In fact, we can say that temperature increase can act as a catalyst, which is only valid to a certain point. For example, in some cases, the maximum allowed temperature was 60°C. Increasing the electrolysis interval can also increase the interval for reaction, which would ultimately increase the efficiency (30). Basic principles of ferrate(VI) are shown in the following equations:
Anode Reaction:

Cathode Reaction:

General Reaction:


The intensity of the electric current, the anode electrode material, and the type and concentration of the electrolyte significantly affect the efficiency of ferrate(VI) production. The highest ferrate(VI) production rate was achieved at the flow rate of 3 mA/cm2 and electrode temperature of 40°C, which is equivalent to 40%. Greater amounts of carbon in anode electrode can also increase the production of ferrate(VI). Efficiencies above 70% can be achieved by utilizing iron and silver electrodes containing 0.9% carbon. This is while the efficiency of the flow rate using the same electrode containing only 0.08% carbon amounted to only 12%. The production of ferrate(VI) using iron electrode with purity of 99.99% and temperatures of 30 - 60°C using the AC current was also reported. These test results demonstrated that the maximum efficiency of this system for ferrate(VI) production was equivalent to 43%, achieved at the current density of 88 mA/cm2 and the frequency of 50 Hz under the temperature of 40°C.
In 1901, Haber and Pick (cited in Macova et al.) observed the positive effects of increasing the concentration of hydroxide ion in the synthesis of ferrate(VI) (6). Other researches involving electrolytes such as NaOH, KOH and LiOH revealed that NaOH provided the best condition for ferrate(VI) production (19). Bouzek et al. also reported that the optimal concentration in case of using NaOH as electrolyte was 14 molar (31-33).
Another example is the production of BaFeO4 directly by the electrochemical method. This method uses the oxidation of an iron wire as the anode electrode in a common electrolyte of NaOH/Ba(OH)2. Throughout the electrolysis, the concentration of BaFeO4 increases (8). The produced BaFeO4 can be filtered to separate it from electrolyte and then it must be rinsed three times with distilled water. The above mentioned method is known as direct electrochemical method. The direct electrochemical method for the production of ferrate(VI) provides several advantages including: i, easy ferrate(VI) synthesis in one step; ii, in this method, due to the formation of solid samples, the instability of ferrate(VI) can be avoided; and iii, no chemical oxidants are used to synthesize ferrate(VI) (8).
In another experiment for direct K2FeO4 synthesis, a metal delicate lace was used in KOH electrolyte. The resulting product was synthesized with an efficiency of 73.2% and purity grade of 95.3% - 98.1% (34-36). Later studies revealed that ferrate(VI) production efficiency was also dependent on the concentration of electrolyte (37).
3.1.3. Ferrate(VI) Production by Wet Oxidation Method
The wet oxidation method involves oxidizing a solution containing trivalent iron with high alkalinity (in the presence of NaOH) and conversion into ferrate(VI). Since the solution containing ferrate(VI) is degraded rapidly, it is necessary to apply the sequestration, washing and drying processes for a more stable product (38, 39). Several efforts have been made to produce sodium ferrate(VI). Although, there are still many problems in isolating and obtaining dry product from the corresponding solution, due to the high solubility of Na2FeO4 in NaOH-saturated solution. By modifying the production procedure in which the chlorine gas is passed through trivalent iron salt solution, saturated by NaOH, one can obtain a dry compound containing 41.38% of Na2FeO4 (40).
The production of potassium ferrate(VI) by wet oxidation method has been studied since 1950 (41). The production of this compound initially involved obtaining of potassium ferrate from the ferric chloride reaction with sodium hypochlorite (NaCLO) in the presence of sodium hydroxide which in turn effectuates the sequestration of potassium ferrate from the solution by adding potassium hydroxide. The corresponding reactions are presented below:



Even though the production rate of potassium ferrate is low in this method, the produced potassium can still possess a purity of about 96%. The potassium ferrate production rate was investigated by Schreyer et al. and also Williams and Riley, in which potassium hydroxide was used instead of sodium hydroxide (32, 42). This replacement prevented the production of sodium ferrate in intermediate reactions. As a result, the production rate of potassium ferrate raised to 75% with purity of 80% - 90%. This method also helped reduce the production and purification interval of potassium ferrate.
