Abstract
Background:
Textile dyes are the largest organic compounds associated with some problems for the environment. Advanced oxidation process is the most effective method for dye removal from wastewater.Objectives:
The aim of this study was to investigate the efficiency of the photo Fenton process for decolorization of direct red 81 (DR 81) dye, and to determine the optimal process conditions.Methods:
This is a cross-sectional study conducted on a laboratory scale. The effective parameters in the photo Fenton process include pH, ferrous sulfate heptahydrate concentration, hydrogen peroxide concentration, initial dye concentration and reaction time. These parameters were studied, and finally analyzed using the Excel software.Results:
According to the obtained results, maximum removal efficiency was 98.1% at pH = 3, concentration of Fe (II) was 10 mg/L, hydrogen peroxide concentration was 50 mg/L, initial concentration of dye was 100 mg/L, and reaction time was 120 minutes. Results showed that increasing the concentration of Fe (II), H2O2 and the initial concentration of dye to a certain extent resulted in increasing removal efficiency, then by increasing the concentrations of these parameters, removal efficiency decreased. Results also indicated that increasing a reaction time resulted in increasing removal efficiency.Conclusions:
The photo Fenton method with optimized parameters can be used to conduct high efficiency dye removal in a short period of time and this process is based on hydroxyl radical formation.Keywords
Industrial Wastewater Direct Red Dye 81 Photo Fenton Process Removal Efficiency
1. Background
Textile industrial wastewater contains various pollutants such as organic matters, dyes and nutrients. These pollutants are produced in different steps such as washing, sizing, belching, dyeing, printing, and other operations. The most important problem in relation to textile industrial wastewater treatment is residual dye removal from none-permanent dyes. During the dyeing processes, 5% - 20% of dye enters wastewater due to lack of stability (1-4). On the other hand, these dyes may cause adverse effects such as aesthetic, carcinogenic and toxicity and consequently can be harmful to human beings, animals and plants (5-7).
Nowadays, about 26,000 types of dyes are produced worldwide (8). The major environmental concern for industrial wastewater is related to very intense dye content as well as a high chemical oxygen demand (COD) rate (9). Therefore, continuous and constant discharging of wastewaters into water resources can cause eutrophication and aquatic life disturbance (10). Azo-dyes have different forms such as direct, acid, base, reactive, disperse, metal-complexed, mordant and sulfur dyes (11). Azo dyes, which contain one or more azo bonds (N = N), are among the most widely used synthetic dyes and usually become major pollutants in textile wastewaters (12).
Common methods of physical and chemical treatment such as chemical precipitation, coagulation and adsorption by activated carbon cannot destroy this substances and only can transfer this compound from one phase to another (11, 13, 14). Also, common biological systems cannot treat wastewaters because most of the resistant pollutants in textile industrial wastewater are toxic for living organism and have growth inhibitory properties (15, 16). In recent years, advanced oxidation processes have developed significantly. Generally, advanced oxidation processes include all processes in which active hydroxyl radicals (OH°) are produced using different methods. Hydroxyl radicals that have very high oxidation power can remove most of the pollutants, especially organic pollutants thoroughly (17). The photo Fenton process includes activated hydrogen peroxide with iron salt and removes pollutant and transfers them to CO2 and H2O and other harmless substances by producing hydroxyl radicals (18). The photo-Fenton process was conducted using H2O2, Fe (II), and UV radiation (580 nm) (19). In this process, OH° was produced in the presence of ferrous Fe (II) and peroxide hydrogen as reductive and oxidative reagents, respectively. An increase in the degradation rate of the pollutant can be attributed to the oxidation of Fe (III) to Fe (II) by UV radiation, producing OH° according to the following mechanism (Equation 1) (20):

High efficiency rates of these methods are considered by scientists and researchers of water and industrial wastewaters treatment. Other studies show that the photo Fenton process has a higher efficiency when compared to the UV/H2O2 process or photocatalytic UV/H2O2 process (17, 21). Short reaction time, low chemical consumption, and removal capability of resistant pollutants such as polyaromatic organic compounds are the other advantages of this process (15, 22).
In recent years, the photo Fenton process has been used as an environmental advocating technology and cleaning process to treat different kinds of wastewater (23, 24). The study of Perez et al. indicated that combination of Fenton and photo Fenton reactions has a high efficiency in textile industrials wastewater treatment (25). The study of Zheng et al. in investigating oxidation acidic dye Eosin Y by the photo Fenton process showed that at pH = 3.5 and reaction time of 90 minutes, 94.1% of dye was removed and the degradation rate was dependent on dye concentration (26). The study of Huang et al. on comparative survey of oxidation of dye-reactive black B by different advanced oxidation processes including Fenton, electro-Fenton and photo-Fenton indicated that Fenton alone made 70% of mineralization, whereas photo Fenton made 93% mineralization with rapid and more complete degradation of dye solution (27). The study of Mahavi et al. in investigating removal of reactive red 120 and direct red 81 (DR 81) dyes from aqueous solutions by pumice showed that pumice can be used for sorption of both RR120 and DR81 dyes from aqueous solutions (6). The study of Ali et al. in investigating removal of DR 81 dye from aqueous solution by native and citric acid modified bamboo sawdust - (a kinetic study) and equilibrium isotherm analyses showed that the developed adsorption system is inexpensive, ecofriendly and efficient for the removal of dye from any water due to its small adsorption time, good capacity of adsorption of adsorbent and working at 7 pH; a natural pH of most of natural water resources (28). Also, the study of Heravi et al. investigated equilibrium and kinetic studies of DR 81 biosorption onto modified silk maze as an economical biosorbent indicated that the modified silk maze had the maximum monolayer adsorption capacity, 5.5 mg/g (29).
However, no studies until today have been conducted on removing DR 81 dye from aqueous solutions using the photo- Fenton process. The Fenton and photo-Fenton processes have significant importance because of their high speed in removing contaminant materials and can be used for the treatment of wastewater, especially textile and dyeing wastewater (30). The study of advanced oxidation processes on removing diverse and new chemical structures requires comprehensive information about the industrial application of this process.
2. Objectives
The aim of this study was to investigate the removal efficiency of DR 81 dye using the Photo- Fenton method and also determine the optimal process conditions based on removal efficiency and the impact of various factors such as pH, concentration of ferrous sulfate heptahydrate, hydrogen peroxide concentration, initial dye concentration, and reaction time.
3. Methods
3.1. Materials
Direct red 81 dye with chemical formulation of C29H19N5Na2O8S2 molecular weight of 675.60 g/mol, and chemical materials including hydrogen peroxide (30%), ferrous sulfate heptahydrate (FeSO4.7H2O), sulfuric acid (98%), and sodium hydroxide with a laboratory purity grade were purchased from the Merck Company in Germany.
3.2. Procedures
This was a cross-sectional study performed in a laboratory scale to investigate the impact of the photo-Fenton process by determining the effects of other variables on DR 81 dye removal. The experiments were designed on the basis of one factor at the time to obtain optimum parameters. The number of samples were 25 with pH in 4 levels (3, 5, 7, 11), Fe (II) in 6 levels (0, 10, 30, 50, 100, 150 mg/L), hydrogen peroxide in 5 levels (0, 20, 50, 100, 150 mg/L), dye concentration in 4 levels (50, 100, 150, 200 mg/L), and reaction time (15, 30, 45, 60, 90, 120 minutes). Each sample was repeated 3 times to improve the accuracy and precision of the experiment. Therefore, the total number of samples was 75, and the results were presented based on the average of total number of samples. The reactor used in the experiments was a glass reactor with the volume of 1 Lit equipped with a magnetic stirrer to uniform the solution. A UV lamp with intensity of 8 W/m2 was used in the experiments Figure 1. A dye removal rate was measured using the spectrophotometer DR5000 model at the wavelength of 520. HCl and NaOH used for pH adjustment, and pH meter hatch were used for pH measurement. Dye removal efficiency was calculated by the following formula:
Equation 2.

C0 = Primary absorption percentage (mg /L).
Ce = Final absorption percentage (mg /L).