3.1.4. A Comparison of Ferrate(VI) Production Methods
Sodium ferrate (Na2FeO4) and potassium ferrate (K2FeO4) are hexavalent irons utilized in the treatment of water and wastewater. Sodium ferrate bears different properties in comparison with other hexavalent irons. This compound has the capacity to remain in dissolved state in a saturated environment by sodium hydroxide. For the purpose of water and wastewater treatment, it is necessary to obtain a dry powder, since ferrate(VI) is unstable. Although, obtaining a sodium ferrate dry powder is highly difficult due to the problems mentioned in earlier sections. Fortunately, the insolubility of potassium ferrate in water in a saturated environment by potassium hydroxide (KOH) allows easy sequestration and isolation from water. This enabled the conditions necessary to produce and store potassium ferrate for long periods of time which could then be utilized in large scale in water and wastewater treatment industry. A number of conducted researches revealed some of the problems associated with producing ferrate(VI). Many considerations have to be taken in the production and purification of ferrate(VI). Although, despite these considerations, the purity of the produced ferrate(VI) is still variable due to other affecting factors. For example, in the electrolysis method, the electrode material could affect the rate and purity of the produced ferrate(VI). Temperature, the retention time and sediments isolation are also affective on ferrate(VI) production in wet oxidation method. As a result, the produced ferrate(VI) is always accompanied by other impurities such as alkali metallic hydroxides, chlorides and ferric oxides. One of the disadvantages of the wet oxidation method is the utilization of hypochlorite, which is associated with the production chlorine gas. Therefore, to obtain a high-quality product, all the steps and conditions of ferrate(VI) production must be closely monitored and controlled.
3.2. Ferrate(VI) Iron Salts Measurement Methods
Researchers have mentioned two methods for measuring ferrate(VI) in aquatic environments which includes the titration and spectrophotometry methods (43). One of the most appropriate methods in measuring ferrate(VI) is its reaction with chromite salt. The following reaction explains the reaction between ferrate(VI) and chromite in an acidic environment (44). In this method, sodium diphenylamine-4-sulfonate is used as an indicator. It should be noted that this method can only be applied for solutions with low ferrate(VI) ion concentrations.

Another way to measure ferrate(VI) is to convert it into Fe(III) in an alkaline environment along with arsenite (45). In this method, a weighed quantity of ferrate(VI) iron salt is added to a solution containing arsenite, in which the concentration must be higher than the required amount for the conversion of ferrate(VI) to Fe(III) (46). The remaining arsenite can then be measured by the bromate and cerate standard solutions. By measuring the concentration of bromate and cerate, the ferrate(VI) ion can then be measured.



A ferrate(VI)-containing solution has a red-purple color with a light absorption rate in wavelengths of 500-800 nm (47). Other studies identified wavelengths such as 505 nm appropriate for the concentration evaluation of ferrate(VI) (42, 48). Among the various methods of spectrophotometry, infrared spectrophotometry (in wavelengths of 324 and 800 cm-1) has received much attention due to both qualitative and quantitative capabilities in ferrate(VI) measurement (49-56).
3.3. The Stability of Ferrate(VI) Iron Salt
When ferrate(VI) iron salts dissolve in water, it causes the release of oxygen and sequestration of iron hydroxide. This property would lead to instability of ferrate(VI) when dissolved in water. The following reaction explains the Fe(III) sequestration when dissolved in water. The production of high quantities of oxygen by ferrate(VI) can be considered as an advantage in utilizing this compound in treating wastewater. It should be noted that the presence of oxygen would prevent anaerobic condition in wastewater, thus inhabiting the production of odor-causing compounds such as hydrogen sulfide. Furthermore, considering the high oxidation capacity of ferrate(VI), the organic and inorganic odor-causing compounds are easily oxidized, thus inhibiting the emission of odor in wastewater facilities.

The decomposition rate of ferrate(VI) in the above reaction is dependent on factors such as I, the initial concentration of the dissolved ferrate(VI) in water; ii, the concentration of other ions present in water; iii, pH of the solution; and iv, the temperature of the environment (55). The complete understanding of the effects of these factors on the decomposition rate of ferrate(VI) is a necessary step in utilizing it in water and wastewater treatment industry. The stability of ferrate(VI) salts dissolved in water in low concentrations is much lower than higher concentrations (57). In a solution containing ferrate(VI) ions in a concentration of less than 0.025 molar, 89% of the ion still remains in the solution after 60 minutes, while in concentrations higher than 0.03 M, the entire ferrate(VI) is removed within the same interval. Another study demonstrated that 79.5% of ferrate(VI) in a solution of potassium ferrate with concentration of 0.01 M was decomposed in 2.5 hours. This is while 37.4% of the 0.0019 M solution was decomposed in three hours and 50 minutes and a temperature of 25°C. To investigate the effect of ions on the stability of ferrate(VI), a 0.5 M potassium ferrate solution was provided along with chloride potassium ion, potassium nitrate, sodium chloride and hydrous ferric oxides, and the decomposition rate of ferrate(VI) was evaluated under 25°C. The test result suggested that ferrate(VI) in the presence of potassium chloride and potassium nitrate had higher decomposition rates in early stages of the experiment, but as the time passed, the decomposition rate decreased with decline in the ferrate(VI) concentration (Figure 4). Nevertheless, the presence of ions such as sodium chloride and hydrous ferric oxides increased the decomposition speed of ferrate(VI). The decomposition of ferrate(VI) declines in the presence of phosphate. The increase in pH or alkalinity of the solution can also increase the stability of this ion. Based on Figure 5, alkalinity can decrease the decomposition rate of ferrate(VI). Adding a 6 M solution of KOH can significantly increase the stability of ferrate(VI) in comparison with the 3 M solution under similar conditions (Figure 5). The ferrate(VI) ion made by buffer solution 8 was more stable than the ferrate(VI) ion made by buffer solution 7. In pH of 7, only 49% of ferrate(VI) remained in the solution after 10 hours (57), while in pH of 8 under similar conditions, ferrate(VI) remained after 10 hours. Temperature is also considered as an important factor in stability of ferrate(VI). High ferrate(VI) stability was observed in a solution in 0.5°C. The solution containing 0.01 M potassium ferrate lost 10% of it ferrate(VI) in two hours in 10°C, while the concentration of ferrate(VI) in the same solution in 0.5°C did not change after two hours. Furthermore, if the solution containing ferrate(VI) is produced under the laboratory temperature (25°C) and cooled in the fridge down to 0.5°C, it will lose 5% of its ferrate(VI) ions before reaching 0.5°C. By considering all these parameters, it is obvious that a minimal temperature and pH higher than 8 is required if a high quality product is wished to be achieved.
Effect of the Presence of Different Ions on the Stability of Ferrate(VI) in Water Solution (38)
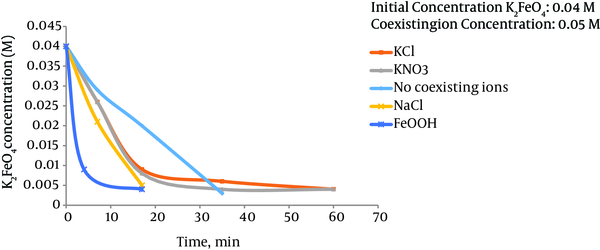
Effect of Alkalinity Increase on Stability of Ferrate(VI) (39)
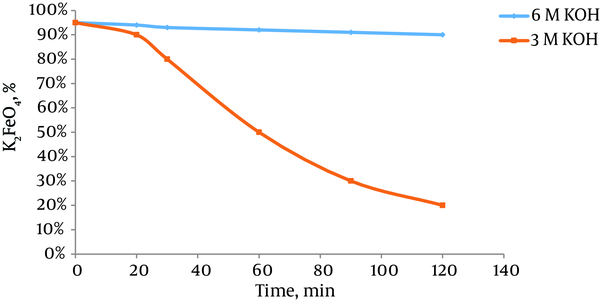
3.4. Applications of Ferrate(VI)