Finally, the data were analyzed using the Excel software to determine the effects of different variables on the efficiency of the decolorization process.
Photochemical Reactor
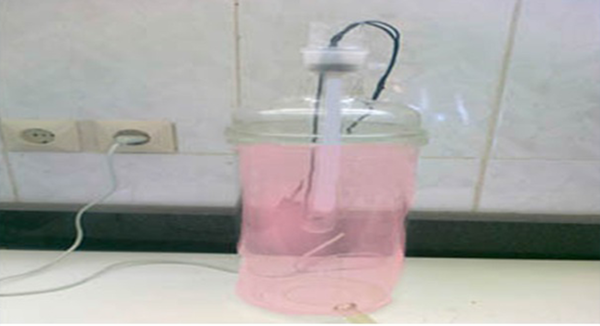
4. Results
4.1. The Effect of pH on the Removal Efficiency of Direct Red 81 Dye Using the Photo-Fenton Process
The experiments were performed at 4 levels of pH (3, 5, 7, 11) in order to determine the impact of pH on the DR 81 dye removal rate. As shown in the Figure 1, the maximum removal efficiency (31.85%) was at pH = 3. The removal efficiency decreased by increasing the pH to 5 after which pH was set on neutral value. After that, increasing pH and the alkali environment (according to Figure 2) resulted in decreasing the removal efficiency rapidly down to 1.78%
The Impact of pH on the Direct Red Dye 81 Removal Efficiency, at the Concentration of Fe (II) = 100 mg/L, H2O2 = 50 mg/L, Initial Dye Concentration of 100 mg/L, and Reaction Time of 30 minutes
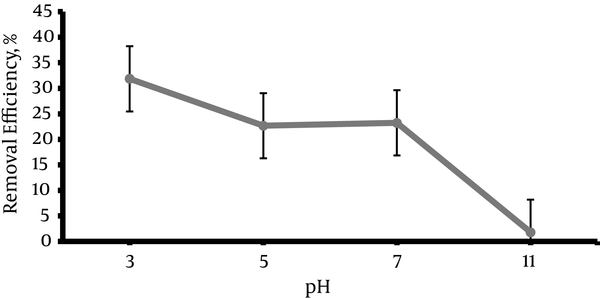
4.2. The Effect of Fe (II) Concentration on the Removal Efficiency of Direct Red 81 Dye Using the Photo- Fenton Process
The concentration of Fe (II) was investigated at different levels (0, 10, 30, 50,100, 150 mg/L). According to Figure 3, maximum removal efficiency occurred at the concentrations of 10 mg/L and 30 mg/L. According to the results, removal efficiency was increased by increasing the concentration up to 30 mg/L. Then the efficiency was reduced and in concentrations of 100 to 150 mg/L remained at a fixed value.
The Impact of Fe (II) Concentration on the Direct Red Dye 81 removal Efficiency at pH = 3 , H2O2 Concentration of 50 mg/L, Initial Dye Concentration of 100 mg/L and Reaction Time of 30 Minutes
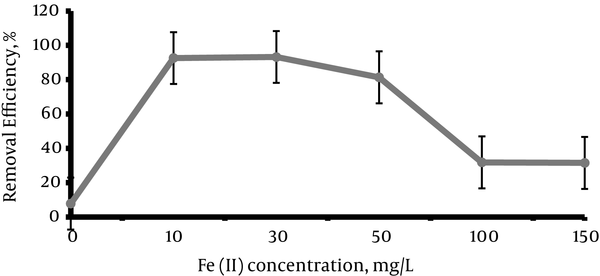
4.3. The Effect of H2O2 Concentration on the Removal Efficiency of Direct Red 81 Dye Using the Photo-Fenton Process
The concentrations of H2O2 in the experiment were 0, 20, 50, 100, 150 mg/L. According to the Figure 4, dye removal efficiency increased by increasing the concentration of H2O2 from 0 to 50 mg/L and reached to 92.59% and then the efficiency decreased and reached to 70.89% at the concentration of 150 mg/L.
The Impact of H2O2 Concentration on the Direct Red Dye 81 Removal Efficiency in pH = 3, Fe (II) Concentration of 10 mg/L, Initial Dye Concentration of 100 mg/L and Reaction Time of 30 Minutes
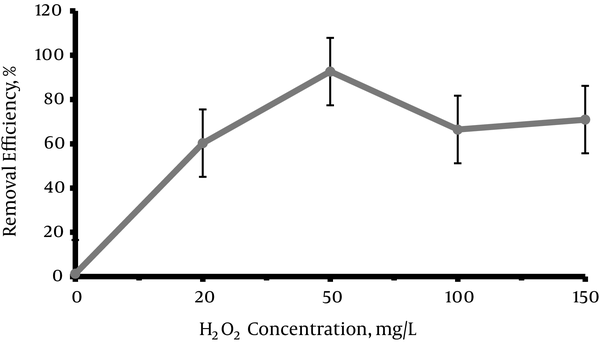
4.4. The Effect of Initial Dye Concentration on the Removal Efficiency of Direct Red 81 Dye Using the Photo Fenton Process
Four levels of dye concentrations (50, 100, 150, 200 mg/L) were experimented in this step. According to Figure 5, maximum removal efficiency was at the concentration of 100 mg/L and reached 92.59%. Then removal efficiency was reduced by increasing the concentration and reached to 37.87% at concentration of 200 mg/L.
The Impact of Initial Dye Concentration on the Direct Red Dye 81 Removal Efficiency in pH = 3, Fe (II) Concentration of 10 mg/L, H2O2 Concentration of 50 mg/L and Reaction Time of 30 Minutes
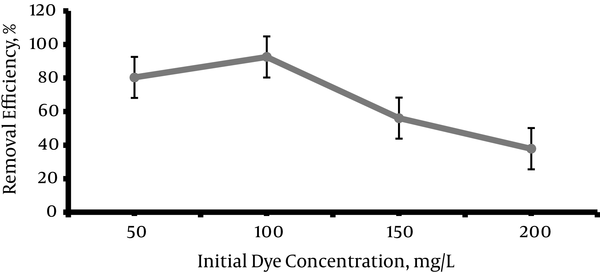
4.5. The Effect of Reaction Time on the Removal Efficiency of Direct Red 81 Dye Using the Photo Fenton Process
The experiments were performed with different reaction times of 0, 15, 30, 45, 60, 90, and 120 min. As shown in Figure 6, removal efficiency increased by increasing reaction time, then the decolorization rate increased at the beginning and declined in the final period.
The Impact of Reaction Time on the Direct Red 81 Dye Removal Efficiency in pH = 3, Fe(II) Concentration of 10 mg/L, H2O2 Concentration of 50 mg/L and Initial Dye Concentration of 100 mg/L
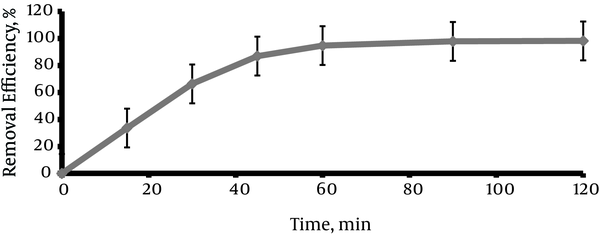
5. Discussion
According to previous studies on advanced oxidation processes, photochemical and photocatalytic, pH and contact time are considered as effective parameters on these processes (23, 31).
5.1. The Effect of pH on the Removal Efficiency of Direct Red 81 Dye Using the Photo Fenton Process
The value of pH is one of the most important environmental factors that influences the chemical process (31-33). According to Figure 2, maximum removal efficiency was in pH = 3. The removal efficiency was reduced rapidly by increasing pH in an alkali environment because of pH is a effective factor on the hydroxyl radical formation and Fe (II) concentration. Fast decreasing of efficiency in alkaline pH can be explained by the formation and precipitation of Fe (OH)3 that inhibit the development of the photo-Fenton reaction (25). The study of Zheng et al. (26), Peternel et al. (34), and Kang et al. (35) also showed that the maximum removal efficiency in the photo Fenton process occurred in the acidic pH about 3, because the maximum hydroxyl radical formation occurs in this pH and it is also more stable in this condition, This result confirms the results of the present study. At a pH lower than 3, the generated ferric hydroxide reacted with H2O2, which resulted in a decrease in the OH°, eventually decreasing the removal efficiency (Equation 3) (36).