Coagulation, chemical oxidation and disinfection are essential processes in water treatment. Coagulants attach the non-settling suspended particles to each other, forming coarse particles. In some cases, these coarse particles formed by chemical coagulation are capable of adsorbing pollutants in wastewater. These coarse particles would be removed, in turn, through sedimentation or filtration processes. Disinfection process is also designed to eliminate organisms harmful for human health such as bacteria and viruses. In certain cases, the disinfection process is used to remove or control odor ingredients. A wide range of coagulants, oxidizers and disinfectants can be used to treat water or wastewater. The most common coagulant compounds include: ferric sulfate (Fe2(SO4)3), aluminum sulfate (Al(SO4)3) and ferric chloride (FeCl3). Chlorine (Cl2), sodium hypochlorite (NaClO), chlorine dioxide (ClO2) and ozone (O3) are also among the most important oxidizers and disinfectants. Due to the increasing level of water pollution and strict drinking water production and distribution standards, it is necessary to address this issue by identifying new chemical compounds and new standards. Such a compound should ideally possess the following properties: i, the capability to disinfect water; ii, to some extent, the ability to decompose organic and inorganic contaminants in water and wastewater; and iii, the ability to remove suspended particles and heavy metals in water. A substance that can meet all the above criteria is ferrate(VI). Ferrate(VI) is a strong oxidizer with the chemical formula of FeO42-. In acidic conditions, the oxidation and reduction capacity of ferrate(VI) not only exceeds to that of ozone, but is also superior to all currently utilized oxidizers and disinfectants in water and wastewater treatment. In addition, during the oxidation and disinfection processes, this compound is converted into trivalent iron or ferric hydroxide (Fe(OH)3). Since trivalent iron is a strong coagulant, by simultaneously adding ferrate(VI) during one chemical injection, the following three processes could be achieved in one operational unit: I, The oxidation of pollutants in water; ii, water disinfection; and iii, elimination of suspended and colloidal particles. The oxidization and disinfection capacities of commonly used compounds in water and wastewater treatment are presented in Figure 6, which is easily comparable to ferrate(VI). All applications of Ferrate(VI) are shown in Figure 7. Also, all advantages and disadvantages of using ferrate(VI) as oxidant, disinfectant and coagulant are shown in Tables 2 and 3.
Advantages and Disadvantages of Using Different Current Oxidants and Disinfectants
Oxidant and Disinfectant | Advantages | Disadvantages |
---|---|---|
Chlorine (Cl2) | (i) Low cost; (ii) high removal efficiency; (iii) availability; (iv) the possibility of long-term storage of chlorine in cylinders. | (i) The possibility of producing dangerous byproducts such as three halomethanes; (ii) toxicity for water and sewage treatment plant staffs, and (iii) the need to dechlorinate treated wastewater before releasing into environment. |
Ozone (O3) | (i) High capacity and high speed of oxidation and disinfection; (ii) converting of ozone to oxygen after disinfection process; (iii) non-toxic byproducts; (iv) ability of odor and taste removal. | (i) Possibility of remaining few cysts and viruses after disinfection process; (ii) oxidation by ozone requires complex equipment; (iii) the high price of ozone production; (iv) producing of solid byproducts, and (v) corrosion in the equipment of water and wastewater treatment. |