5.2. The Effect of Fe (II) Concentration on the Removal Efficiency of Direct Red 81 Dye Using the Photo Fenton Process
According to Figure 3, the removal efficiency increased by increasing the concentration of Fe (II) to 10 mg/L, and reached to 92.6%. After that, the removal efficiency was almost constant up to Fe (II) = 30 mg/L. Then, the removal efficiency was reduced by increasing the concentration of Fe (II). These results agreed with the study results of Zheng et al., (26) and Elmorsi et al. (37). The Fe (II) additive effect is due to the catalytic properties of Fe (II) and more production of hydroxyl radical by increasing the concentration of Fe (II) (27, 38). However, the addition of extra Fe (II) concentrations more than 10 mg/L resulted in a reduction in the process efficiency and acted as a scavenger for the OH° (39).
5.3. The Effect of H2O2 Concentration on the Removal Efficiency of Direct Red 81dye Using the Photo Fenton Process
According to the Figure 4, removal efficiency increased and reached 92.59% by increasing the concentration of H2O2 to 50 mg/L. Then removal efficiency was reduced by increasing the concentration, and reached 70.89% in the concentration of 150 mg/L. In the numbers of studies, the concentration of H2O2 has been a very important factor for the oxidation reaction of organic compounds and with increasing the concentration of H2O2 to the environment optical dispersion of organic material increased, however up to a certain point. The reason is that the H2O2 concentration more than the optimal concentration may enhance the production of OH-, which consumes the OH° and decreases the removal rate (19, 37, 38). Rahmani et al. (40) revealed that BV16 and RR120 removal rates were accelerated by increasing the concentration of H2O2 until to the optimum level, after which point the dye removal efficiency decreased. Many studies including the study of Daneshvar et al. (3), Zheng et al. (26), and Li et al. (41) also confirmed the results of the present study.
5.4. The Effect of Initial Dye Concentration on the Removal Efficiency of Direct Red 81 Dye Using the Photo Fenton Process
According to the Figure 5, removal efficiency increased (92.6%) by increasing the initial dye concentration up to 100 mg/L, but, after that, decolorizing efficiency decreased with increasing an initial dye concentration and reached 37.9% in the concentration of 200 mg/L. Many studies have shown that decolorizing percent could be reduced by increasing the initial dye concentration. The reason for this reduction is decreasing in penetrating of photons in dye solution due to increase in UV absorption by dye solution. These results corresponded with the results of this study (26, 41-43).
5.5. The Effect of Reaction Time on the Removal Efficiency of Direct Red 81 Dye Using the Photo Fenton Process
The reaction time is one of the most effective factors in the advanced oxidation processes. Figure 6 shows the effect of reaction time in determining optimal conditions of experiment. According to the illustration, dye removal rate is very low (33.65%) in the first 15 minutes, but increases significantly as time goes by and results in further formation of hydroxyl radicals, and maximum efficiency (98.2%) is observed in 120 minutes that corresponds with the other studies (27, 44, 45). The decolorization rate in this study, as in many other studies, increases by increasing the reaction time, and maximum removal efficiency occurs in the initial times, however, the efficiency does not increase significantly in final phases (35, 38, 44). According to time and economical aspects, removal efficiency does not increase significantly in the final phases, and only the initial 45 minutes is the optimum time.
5.6. Conclusions
The results show that removal efficiency by the photo Fenton process increased by increasing the Fe (II), H2O2, and initial dye concentration to a certain limit, due to further formation of hydroxyl radical. The removal efficiency was also increased by increasing the reaction time. In this study, pH = 3, Fe (II) concentration of 10 mg/L, H2O2 concentration of 50 mg/L, dye concentration of 100 mg/L, and reaction time of 45 minutes were considered as the optimal parameters. Moreover, the dye removal efficiency from aqueous solutions was more than 85% at optimal conditions. Therefore, the study results indicated that the photo Fenton process could be used as an effective method to remove DR 81 dye from industrial wastewater. Due to the financial limitations of the study, we only determined the removal efficiency of DR 81 dye using a spectrophotometer (DR 5000). Therefore, it is highly recommended that future studies use the gas chromatography-mass spectrometry (GC-MS) method to determine the intermediate compound, which may be produced from the photo-Fenton reaction.
Acknowledgements
References
-
1.
Kafi Z, Ayati B. Study of dye removal from aqueous solution using sawdust and clay. Modares Civil Engineering. 2011;11(3):67-76.
-
2.
Massoudinejad M, Yazdanbakhsh A, Sharifi M. Investigation of electrocoagulation process efficiency for color removal from polyacrylic textile industrial wastewater. Water Wastewater. 2013;4(88).
-
3.
Daneshvar N, Khataee AR. Removal of azo dye C.I. acid red 14 from contaminated water using Fenton, UV/H(2)O(2), UV/H(2)O(2)/Fe(II), UV/H(2)O(2)/Fe(III) and UV/H(2)O(2)/Fe(III)/oxalate processes: a comparative study. J Environ Sci Health A Tox Hazard Subst Environ Eng. 2006;41(3):315-28. [PubMed ID: 16484066]. https://doi.org/10.1080/10934520500423196.
-
4.
Zaied M, Bellakhal N. Electrocoagulation treatment of black liquor from paper industry. J Hazard Mater. 2009;163(2-3):995-1000. [PubMed ID: 18814966]. https://doi.org/10.1016/j.jhazmat.2008.07.115.
-
5.
Ashrafi SD, Rezaei S, Forootanfar H, Mahvi A, Faramarzi MA. The enzymatic decolorization and detoxification of synthetic dyes by the laccase from a soil-isolated ascomycete, Paraconiothyrium variabile. Int Biodeter Biodegr. 2013;85:173-81.
-
6.
Mahavi H, Behzad H. Removal of reactive red 120 and direct red 81 dyes from aqueous solutions by pumice. Res J Chemistry Environment. 2012;16(1):62-8.
-
7.
Mahvi AH, Ghanbarian M, Nasseri S, Khairi A. Mineralization and discoloration of textile wastewater by TiO 2 nanoparticles. Desalination. 2009;239(1):309-16.
-
8.
Zohra B, Aicha K, Fatima S, Nourredine B, Zoubir D. Adsorption of Direct Red 2 on bentonite modified by cetyltrimethylammonium bromide. Chemi Eng J. 2008;136(2):295-305.
-
9.
Kurt U, Apaydin O, Gonullu MT. Reduction of COD in wastewater from an organized tannery industrial region by Electro-Fenton process. J Hazard Mater. 2007;143(1-2):33-40. [PubMed ID: 17014953]. https://doi.org/10.1016/j.jhazmat.2006.08.065.
-
10.
Bellakhal N, Dachraoui M, Oturan N, Oturan MA. Degradation of tartrazine in water by electro-Fenton process. SCT. 2006;8(2):223.
-
11.
Ay F, Catalkaya EC, Kargi F. A statistical experiment design approach for advanced oxidation of Direct Red azo-dye by photo-Fenton treatment. J Hazard Mater. 2009;162(1):230-6. [PubMed ID: 18555599]. https://doi.org/10.1016/j.jhazmat.2008.05.027.
-
12.
Mahmoodi NM, Arami M, Limaee N, Tabrizi N. Decolorization and aromatic ring degradation kinetics of Direct Red 80 by UV oxidation in the presence of hydrogen peroxide utilizing TiO 2 as a photocatalyst. Chem Eng J. 2005;112(1):191-6.
-
13.
Daneshvar N, Salari D, Khataee A. Photocatalytic degradation of azo dye acid red 14 in water: investigation of the effect of operational parameters. J Photochem Photobiol. 2003;157(1):111-6.
-
14.
Daneshvar N, Salari D, Khataee A. Photocatalytic degradation of azo dye acid red 14 in water on ZnO as an alternative catalyst to TiO 2. 2004;162(2):317-22.