Ferrate(VI) (FeO42-) | (i) Excessive capacity of oxidation and disinfection; (ii) non-toxic byproducts, (iii) ability of colloidal particles coagulation; (iv) ability of coagulation, oxidation and disinfection simultaneously; (v) needing smaller wastewater treatment plant; (vi) low application cost, and (vii) ability of heavy metal and inorganic removal. | (i) Low ferrate(VI) production rate and (ii) lack of stability for long-term storage. |
Advantages and Disadvantages of Using Different Current Coagulants
Coagulant | Advantages | Disadvantages |
---|---|---|
Fe (III) | (i) Low residue after coagulation process; (ii) high efficiency for colloidal particles removal; (iii) effective in pHs from 4 to 6 and 6.8 to 9.2, and (iv) low cost. | (i) Producing non-soluble solids in water and (ii) usually for achieving better performance needs to add alkaline compounds. |
Al3+ | (i) Easy control of the coagulation process; (ii) low sludge production, and (iii) effective in pHs between 6.5 and 7.5 (neutral pH). | (i) Producing non-soluble solids in water and (ii) low range of pH to achieve highest performance. |
Ferrate(VI) | (i) Non-toxic byproducts, (ii) Ability of coagulation, oxidation and disinfection simultaneously; (iii) needing smaller wastewater treatment plant; (iv) low application cost, and (v) ability of heavy metal and inorganic removal. | (i) Low ferrate(VI) production rate and (ii) lack of stability for long-term storage. |
Comparison of Current Disinfectants and Oxidant Ability to Use for Treatment of Water and Wastewater
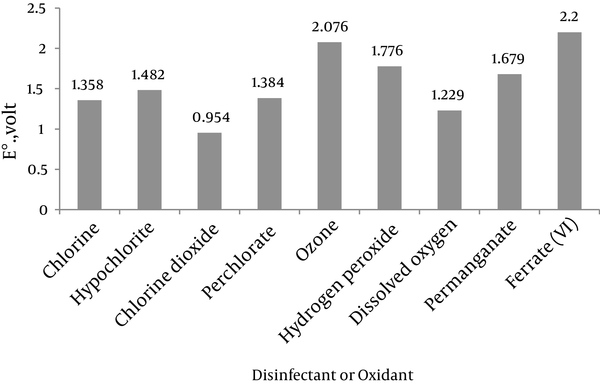
Various Applications of Ferrate(VI) in Water and Wastewater Treatment Technology
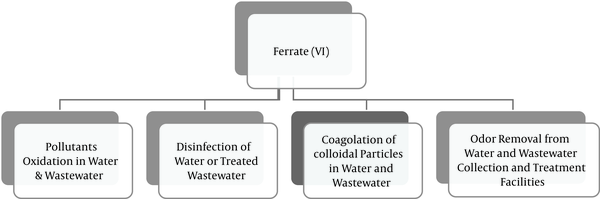
4. Conclusions
The present study showed that ferrate(VI) was a powerful oxidant for the removal of a wide range of organic and inorganic pollutants from water and wastewater. Different methods have been developed to produce ferrate(VI) among which electrochemical methods seem to be suitable for water and wastewater treatment. Since oxidization of pollutants using ferrate(VI) does not produce any toxic and mutagenic byproducts, it is known as a green technology and ferrate(VI) is known as green chemical.
Acknowledgements
References
-
1.
Norcross BE, Lewis WC, Gai H, Noureldin NA, Lee DG. The oxidation of secondary alcohols by potassium tetraoxoferrate(VI). Canad J Chem. 1997;75(2):129-39. https://doi.org/10.1139/v97-017.
-
2.
Bouzek K, RousA I. J Appl Electrochem. 1997;27(6):679-84. https://doi.org/10.1023/a:1018483603666.
-
3.
Madarasz J, Zboril R, Homonnay Z, Sharma VK, Pokol G. Thermal decomposition of iron(VI) oxides, K2FeO4 and BaFeO4, in an inert atmosphere. J Solid State Chem. 2006;179(5):1426-33. https://doi.org/10.1016/j.jssc.2006.01.054.
-
4.
Sharma VK, Kazama F, Jiangyong H, Ray AK. Ferrates (iron(VI) and iron(V)): environmentally friendly oxidants and disinfectants. J Water Health. 2005;3(1):45-58. [PubMed ID: 15952452].
-
5.
Ibanez JG, Tellez-Giron M, Alvarez D, Garcia-Pintor E. Laboratory Experiments on the Electrochemical Remediation of the Environment. Part 6: Microscale Production of Ferrate. J Chem Educ. 2004;81(2):251. https://doi.org/10.1021/ed081p251.
-
6.
Macova Z, Bouzek K, Sharma VK. The role of the electrode and electrolyte composition in the anode dissolution kinetics: Comparison of the ferrate (vi) synthesis in the solutions of naoh and koh of various ratio. In: Sharma VK, editor. Ferrates: Synthesis, properties, and applications in water and wastewater treatment, ACS Symposium Series 985. Washington, DC: American Chemical Society; 2008. p. 52-67.
-
7.
Sharma VK, Yngard RA, Cabelli DE, Clayton B. J. Ferrate(VI) and ferrate(V) oxidation of cyanide, thiocyanate, and copper(I) cyanide. Radiat Phys Chem. 2008;77(6):761-7. https://doi.org/10.1016/j.radphyschem.2007.11.004.
-
8.
Licht S, Yu X. Recent Advances in Fe(VI) Synthesis. In: Sharma VK, editor. Ferrates: Synthesis, Properties, and Applications in Water and Wastewater Treatment, ACS SymposiumSeries. 985. 2008. p. 2-51. https://doi.org/10.1021/bk-2008-0985.ch001.
-
9.
Tsapin A. Iron(VI): Hypothetical Candidate for the Martian Oxidant. Icarus. 2000;147(1):68-78. https://doi.org/10.1006/icar.2000.6437.
-
10.
Kazama F. Viral inactivation by potassium ferrate. Water Sci Technol. 1995;31(5-6):165-8. https://doi.org/10.1016/0273-1223(95)00259-p.
-
11.
Sharma VK, O'Connor DB. Ferrate(V) oxidation of thiourea: a premix pulse radiolysis study. Inorganica Chimica Acta. 2000;311(1-2):40-4. https://doi.org/10.1016/s0020-1693(00)00322-4.
-
12.
Thompson GW, Ockerman LT, Schreyer JM. Preparation and Purification of Potassium Ferrate. VI. J Am Chem Soc. 1951;73(3):1379-81. https://doi.org/10.1021/ja01147a536.
-
13.
Ni X, Ji M, Yang Z, Zheng H. Preparation and structure characterization of nanocrystalline BaFeO4. J Cryst Grow. 2004;261(1):82-6. https://doi.org/10.1016/j.jcrysgro.2003.09.024.
-
14.
Hoppe M, Schlemper E, Murmann R. Structure of dipotassium ferrate (VI). Acta Crystallographica Section B: Structural Crystallography and Crystal Chemistry. 1982;38(8):2237-9.
-
15.
Goff H, Murmann RK. Mechanism of isotopic oxygen exchange and reduction of ferrate(VI) ion (FeO42-). J Am Chem Soc. 1971;93(23):6058-65. https://doi.org/10.1021/ja00752a016.
-
16.
Lee Y, Cho M, Kim JY, Yoon J. Chemistry of ferrate (Fe (VI)) in aqueous solution and its applications as a green chemical. J Ind Eng Chem. 2004;10(1):161-71.
-
17.
Rush JD, Zhao Z, Bielski BH. Reaction of ferrate (VI)/ferrate (V) with hydrogen peroxide and superoxide anion--a stopped-flow and premix pulse radiolysis study. Free Radic Res. 1996;24(3):187-98. [PubMed ID: 8728120].
-
18.
Lee DG, Gai H. Kinetics and mechanism of the oxidation of alcohols by ferrate ion. Can J Chem. 1993;71(9):1394-400. https://doi.org/10.1139/v93-180.
-
19.
Bouzek K, Schmidt MJ, Wragg AA. Influence of electrolyte composition on current yield during ferrate(VI) production by anodic iron dissolution. Electrochemistry Communications. 1999;1(9):370-4. https://doi.org/10.1016/s1388-2481(99)00075-2.
-
20.
Villanueva-Rodriguez M, Hernandez-Ramirez A, Peralta-Hernandez JM, Bandala ER, Quiroz-Alfaro MA. Enhancing the electrochemical oxidation of acid-yellow 36 azo dye using boron-doped diamond electrodes by addition of ferrous ion. J Hazard Mater. 2009;167(1-3):1226-30. [PubMed ID: 19195775]. https://doi.org/10.1016/j.jhazmat.2008.12.137.
-
21.
Denvir A, Pletcher D. Electrochemical generation of ferrate Part I: Dissolution of an iron wool bed anode. J Appl Electrochem. 1996;26(8):815-22. https://doi.org/10.1007/bf00683743.
-
22.
Lescuras‐Darrou V, Lapicque F, Valentin G. J Appl Electrochem. 2002;32(1):57-63. https://doi.org/10.1023/a:1014269523898.
-
23.
Denvir A, Pletcher D. Electrochemical generation of ferrate Part II: Dissolution of an iron wool bed anode. J Appl Electrochem. 1996;26(8):823-7. https://doi.org/10.1007/bf00683743.
-
24.
Ninane L, Kanari N, Criado C, Jeannot C, Evrard O, Neveux N. Ferrates: Synthesis, properties and applications in water and wastewater treatment,.In: Sharma VK, editor. Proceedings of the 2nd ACS National Meeting. San Francisco. American Chemical Society; 2006. 558 p.