-
15.
Ventura A, Jacquet G, Bermond A, Camel V. Electrochemical generation of the Fenton's reagent: application to atrazine degradation. Water Res. 2002;36(14):3517-22. [PubMed ID: 12230197].
-
16.
Oturan MA, Sires I, Oturan N, Perocheau S, Laborde J, Trevin S. Sonoelectro-Fenton process: a novel hybrid technique for the destruction of organic pollutants in water. J Electroanal Chem. 2008;624(1):329-32.
-
17.
Daneshvar N, Salari D, Niaei A, Khataee AR. Photocatalytic degradation of the herbicide erioglaucine in the presence of nanosized titanium dioxide: comparison and modeling of reaction kinetics. J Environ Sci Health B. 2006;41(8):1273-90. [PubMed ID: 17090492]. https://doi.org/10.1080/03601230600962302.
-
18.
Kusic H, Koprivanac N, Bozic AL, Selanec I. Photo-assisted Fenton type processes for the degradation of phenol: a kinetic study. J Hazard Mater. 2006;136(3):632-44. [PubMed ID: 16466856]. https://doi.org/10.1016/j.jhazmat.2005.12.046.
-
19.
Biglari H, Kord MF, Joneydi JA, Bazrafshan E. Removal of Humic acid from environmental aqueous by Fenton Oxidation process. NKHMJ. 2013;5(1).
-
20.
Vaishnave P, Kumar A, Ameta R, Punjabi P, Ameta SC. Photo oxidative degradation of azure-B by sono-photo-Fenton and photo-Fenton reagents. Arabian J Chem. 2014;7(6):981-5.
-
21.
Chen F, Xie Y, He J, Zhao J. Photo-Fenton degradation of dye in methanolic solution under both UV and visible irradiation. J Photochem Photobiol. 2001;138(2):139-46.
-
22.
Kos L, Perkowski J. Decolouration of real textile wastewater with advanced oxidation processes. Fibres and textiles in Eastern Europe. 2003;11(4):81-5.
-
23.
Panizza M, Cerisola G. Removal of organic pollutants from industrial wastewater by electrogenerated Fenton's reagent. Water Res. 2001;35(16):3987-92. [PubMed ID: 12230183].
-
24.
Anotai J, Lu MC, Chewpreecha P. Kinetics of aniline degradation by Fenton and electro-Fenton processes. Water Res. 2006;40(9):1841-7. [PubMed ID: 16624370]. https://doi.org/10.1016/j.watres.2006.02.033.
-
25.
Perez M, Torrades F, Domenech X, Peral J. Fenton and photo-Fenton oxidation of textile effluents. Water Res. 2002;36(11):2703-10. [PubMed ID: 12146857].
-
26.
Zheng H, Pan Y, Xiang X. Oxidation of acidic dye Eosin Y by the solar photo-Fenton processes. J Hazard Mater. 2007;141(3):457-64. [PubMed ID: 17250960]. https://doi.org/10.1016/j.jhazmat.2006.12.018.
-
27.
Huang YH, Huang YF, Chang PS, Chen CY. Comparative study of oxidation of dye-Reactive Black B by different advanced oxidation processes: Fenton, electro-Fenton and photo-Fenton. J Hazard Mater. 2008;154(1-3):655-62. [PubMed ID: 18063472]. https://doi.org/10.1016/j.jhazmat.2007.10.077.
-
28.
Ali I, Dahiya S, Tabrez K. Removal of direct red 81 dye from aqueous solution by native and citric acid modified bamboo sawdust-Kinetic study and equilibrium isotherm analyses. Gazi University J Sci. 2012;25(1):59-87.
-
29.
Heravi M, Ardalan P, Vafaie A. Equilibrium and kinetic studies of direct red 81 biosorption onto modified silk maze as an economical biosorbent. J Chem Health Risks. 2014;4(1).
-
30.
Khataee A, Daneshvar N, Rasoulifard MH, Doraji MS. Removal of organic dyes from industrial wastewaters using UV/H2O2, UV/H2O2/Fe (II), UV/H2O2/Fe (III) processes. Water Wastewater. 2007;61.
-
31.
Dehghani M, Haidari E, Shahsavani S, Shamsedini N. Removal of nitrate in the aqueous phase using granular ferric hydroxide. Jundishapur J Health Sci. 2015;7(2).
-
32.
Dehghani M, Taghizadeh M, Gholami T, Ghadami M, Keshtgar L, Elhameyan Z, et al. Optimization of the Parameters Influencing the Photo-Fenton Process for the Decolorization of Reactive Red 198 (RR198). JJHS. 2015;7(2).
-
33.
Dehghani M, Shahsavani S, Shamsedini N, Javaheri M. Removal of nitrate from aqueous solution using rice chaff. Jentashapir J Health Res. 2015;6(5).
-
34.
Peternel IT, Koprivanac N, Bozic AM, Kusic HM. Comparative study of UV/TiO2, UV/ZnO and photo-Fenton processes for the organic reactive dye degradation in aqueous solution. J Hazard Mater. 2007;148(1-2):477-84. [PubMed ID: 17400374]. https://doi.org/10.1016/j.jhazmat.2007.02.072.
-
35.
Kang SF, Liao CH, Po ST. Decolorization of textile wastewater by photo-fenton oxidation technology. Chemosphere. 2000;41(8):1287-94. [PubMed ID: 10901260].
-
36.
Bahmani P, Maleki A, Ghahremani A, Kohzadi S. Efficiency of Fenton oxidation process in removal of Remazol Black-B from aqueous medium. 2013;4(1):57-67.
-
37.
Elmorsi TM, Riyad YM, Mohamed ZH, Abd El Bary HM. Decolorization of Mordant red 73 azo dye in water using H2O2/UV and photo-Fenton treatment. J Hazard Mater. 2010;174(1-3):352-8. [PubMed ID: 19825508]. https://doi.org/10.1016/j.jhazmat.2009.09.057.
-
38.
Mehrasbi M, Safa S, Mahvi A, Assadi A, Mohammadi H. Application of photo-Fenton process for COD removal from wastewater produced from surfactant-washed oil-contaminated (TPH) soils. IJHE. 2012;5(3):295-306.
-
39.
Standards methods for the examination of water and wastewater. Washington; 2005.
-
40.
Rahmani Z, Kermani M, Gholami M, Jafari AJ, Mahmoodi NM. Effectiveness of photochemical and sonochemical processes in degradation of Basic Violet 16 (BV16) dye from aqueous solutions. Iranian J Environ Health Sci Eng. 2012;9(1):14. [PubMed ID: 23369268]. https://doi.org/10.1186/1735-2746-9-14.
-
41.
Li Y, Lu Y, Zhu X. Photo-Fenton discoloration of the azo dye X-3B over pillared bentonites containing iron. J Hazard Mater. 2006;132(2-3):196-201. [PubMed ID: 16621280]. https://doi.org/10.1016/j.jhazmat.2005.07.090.
-
42.
Feng J, Hu X, Yue PL, Zhu HY, Lu GQ. Discoloration and mineralization of Reactive Red HE-3B by heterogeneous photo-Fenton reaction. Water Res. 2003;37(15):3776-84. [PubMed ID: 12867346]. https://doi.org/10.1016/S0043-1354(03)00268-9.
-
43.
Chakrabarti S, Dutta BK. Photocatalytic degradation of model textile dyes in wastewater using ZnO as semiconductor catalyst. J Hazard Mater. 2004;112(3):269-78. [PubMed ID: 15302448]. https://doi.org/10.1016/j.jhazmat.2004.05.013.
-
44.
Massoudinejad M, SHarifimaleksari H, Yazdanbakhsh A, Mahdipour F. Decolorization of the real textile effluent by electrocoagulation process using monopolar iron electrodes. 2014.
-
45.
Dehghani M, Shabestari R, Anushiravani A, Shamsedini N. Application of electrocoagulation process for reactive red 198 dye removal from the aqueous solution. IJHS. 2014;2(2):1-9.