-
25.
Perfiliev YD, Benko EM, Pankratov DA, Sharma VK, Dedushenko SK. Formation of iron(VI) in ozonalysis of iron(III) in alkaline solution. Inorg Chim Acta. 2007;360(8):2789-91. https://doi.org/10.1016/j.ica.2006.11.019.
-
26.
Macova Z, Bouzek K, Hives J, Sharma VK, Terryn RJ, Baum JC. Research progress in the electrochemical synthesis of ferrate(VI). Electrochimica Acta. 2009;54(10):2673-83. https://doi.org/10.1016/j.electacta.2008.11.034.
-
27.
Poggendorf JG. Methode zur quantitativen Bestimmung der elektro-motorischen Kraft in konstanter galvanischer Kette. Pogg. Ann. 1841;54:161-91.
-
28.
Grube G, Gmelin H. Der EinflussÜberlagertenWechselstromes auf die AnodischeFerratbildung. Zeits- chriftfürElektrochemie und AngewandtePhysikalischeChemie. 1920;26:153.
-
29.
G G. Die Passivitat der MetallebeiAnodischerPolarisation. ZeitschriftfürElektrochemie und Ange- wandtePhysikalischeChemie. 1927;33:389.
-
30.
Maghraoui AE, Zerouale A, Ijjaali M, Sajieddine M. Synthesis and Characterization of Ferrate(VI) Alkali Metal by Electrochemical Method. Adv Materi Phys Chem. 2013;3(1):83-7. https://doi.org/10.4236/ampc.2013.31013.
-
31.
Bouzek K, Rousar I. Current efficiency during anodic dissolution of iron to ferrate(vi) in concentrated alkali hydroxide solutions. Journal of Applied Electrochemistry. 1993;23(12):1317-22. https://doi.org/10.1007/bf00234818.
-
32.
Bouzek K, Rousar I, Taylor MA. Influence of anode material on current yield during ferrate(vi) production by anodic iron dissolution Part I: Current efficiency during anodic dissolution of white cast iron to ferrate(vi) in concentrated alkali hydroxide solutions. J Appl Electrochem. 1996;26(9):925-32. https://doi.org/10.1007/bf00242044.
-
33.
Bouzek K, Roušar I, Taylor MA. Influence of anode material on current yield during ferrate(vi) production by anodic iron dissolution Part II: Current efficiency during anodic dissolution of white cast iron to ferrate(vi) in concentrated alkali hydroxide solutions. J Appl Electrochem. 1996;26(9):925-31. https://doi.org/10.1007/bf00242044.
-
34.
He W, Wang J, Shao H, Zhang J, Cao C. Novel KOH electrolyte for one-step electrochemical synthesis of high purity solid K2FeO4: Comparison with NaOH. Electrochem. 2005;7(6):607-11. https://doi.org/10.1016/j.elecom.2005.04.011.
-
35.
Zhihua XU, Jianming W, Haibo S, Jianqing Z. Physical Properties and Electrochemical Performance of Solid K2FeO4 Samples Prepared by Ex-situ and in-situ Electrochemical Methods. Chin J Chem Eng. 2007;15(1):39-43. https://doi.org/10.1016/s1004-9541(07)60031-7.
-
36.
Wang HL, Liu SQ, Zhang XY. Preparation and application of sustained release microcapsules of potassium ferrate(VI) for dinitro butyl phenol (DNBP) wastewater treatment. J Hazard Mater. 2009;169(1-3):448-53. [PubMed ID: 19398157]. https://doi.org/10.1016/j.jhazmat.2009.03.130.
-
37.
Chengchun J, Chen L, Shichao W. Ferrates: Synthesis, Properties, and Applications in Water and Wastewater Treatment, ACS SymposiumSeries. Oxford University Press. 2008;985:84-101.
-
38.
Paing J, Picot B, Sambuco JP, Rambaud A. Sludge accumulation and methanogenic activity in an anaerobic lagoon. Water Sci Technol. 2000;42(10-11):247-55.
-
39.
Parande AK, Palanisamy N, Ramsamy PL, Ethirajan S, Rao CRK. Deterioration of reinforced concrete in sewer environments. Proc Inst Civ Eng-Municipal Eng. 2006;159(1):11-20. https://doi.org/10.1680/muen.2006.159.1.11.
-
40.
Hvitved-Jacobsen T, Vollertsen J, Tanaka N. An integrated aerobic/anaerobic approach for prediction of sulfide formation in sewers. Water Sci Technol. 2000;41(6):107-15.
-
41.
Yang W, Vollertsen J, Hvitved-Jacobsen T. Anoxic sulfide oxidation in wastewater of sewer networks. Water Sci Technol. 2005;52(3):191-9. [PubMed ID: 16206859].
-
42.
Gadekar S, Nemati M, Hill GA. Batch and continuous biooxidation of sulphide by Thiomicrospira sp. CVO: reaction kinetics and stoichiometry. Water Res. 2006;40(12):2436-46. [PubMed ID: 16730776]. https://doi.org/10.1016/j.watres.2006.04.007.
-
43.
Fu X, Shen W. Physical-Chemistry. 1990;4.
-
44.
Yongsiri C, Vollertsen J, Hvitved-Jacobsen T. Influence of Wastewater Constituents on Hydrogen Sulfide Emission in Sewer Networks. J Environ Eng. 2005;131(12):1676-83. https://doi.org/10.1061/(asce)0733-9372(2005)131:12(1676).
-
45.
Yongsiri C, Vollertsen J, Hvitved-Jacobsen T. Hydrogen sulfide emission in sewer networks: a two-phase modeling approach to the sulfur cycle. Water Sci Technol. 2004;50(4):161-8. [PubMed ID: 15484757].
-
46.
Pomeroy R, Bowlus FD. Progress report on sulfide control research. Sewage Work J. 1946;18(4):597-640. [PubMed ID: 20999451].
-
47.
Jenkins SH. Advances in Water Pollution Research: Proceedings of the Sixth International Conference Held in Jerusalem. Proceedings of the Sixth International Conference, Jerusalem: Elsevier; 2013. p. 281-8.
-
48.
Matos J, Aires C. Mathematical modelling of sulphides and hydrogen sulphide gas build-up in the costa do estoril sewerage system. Water Science and Technology. 1995;31(7):255-61. https://doi.org/10.1016/0273-1223(95)00342-k.
-
49.
Islander RL, Devinny JS, Mansfeld F, Postyn A, Hong S. Microbial ecology of crown corrosion in sewers. J Environ Eng. 1991;117:751-70. https://doi.org/10.1061/(asce)0733-9372(1991)117:6(751).
-
50.
Redner J. EVALUATING COATINGS FOR CONCRETE IN WASTE WATER FACILITIES: AN UPDATE. J Prot Coatings Linings. 1994;11(12):50-61.
-
51.
US Environmental Protection Agency. Hydrogen sulphide corrosion in wastewater collection and treatment system. Technical Report; 1991.
-
52.
Davis JL, Nica D, Shields K, Roberts DJ. Analysis of concrete from corroded sewer pipe. Int Biodeterior Biodegradation. 1998;42(1):75-84. https://doi.org/10.1016/s0964-8305(98)00049-3.
-
53.
Vincke E. Biogenic sulfuric acid corrosion of concrete: microbial interaction, simulation and prevention [Dissertation]. Ghent, Belgium: Faculty of Bio-engineering Science, University Ghent; 2002.
-
54.
Monteny J, De Belie N, Vincke E, Verstraete W, Taerwe L. Chemical and microbiological tests to simulate sulfuric acid corrosion of polymer-modified concrete. Cement Concr Res. 2001;31(9):1359-65. https://doi.org/10.1016/s0008-8846(01)00565-8.
-
55.
Yu T, Bishop PL, Galal A, Mark HB. Fabrication and evaluation of a sulfide microelectrode for biofilm studies. 1998;690:231-47. https://doi.org/10.1021/bk-1998-0690.ch019.
-
56.
Hvitved-Jacobsen T, Vollertsen J, Matos JS. The sewer as a bioreactor--a dry weather approach. Water Sci Technol. 2002;45(3):11-24. [PubMed ID: 11902461].
-
57.
Ochi T. Controlling sulfide generation in force mains by air injection. Water Sci Technol. 1998;37(1):87-95. https://doi.org/10.1016/s0273-1223(98)00003-1.